1 Introduction
In recent years, design researchers have increasingly attempted to establish links between the design domain and the broader area of cognitive science. This aims to foster the development of common models and theories across the two domains and to remove some of the inconsistencies within design research by relating them to more established notions and terms in cognitive science (Hay et al. Reference Hay, Duffy, McTeague, Pidgeon, Vuletic and Grealy2017). Methods and advances in cognitive science can then flow into design science. This can also provide design practitioners access to a new range of methods and tools such as creativity tools (Howard, Culley & Dekoninck Reference Howard, Culley and Dekoninck2008).
One well-established model of human thinking from cognitive psychology that is supported by numerous empirical studies is the dual-system theory or, sometimes called, dual-process theory (Wason & Evans Reference Wason and Evans1975; Sloman Reference Sloman1996; Stanovich & West Reference Stanovich and West2000; Kahneman & Frederick Reference Kahneman, Frederick, Gilovich, Griffin and Kahneman2002; Evans & Stanovich Reference Evans and Stanovich2013). A number of accounts of this theory exist that differ in a few details (Evans & Stanovich Reference Evans and Stanovich2013). Daniel Kahneman, in his book Thinking, Fast and Slow (Kahneman Reference Kahneman2011), made dual-system theory accessible to people outside cognitive psychology. What is common to all accounts of dual-system theory is that human cognition is described to be governed by two systems: system 1 for fast, intuitive and effortless thinking, and system 2 for slower, analytic thinking that requires greater cognitive effort. System 1 is assumed to govern much of our daily behaviour. It is only when the fast responses generated by system 1 do not produce the results expected that system 2 comes into play. Dual-system theory challenges common beliefs of ourselves as rational beings that process information and take decisions objectively and analytically.
In this paper, we examine the link between dual-system theory and a special area of human cognition: design thinking. Design thinking has a tradition as a scientific study (e.g. Simon Reference Simon1969). Recently, it has been understood as a notion covering a set of techniques for stimulating creative solutions to problems in business, education and social domains (Brown Reference Brown2009; Verganti Reference Verganti2009; Liedtka & Ogilvie Reference Liedtka and Ogilvie2011). This paper uses the concept of design thinking in the more general sense, encompassing the specific techniques applied in different domains. It thereby aims to lay the foundations for improved design tools: if dual-system theory plays a role in design, then tool support for system 1 and system 2 design thinking is likely to differ. The work presented here contributes to the development of such a design support by identifying the locations of the two modes of design thinking.
Specifically, we relate dual-system theory and design thinking based on the function–behaviour–structure (FBS) ontology (Gero Reference Gero1990; Gero & Kannengiesser Reference Gero and Kannengiesser2004, Reference Gero, Kannengiesser, Chakrabarti and Blessing2014). System 1 design thinking is shown as a new process within the FBS ontology. The FBS ontology is viewed as a suitable framework for the purposes of this study because it is widely referenced in academia (currently, more than 3,000 citations on Google Scholar for the two papers: Gero (Reference Gero1990) and Gero & Kannengiesser (Reference Gero and Kannengiesser2004)) and its meta-cognitive, descriptive perspective that focuses on individual mental activities of designing (Wynn & Clarkson Reference Wynn and Clarkson2018). The ‘micro-level’ (ibid.) focus of the FBS ontology has enabled its widespread use as a coding schema for design protocols. Studies carried out include aerospace system development projects in industry, where more than 10,000 hours of designing spread over multiple years were coded based on FBS (Bott & Mesmer Reference Bott and Mesmer2019). Using the FBS ontology as a basis for representing dual-system theory can therefore provide the foundation for empirically testing the hypothesis that systems 1 and 2 thinking occur in design.
The paper is organised as follows: Section 2 describes the foundations of dual-system theory mainly based on Kahneman’s (Reference Kahneman2011) account. Section 3 presents previous research on design thinking and its relation to fast and slow modes of reasoning. Section 4 introduces the FBS ontological model of design thinking. Section 5 represents the two systems in the FBS ontology and explains how they interact. Section 6 casts instances of fast and slow design thinking in this ontology. These cover design fixation, case-based design, pattern-language-based design and brainstorming. Section 7 discusses these examples. Section 8 concludes the paper with a summary and discussion of future work.
2 Dual-system theory
According to dual-system theory, human cognition has two distinct types of thinking: one that is fast, automatic and effortless, and one that is slow, analytic and effortful. This theory originates from the 1970s (e.g. Wason & Evans Reference Wason and Evans1975) and is now well established with a large amount of experimental evidence in cognitive psychology and neuroscience. While the two types of thinking are independent of particular areas in the brain (Evans & Stanovich Reference Evans and Stanovich2013), Kahneman (Reference Kahneman2011) refers to them as ‘system 1’ and ‘system 2’, respectively. He does this to enable his readers to conceptualise them as two different characters with distinct personalities rather than as abstract concepts, and thus to facilitate understanding. In this paper, we will also use Kahneman’s terms and descriptions. This is to build on Kahneman’s successful transfer of dual-system theory concepts outside the cognitive science domain, thus enabling their understanding by design scientists.
Most of Kahneman’s (Reference Kahneman2011) book is about system 1. This is because its influence on human reasoning is underestimated by many people who are commonly convinced about the rationality and objectiveness of their beliefs, decisions and actions. Kahneman shows that system 1 is involved in a large part of human reasoning, which becomes most evident by the many systematic errors of judgement (also called cognitive biases). One of the examples provided by Kahneman (Reference Kahneman2011, p. 44) is based on the so-called ‘bat-and-ball problem’:
‘A bat and ball cost $1.10.
The bat costs one dollar more than the ball.
How much does the ball cost?’
While the correct answer to this problem is 5 cents, most people’s intuition tells them it is 10 cents. The fast but wrong response generated by system 1 in this case was not challenged by system 2. The reason is, on the one hand, that system 1 is ‘overconfident’ (ibid.). On the other hand, system 2 is ‘lazy’ (ibid.): it directs attention for system 1 and then relies on its automatic response, rather than spending effort in checking the correctness of that response. Errors are detected only when they are obvious or when a likely risk of failure is known in advance.
