Introduction
Jamestown Canyon virus (JCV) and snowshoe hare virus (SHV) are two arboviruses belonging to the California serogroup (Family Bunyaviruses, Genus Orthobunyavirus). Both viruses have been associated with cases of febrile and neuroinvasive disease in humans in North America. Although exposure to California serogroup viruses (CSGV) often does not result in disease or causes only mild symptoms, JCV infection can result in moderate to severe encephalitis in adults which can be accompanied by respiratory symptoms, while severe SHV infections have been mostly identified in young patients who display neurologic symptoms [Reference Drebot1, Reference Calisher2]. Recent information suggests that infection by these viruses may be underreported and could be increasing [Reference Drebot1, Reference Kulkarni3]. In Canada, from 1994 to 2008, no aetiological agent was identified in approximately 50% of the patients hospitalised with encephalitis [Reference Kulkarni4]. In some Canadian provinces, including Québec, the incidence of these idiopathic encephalitis cases seems to peak during summer months, leading to a hypothesis that some of these cases could represent undetected infections by arboviruses, including CSGV [Reference Kulkarni4]. Epidemiological reports and serological surveys conducted in different locations suggest that humans are frequently exposed to CSGV in North America [Reference Kosoy5–Reference Lindsey8].
JCV is thought to be amplified in a transmission cycle involving wild ungulates such as white-tailed deer and a variety of mosquito species, while for SHV the reservoir hosts are small mammals such as hares, squirrels and chipmunks [Reference Issel9–Reference Ksiazek and Yuill13]. High seroprevalence rates have been reported for both viruses in different wild mammal species from North America [Reference Goff, Whitney and Drebot14–Reference Nelson20], suggesting that natural transmission cycles can be highly effective in maintaining CSGV activity. However, there has been little effort to assess which types of environment present a high risk of exposure to these viruses.
From 2012 to 2014, we conducted a serological survey for arboviruses in human and pet dog populations from southern Québec. Previous studies reporting high seroprevalence estimates to various arboviruses in dogs suggested that dogs could represent a sensitive sentinel population for arboviral activity [Reference Rocheleau21]. Although dogs are not clinically affected by CSGV, using them as sentinels may present some advantages for studying environmental risk factors for arboviruses that rarely manifest clinically in humans as it is the case for CSGV. Considering that antibodies against arboviruses may persist for many years [Reference Rocheleau21], studying risk factors for seropositivity in humans may be challenging because the time and place of the infection that lead to seroconversion are often unknown. Considering that dogs can be accessed through veterinary clinics, serology in very young dogs could provide a relatively precise spatiotemporal overview of arboviral activity [Reference Rocheleau21].
In Québec, 2012–2014, regional seroprevalence estimates ranged from 10% to 33% in humans, 0% to 20% in adult dogs and 0% to 10% in dogs less than 1-year-old for JCV and SHV combined [Reference Rocheleau21]. Although only a few indigenous human clinical cases of Jamestown Canyon encephalitis have been reported from the province of Québec [22], these results suggested significant and ongoing CSGV transmission in this region. Based on the serological data in Rocheleau et al. [Reference Rocheleau21], the current study aimed at assessing the geographical distribution and identifying risk factors for seropositivity to these two arboviruses in humans and pet dogs.
Material and methods
Study design and study area
A cross-sectional study was conducted in five administrative regions of southern Québec: Montréal, Laval, Montérégie, and the southern parts of Lanaudière and Laurentides (Fig. 1). The city of Montréal, in the centre of the study area, is surrounded by suburban areas themselves surrounded by flat agricultural land and rolling hills mainly covered by hardwood and mixed forests. The study area also includes numerous wooded and non-wooded wetlands and islands of coniferous forests.