Despite the various fallacies of fast thinking, system 1 also provides a tool for responding to uncertain or ambiguous situations where the use of analytical reasoning would be impossible or impractical. This is done by what Kahneman calls ‘substitution’ (ibid.): replacing complex problems by simpler ones. For example, the question ‘How happy are you with your life these days?’, which may be rather difficult to answer analytically, can be substituted by the simpler question ‘What is my mood right now?’, which can be answered quickly by system 1 (Kahneman Reference Kahneman2011, p. 98). Substitution thus provides us with imperfect yet often adequate answers to difficult questions.
The use of system 1 in these situations is not completely under our control. Kahneman illustrates this with a well-known optical illusion of the kind depicted in Figure 1. As printed on the page, the three human figures are of equal size. Yet, the figure on the left appears larger than the one on the right. This is because the cues contained in the image suggest a 3D interpretation, making system 1 automatically substitute the question posed in the caption of Figure 1 with the following: ‘How tall are the three people?’ (Kahneman Reference Kahneman2011, p. 101).
The three-people example shows that another characteristic of system 1 is that it performs many computations at once, many of which are contextual and beyond our conscious control. Kahneman (Reference Kahneman2011, p. 95) calls this the ‘mental shotgun’:
‘[…] we often compute much more than we want or need. I call this excess computation the mental shotgun. It is impossible to aim at a single point with a shotgun because it shoots pellets that scatter, and it seems almost equally difficult for System 1 not to do more than System 2 charges it to do’.

Figure 1. Are the three figures, as printed on the page, of different size? (Source: https://therealweeklyshow.wordpress.com/2014/01/15/5-more-mind-bending-optical-illusions/.)
A summary of characteristics commonly ascribed to system 1 and system 2 is provided in Table 1. It is based on a list of attributes compiled by Evans & Stanovich (Reference Evans and Stanovich2013).
Table 1. Characteristics of system 1 and system 2
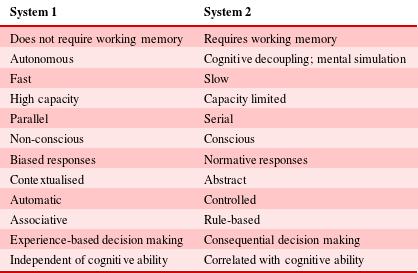
3 Does a fast mode of design thinking exist?
Designing is commonly viewed as a complex activity that is not associated with a fast, effortless mode of thinking. Most design processes in industry take place within timeframes of weeks and months and, in some cases, several years. Even the accomplishment of design exercises in university classrooms takes at least a couple of hours – which is very slow compared to most instances of fast, intuitive thinking provided by system 1. Yet, a number of theoretical and empirical studies show that a fast mode of thinking does play a role in design, at least for parts of the design process.
Fostering fast design thinking has been one of the goals of research in design methods. For example, design catalogues (Roth Reference Roth2000), selection charts (Pahl & Beitz Reference Pahl and Beitz2007) and design patterns (Alexander et al. Reference Alexander, Ishikawa, Silverstein and Jacobson1977; Gamma et al. Reference Gamma, Helm, Johnson and Vlissides1995) can be viewed as methods for increasing the efficiency with which designers come up with design concepts and solutions. They are based on collections of existing design solutions that are indexed and stored for fast reuse by designers when confronted with similar design problems. While finding and selecting suitable instances of these methods can be time-consuming especially in non-routine design situations, the ultimate goal is always to speed up the process of design synthesis based on codifications of previous design knowledge.
Recent studies examine the role of intuition or gut feeling in design. Badke-Schaub & Eris (Reference Badke-Schaub, Eris, Chakrabarti and Blessing2014) characterise design intuition as holistic, fast, multisensorial and experience-based. In addition, they see intuition as related to unconscious processes, emotions and creativity. They propose a framework of design thinking that integrates intuitive and rational decision making. Taura & Nagai (Reference Taura and Nagai2017) examine the role of intuition in creative design, distinguishing two kinds of intuition: experiential and associative intuition. Experiential intuition uses previously grounded patterns of experience and associative intuition combines sets of different experiences. The notion of grounding refers to the construction of design experiences based on the interaction of designers with their current view of the world that is formed by their previous experiences and interactions (Gero Reference Gero1999).
Research in design computing has come up with three modes of reasoning (Maher & Gero Reference Maher and Gero2002) – reflexive, reactive and reflective reasoning – in a design agent; these imply different speeds with which they are carried out.
Reflexive reasoning is a direct response of the design agent to specific sets of stimuli. Reasoning here does not entail any internal processing or decision making; it is merely a mapping of sensory input to actions performed by the design agent’s effectors. Hence, reflexive reasoning can be considered as fast. Examples include hard-wired, biological reflexes and habituated responses to recurring stimuli.
Reactive reasoning involves a limited form of interaction between the design agent’s internal representations. This interaction can be viewed as the process of selecting among several alternatives the most appropriate schema, given the stimuli presented, where each alternative is a mapping of sensory input to actions. As a result, agents assess their decisions by monitoring the effects of their actions and comparing them against a set of criteria. Reactive reasoning can still be considered rather fast but requiring slightly more time and effort than reflexive reasoning.
Reflective reasoning involves a more significant amount of interaction between a model of the external world and the design agent’s goals and concepts. It is a construction process that uses filtering, emphasising and distorting of certain aspects of the external cues, driven by changes in the agent’s expectations. The outcomes of actions devised by this mode of reasoning produce new expectations that provide new criteria for assessing these actions. Reflective reasoning is usually slow and requires high amounts of cognitive effort by the agent.
The three modes of reasoning have been used for a model of affordances (Gero & Kannengiesser Reference Gero and Kannengiesser2012; Kannengiesser & Gero Reference Kannengiesser and Gero2012) that shares similarities with some of the examples of fast and slow thinking presented in Kahneman’s (Reference Kahneman2011) book. The effect of learning on the three modes of reasoning has been shown computationally by Gero & Peng (Reference Gero and Peng2009), as experiences become more grounded in an agent’s memory, the corresponding reasoning processes over time transform from being reflective to reactive and then reflexive.
Some evidence for the existence of fast ways of thinking in design comes from empirical studies. Designers are frequently observed to ‘jump’ to concrete design concepts right from the beginning of a design task (Ullman, Dietterich & Stauffer Reference Ullman, Dietterich and Stauffer1988; Lawson Reference Lawson1994; Cross Reference Cross, Eastman, Newstetter and McCracken2001; Kannengiesser & Gero Reference Kannengiesser and Gero2017). This may lead to designers getting stuck or ignoring alternative concepts – a phenomenon commonly known as design fixation (Jansson & Smith Reference Jansson and Smith1991). It can be seen as a downside of approaches for increasing design efficiency based on previous design solutions.
The designers’ preference for swift solutions rather than lengthy analyses has also been observed in other empirical studies even though they have different research goals. For example, the low acceptance of functional modelling methods by design practitioners has partially been explained by their tendency ‘to avoid abstract and solution-neutral thinking, […] instead preferring to work in a goal/solution-oriented fashion that swiftly leads towards a concrete result’ (Eisenbart & Kleinsmann Reference Eisenbart and Kleinsmann2017). Similar observations were made by Eckert (Reference Eckert2013) and Eisenbart, Gericke & Blessing (Reference Eisenbart, Gericke and Blessing2017).
4 A framework for design thinking
A framework for design thinking is based on the FBS ontology (Gero Reference Gero1990; Gero & Kannengiesser Reference Gero, Kannengiesser, Chakrabarti and Blessing2014). It has been proposed as a design ontology that describes all designed things, or artefacts, irrespective of the specific discipline of designing. Its three fundamental constructs – function (F), behaviour (B) and structure (S) – are defined as follows:
Function is the teleology of the artefact (‘what the artefact is for’). It is ascribed to the artefact by establishing a connection between one’s goals and the artefact’s measurable effects.
Behaviour is defined as the artefact’s attributes that can be derived from its structure (‘what the artefact does’). Behaviour provides measurable performance criteria for comparing different artefacts.
Structure is defined as its components and their relationships (‘what the artefact consists of’).
Humans construct connections between function, behaviour and structure through experience and through the development of causal models based on interactions with the artefact. Specifically, function is ascribed to behaviour by establishing a teleological connection between the human’s goals and the observable or measurable performance of the artefact. Behaviour is causally connected to structure, i.e. it can be derived from structure using physical or other causal-type laws or heuristics. There is no direct connection between function and structure.
The FBS ontology defines the processes of designing and design thinking as transformations between function, behaviour and structure (Gero Reference Gero1990; Gero & Kannengiesser Reference Gero, Kannengiesser, Chakrabarti and Blessing2014). The most basic view of designing consists of transformations from function to behaviour and from behaviour to structure:
(1) F
$\rightarrow$ B;
(2) B
$\rightarrow$ S.
In this view, behaviour is interpreted as the performance expected to achieve the desired function. Yet, once a structure is produced, it must be checked whether the artefact’s ‘actual’ performance, based on the structure produced and the operating environment, matches the ‘expected’ behaviour. Therefore, the FBS ontology distinguishes two classes of behaviour: expected behaviour (Be) and behaviour derived from structure (Bs). This extends the set of transformations with which we can describe designing to include:
(1) F
$\rightarrow$ Be;
(2) Be
$\rightarrow$ S;
(3) S
$\rightarrow$ Bs;
(4) Be
$\leftrightarrow$ Bs (comparison of the two types of behaviour).
The observable input and output of any design activity is a set of requirements (R) that come from outside the designer and a description (D) of the artefact, respectively. The FBS ontology subsumes R in the notion of function and defines D as the external representation of a design solution:
(5) S
$\rightarrow$ D.
Based on the common observation that designing is not only a process of iterative, incremental development but frequently involves focus shifts, lateral thinking and emergent ideas, the FBS ontology defines the following additional transformations:
(6) S
$\rightarrow$ S
$^{\prime }$ ;
(7) S
$\rightarrow$ Be
$^{\prime }$ ;
(8) S
$\rightarrow$ F
$^{\prime }$ .
The eight fundamental transformations or processes in the FBS ontology are shown and labelled in Figure 2:
(1) Formulation (R
$\rightarrow$ F and F
$\rightarrow$ Be)
(2) Synthesis (Be
$\rightarrow$ S)
(3) Analysis (S
$\rightarrow$ Bs)
(4) Evaluation (Be
$\leftrightarrow$ Bs)
(5) Documentation (S
$\rightarrow$ D)
(6) Reformulation type 1 (S
$\rightarrow$ S
$^{\prime }$ )
(7) Reformulation type 2 (S
$\rightarrow$ Be
$^{\prime }$ )
(8) Reformulation type 3 (S
$\rightarrow$ F
$^{\prime }$ ).
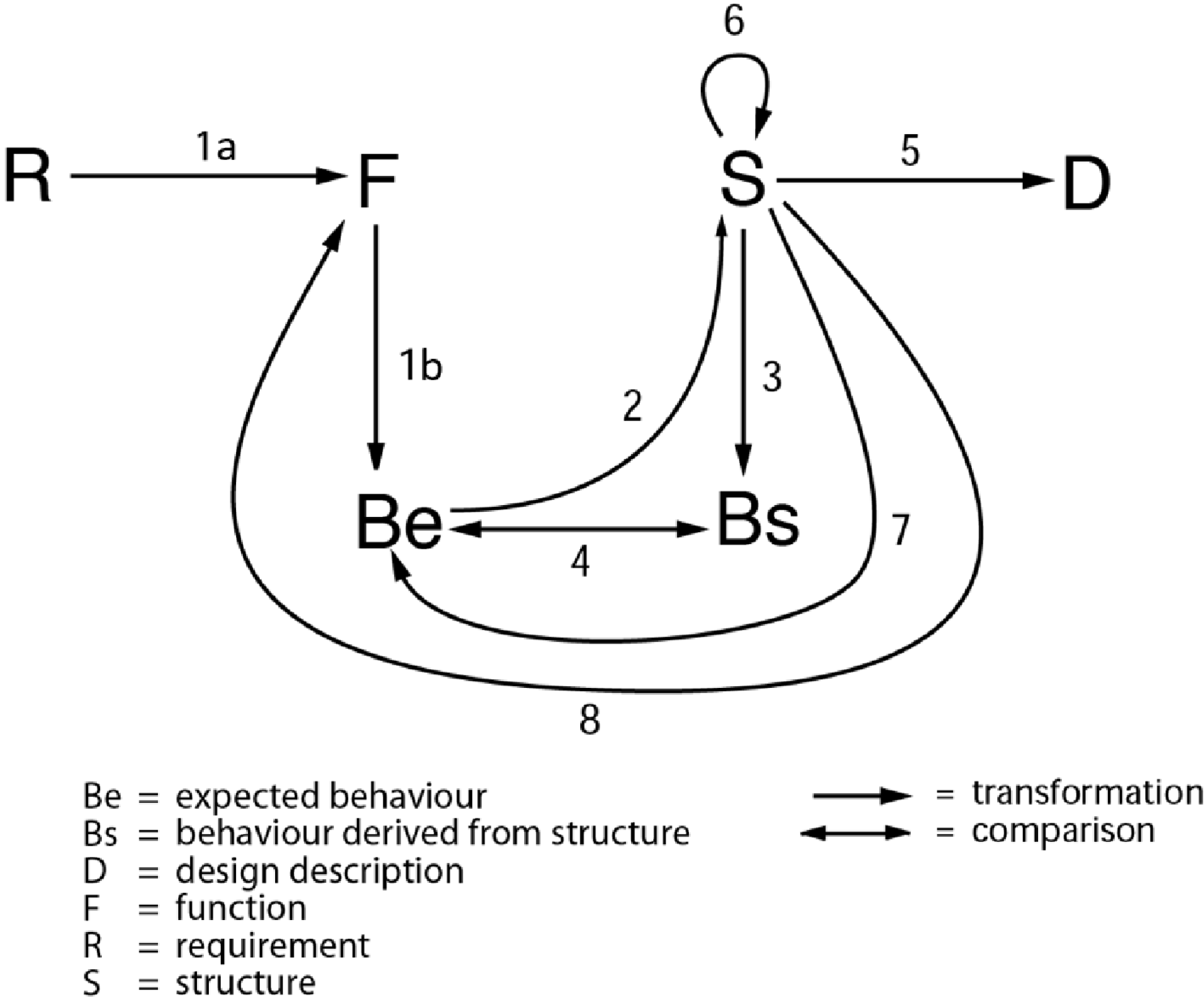
Figure 2. The FBS ontology defining eight fundamental processes in designing.
5 Locating the two systems in the FBS ontology
For locating the two systems of the dual-system theory in the FBS ontology, it is useful to begin with a simplified view of design thinking: as an input–output transformation, where the designer takes requirements as input and produces a design description as output. (The term ‘designer’ is used here to encompass a single designer or a design team.) This is shown in Figure 3.

Figure 3. An input–output view of design thinking: a designer transforming requirements (R) into a design description (D).
The details of the transformation of R into D are hidden inside the designer that is viewed as a ‘black box’ (indicated by the ‘
$+$
’ symbol in Figure 3). In Figure 4, this black box is expanded to show possible pathways from R to D, using the processes defined in the FBS ontology. The entry and exit paths of this process system are the transformations of R into F (part of formulation, process 1) and of S into D (documentation, process 5), respectively. They correspond to activities of interpretation and action that are executed by the designer.
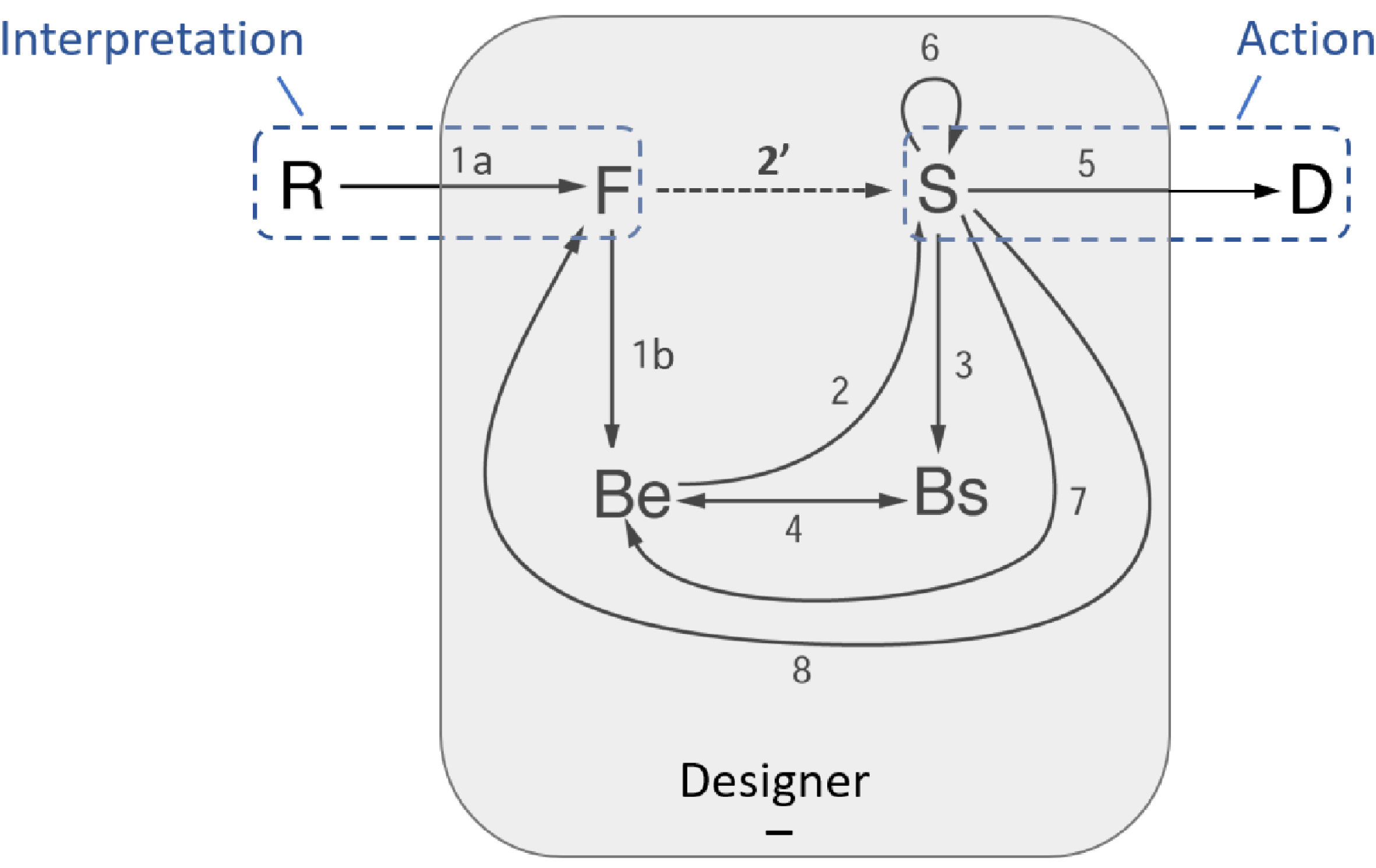
Figure 4. Expanding the transformation of R into D based on the FBS ontology.
While either of the two systems can occur in any of the processes, we focus system 1 thinking on the process that leads to the most reduction in cognitive load: F to S. Figure 4 represents a novel extension of the original FBS ontology, resulting from locating dual-system theory in it for F to S. In addition to the eight fundamental processes in the FBS ontology, a ninth process (
$2^{\prime }$
) is depicted that transforms F into S. This additional process allows distinguishing two basic pathways between the interpretation of R and the action producing D: (1) a direct pathway provided by process
$2^{\prime }$
and (2) an indirect pathway that involves at least four processes: 1b, 2, 3 and 4. Processes 3 and 4 are needed in the latter pathway based on the assumption that designing requires analysis and evaluation of the generated S prior to producing D.
Since
$2^{\prime }$
establishes a direct link between interpretation and action, it can be seen as a reflex – an immediate response to a stimulus without involving any form of reasoning. This corresponds to system 1. The reflex represented by process
$2^{\prime }$
is based on learning a connection between stimulus and response through previous experiences of the designer. Whenever a pattern in the environment is interpreted that matches a previous stimulus, the associated response is executed as an instant reflex. Examples of pattern matching in architectural design include designing using precedents (Clark & Pause Reference Clark and Pause2005).
Process
$2^{\prime }$
can be thought of as subsuming the set of processes 1b, 2, 3 and 4, as is shown in Figure 5:

where
$\unicode[STIX]{x1D703}$
is a substitution.Footnote
1

Figure 5. Process
$2^{\prime }$
(highlighted using a bold, solid arrow) subsumes processes 1b, 2, 3 and 4 (highlighted using bold, dotted arrows).
The new process is termed
$2^{\prime }$
to emphasise its principal role as a producer of synthesised S, even though it substitutes processes 1, 3 and 4 as well. It provides a shortcut for this quadruple of processes using a learned connection between F and S. This increases cognitive efficiency when performing design tasks that address similar Fs. Learning the connection between F and S involves eliminating all intermediate processes that were previously used for transforming F into S. It compiles previous design processes, initially experienced as sequences of design decisions, into a post hoc, explanatory account that ‘edits out false starts and imposes a spurious order on an indeterminate past’ (Weick Reference Weick1995). This distinction corresponds to Conklin and Yakemovic’s (Reference Conklin and Yakemovic1991) notions of ‘structure-oriented’ and ‘process-oriented’ design rationale, respectively. The compilation of a design process into a single step, from F to S, can be seen as an extreme form of structure-oriented design rationale.
Designing often involves a great deal of incompleteness and uncertainty: the requirements may be unclear, conflicting or incomplete, and the environment and its possible interactions with the artefact may be unknown. Process
$2^{\prime }$
can be a useful tool for commencing designing in these conditions by providing heuristics (Gigerenzer & Goldstein Reference Gigerenzer and Goldstein1996) to come up with design structures. The use of heuristics has been linked to creative concept generation (Yilmaz et al.
Reference Yilmaz, Daly, Seifert and Gonzalez2015). However, designers should be wary of the fallacies of system 1 executing these heuristics (Bulleit Reference Bulleit2013), as heuristic knowledge may be too simplified or based on insufficient information about the ranges of applicability. As system 1 is concerned only about the coherence but not the quality or robustness of the responses it generates, system 2 should become involved to avoid potentially serious design errors. It does so by monitoring the results of system 1. In the FBS ontology, this corresponds to processes 1b (setting expectations about the behaviour), 3 (analysing the structure) and 4 (comparing expected and actual behaviour). In case of failure of the generated structure (S) to meet the expectations, a new structure needs to be generated. This can be done in one of two ways: (1) by selecting the second most grounded pattern or (2) by reformulating structure based on reflection (Schön & Wiggins Reference Schön and Wiggins1992).
The first of the two ways of generating new structure is executed by system 1 because it is still based on pattern matching even though the most grounded pattern (which produced the failed S) is now ‘blocked’. In the FBS ontology, this process is captured by applying process
$2^{\prime }$
iteratively. It can be viewed as a new attempt of system 1 to propose an S that is part of a pattern linked to F.
The second way of producing new S is by reformulation, which, in the FBS ontology, is represented by process 6. As it is a reflective process, it is executed by system 2. The relationship between processes
$2^{\prime }$
and 6 is again one of subsumption, with the necessary condition that there already pre-exists a structure, as highlighted in Figure 6:

where
$\mathit{S}^{\text{prev}}$
is a previous structure.
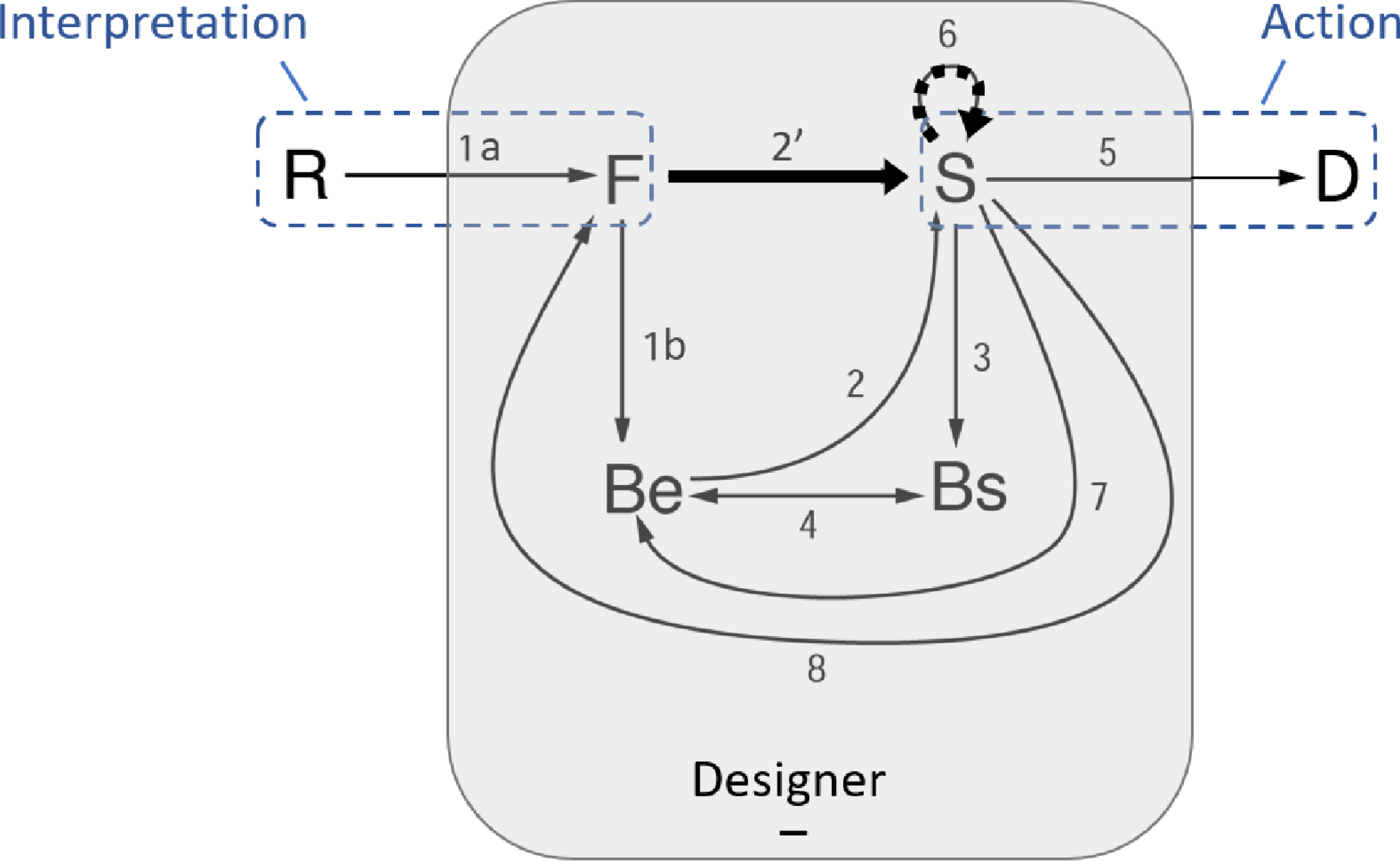
Figure 6. After failure of the initial S, process
$2^{\prime }$
(executed by system 1) can be used again by selecting the second most grounded pattern to be matched. Process
$2^{\prime }$
(bold, solid arrow) subsumes process 6 (bold, dotted arrow).
Process
$2^{\prime }$
can be executed iteratively, each time proposing a new structure that is first monitored and evaluated and, if it fails the evaluation, eventually replaced by another structure proposal. This corresponds to a process of search, in which a given (i.e. grounded) set of structure candidates is examined one by one, until a satisfactory candidate is found.
6 Instances of fast and slow design thinking
In this section, we illustrate the framework of fast and slow design thinking using four instances of designing: design fixation, case-based design, pattern-language-based design and brainstorming. They were chosen for two reasons: first, they all include some aspects of fast, intuitive (system 1) thinking, as described in Section 3. This sets them clearly apart from mainstream models of designing that emphasise slow, analytical (system 2) thinking. Similar to Kahneman’s book that is mainly about system 1 thinking, we see the main contribution of this paper in a better understanding of system 1 design thinking. The selection of the four instances of designing reflects this emphasis on system 1.
Second, the four instances are located within a wide spectrum of granularity where overlaps across instances may be possible. For example, design fixation may be found within case-based design, and brainstorming may be used as a means to reduce the effects of design fixation. Further, all instances may be used in conjunction with other (even mainstream) models of designing not presented in this paper. They are related to routine design (design fixation, case-based design and pattern-language-based design) and non-routine design (brainstorming). Based on their ubiquity in various models of design, the four instances cover a large range of design processes related to system 1 thinking.
6.1 Design fixation
Design fixation is the premature commitment on a design concept early in the process of designing (Jansson & Smith Reference Jansson and Smith1991). This is often perceived as an obstacle for design creativity. Fixation has been attributed to an ‘overactive’ system 2 (Moore, Sauder & Jin Reference Moore, Sauder and Jin2014) that aims to transform initial ideas in an analytic manner (Sowden, Pringle & Gabora Reference Sowden, Pringle and Gabora2015) without considering alternative options. We can use the FBS ontology to describe the role of the two systems in design fixation in more detail.
Fixation starts with the generation of structure (S) (or its presentation to the designer by an external source; for example, as a precedent) as a potential solution to a design problem. For fixation to occur, it is assumed that the structure causing that fixation is highly grounded and there is a strong connection between F and S. In the FBS ontology, this corresponds to process
$2^{\prime }$
(i.e. system 1).
Once S has been produced, it is used as the basis for generating further structures that can be viewed as elaborations of the initial S (e.g. decompositions). This is represented by process 6. It includes analytic forms of reasoning executed by system 2.
The processes involved in design fixation are summarised in Table 2.
Table 2. Processes involved in design fixation
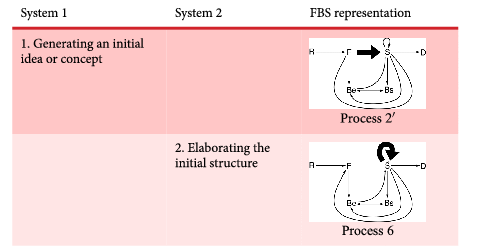
6.2 Case-based design
Case-based design is an application of the artificial intelligence technique of case-based reasoning in the design domain (Kolodner Reference Kolodner1987; Maher, Balachandran & Zhang Reference Maher, Balachandran and Zhang1995). It is based on retrieving and adapting existing design cases stored in memory (also called ‘case base’). A design case is a specific past design episode including the problem, the solution and the process for arriving at the solution (Rosenman, Gero & Oxman Reference Rosenman, Gero, Oxman and Schmitt1991). Case-based design involves the following phases: problem anticipation, search, match, retrieve, select, modify and repair (Maher et al. Reference Maher, Balachandran and Zhang1995).
Problem anticipation: enriches the given information about the design problem (i.e. the requirements, R) with as much implicit information as possible. This is captured by process 1a in Figure 4.
Search, match and retrieve: this phase aims to find an appropriate design case based on the problem description (in terms of F) and information associated with it within the design cases. This corresponds to process
$2^{\prime }$
that is executed by system 1.
Select: determines the ‘best’ matching design case among the retrieved ones. This can be represented using processes 3 and 4, as they are concerned with analysing and evaluating alternative designs.
Modify: involves some adaptations of S to enhance the fit with the specific design situation at hand. This can be represented using process 2, where the new S is subsumed by the previous S.
Repair: is used after some performance failure of the design. This is done either by retrieving and selecting a different design case or by modifying the existing design case. The former is captured by process
$2^{\prime }$
(i.e. system 1) and the latter by process 6 (i.e. system 2). The new S produced by process 6 is subsumed by the previous S.
The processes involved in case-based design are summarised in Table 3.
Table 3. Processes involved in case-based design
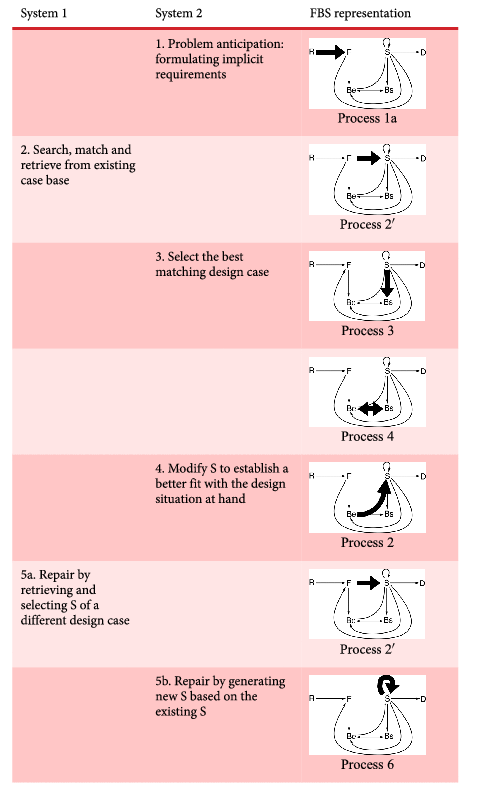
6.3 Pattern-language-based design
The concept of a pattern language was introduced by Alexander et al. (Reference Alexander, Ishikawa, Silverstein and Jacobson1977) in the architectural design domain and has since been applied in various other domains including software design (Gamma et al. Reference Gamma, Helm, Johnson and Vlissides1995), process design (Lerner et al. Reference Lerner, Christov, Osterweil, Bendraou, Kannengiesser and Wise2010) and educational design (Goodyear Reference Goodyear2005; Dehbozorgi et al. Reference Dehbozorgi, MacNeil, Maher and Dorodchi2018). Patterns are descriptions of solutions to recurring problems, including information about context and rationale. A pattern language is a representation of the interconnections between a collection of patterns.
Designing using a pattern language commences a search for potentially applicable patterns based on the problem represented as function (F). This search yields a pattern that contains a solution in terms of structure (S), leading to a direct transformation of F into S according to process
$2^{\prime }$
(which, therefore, is system 1 thinking). Considering the rationale included in the pattern corresponds to processes 3 and 4 that are executed by system 1. In case a potential pitfall is identified, a new search is carried out to identify a pattern that addresses the pitfall as a problem. This can be viewed as a reformulation of structure by means of a new instance of process
$2^{\prime }$
(i.e. system 1).
The processes involved in pattern-language-based design are summarised in Table 4.
Table 4. Processes involved in pattern-language-based design

6.4 Brainstorming
Brainstorming is one of the most known creative design techniques (Cross Reference Cross2000). It is based on bringing together a diverse group of people with a wide range of expertise and capturing their spontaneous ideas about a problem without assessing them.
A brainstorming session commences with the group leader formulating the problem statement. This can be represented as process 1a.
The group members then write down the first ideas that come to their minds. Every idea needs to be articulated succinctly so that it fits on a small card. This corresponds to multiple instantiations of process
$2^{\prime }$
(executed by system 1).
The next stage is the presentation of every idea to the group. No criticism or evaluation is allowed yet. The group rather reflects on the ideas, trying to develop them further or combining them. This can be viewed as process 6.
Only later does the group leader assess the ideas, which corresponds to processes 3 and 4.
The processes involved in brainstorming are summarised in Table 5.
Table 5. Processes involved in brainstorming
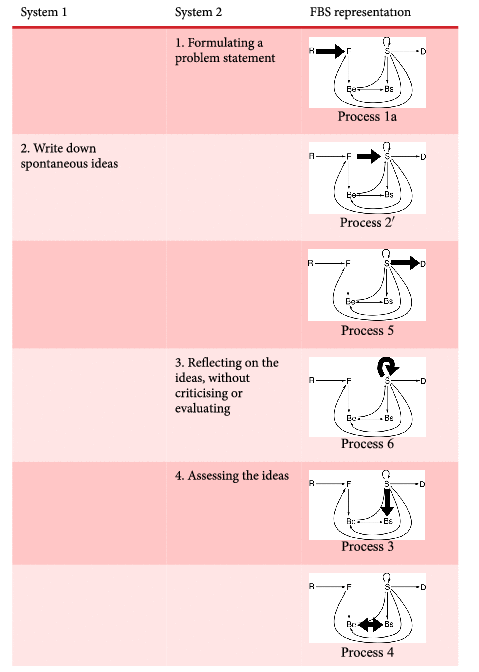
7 Discussion
The four instances of designing illustrate the framework of systems 1 and 2 design thinking developed in this paper. This set of instances is not exhaustive, as there are many more instances where system 1 design thinking can be shown. However, even from the limited set of examples in this research, some lessons can be learned.
One insight can be derived from analysing the four instances of design processes. Three of them – design fixation, case-based design and pattern-language-based design – are instances of routine designing and one of them – brainstorming – is an instance of non-routine (or creative) designing. Yet, in all of them, system 1 plays a distinctive role. In routine designing, system 1 merely reproduces existing design structures, while in non-routine designing, it generates new design structures. This is consistent with Taura and Nagai’s (Reference Taura and Nagai2017) distinction between ‘experiential’ and ‘associative’ intuition and their respective effects on the creativity of the designs produced using these forms of intuition. It is also consistent with Kahneman’s (Reference Kahneman2011) presentation of system 1, on the one hand, as a source of bias towards known experiences and, on the other hand, as a ‘mental shotgun’ that produces more (associative) concepts than it is asked for.
Another observation concerns the relationship between system 1 and system 2 design thinking. In all but one (namely, design fixation) of the four instances in Section 6, the results of system 1 executing process
$2^{\prime }$
are subsequently analysed (process 3) and evaluated (process 4). This is consistent with Kahneman’s claim that system 2 monitors and assesses the decisions made by system 1.
The framework developed in this paper characterises system 1 design thinking only as a transformation of F into S. This is because it is the activity with the highest cognitive load since it involves multiple processes – as a fast response of a designer producing a structure, given a required function. This can be viewed as a black box, top-level model of system 1 in design. Yet, further characterisations are possible locating it on a more fine-grained level. For example, the evaluation of a design (process 4) may be carried out either fast or slow too. The same holds for the formulation of expected behaviours (process 1b) or any of the other eight fundamental processes.
8 Conclusion
Connecting dual-system theory with the FBS ontology of design contributes to the increasing body of research in aligning models of cognitive science and design science. The paper has shown that system 1 and system 2 thinking can be represented ontologically and mapped onto various instances of design thinking. Dual-system theory can be viewed as a meta-cognitive model of human thinking (Barr Reference Barr and Pennycook2018), similar to the FBS ontology that is a meta-cognitive model of design thinking. They are generic and therefore independent of the specific domains or the specific instances of thinking and design thinking. This has the advantage that the specifics can be analysed, compared and discussed using a common terminology and common methods. Cross-domain and cross-instance insights can potentially be gained from such a uniform framework.
The combination of the dual-system theory and the FBS ontology required an extension of the original FBS ontology by adding a direct transformation of F into S. This does not represent a violation of the ‘no-function-in-structure’ principle (De Kleer & Brown Reference De Kleer and Brown1984). The new process (
$2^{\prime }$
) is defined in this paper as a substitution of other processes in the ontology for reasons of cognitive efficiency. Yet, it originates from previous design experiences that originally proceeded from function to expected behaviour and then to structure.
Embedding process
$2^{\prime }$
in the existing FBS ontology has the benefit that empirical findings can now be better accounted for, especially the frequently observed direct transformation of F into S. For example, Yu & Gero (Reference Yu and Gero2016), using a Markov model analysis of design protocols in the context of parametric design, observed a high frequency of F-to-S transformations based on the use of design patterns. Having an explicit process representing this transformation opens up the possibility of revisiting other design protocols and analysing them for the occurrence of system 1 design thinking. This may also empirically validate the ontological model of fast and slow design thinking presented in this paper. Initial results from design protocols of professional engineers and engineering students are available that confirm this model of design thinking that includes system 1 thinking (Kannengiesser & Gero Reference Kannengiesser, Gero, Eriksson and Paetzold2019).
Future design research may use this work for developing new methods and tools for performing and teaching design. This is because it provides access to well-established findings in the various sub-disciplines of cognitive psychology. For example, methods for analysis and evaluation in design may benefit from cognitive approaches that help overcome the laziness of system 2 to avoid biased judgements due to system 1. This gap is shown in Section 6: design fixation occurs because there is a lack of analysis (process 3) and evaluation (process 4) of the structure produced by process
$2^{\prime }$
. Therefore, research may develop tools that stimulate analysis and evaluation activities by system 2 and possibly feed the results back to system 1 for improved generation of structure in future design tasks.
Another possible extension of this work is to investigate the usefulness of Stanovich’s (Reference Stanovich, Evans and Frankish2009) ‘tri-process theory’ for design thinking. This theory splits system 2 into two modes or processes: an algorithmic and a reflective process. The resulting set of three processes may be mapped onto Maher and Gero’s (Reference Maher and Gero2002) reflexive, reactive and reflective modes of reasoning for situated design agents.
Early studies in applying the artificial intelligence technique of machine learning to design resulted in the encoding of B to S, which today would be called system 1 design thinking (Gero & Marmot Reference Gero and Marmot1974). A new range of computational design tools based on deep learning (LeCun, Bengio & Hinton Reference LeCun, Bengio and Hinton2015) are under development and are all examples of system 1 design thinking. They find relationships between F and S and between B and S from large bodies of design data (McComb Reference McComb and Gero2019).
Acknowledgments
This work is supported in part by grants from the US National Science Foundation, grant nos: CMMI-1400466, EEC-1463873 and CMMI-1762415. Any opinions, findings and conclusions or recommendations expressed in this material are those of the authors and do not necessarily reflect the views of the National Science Foundation.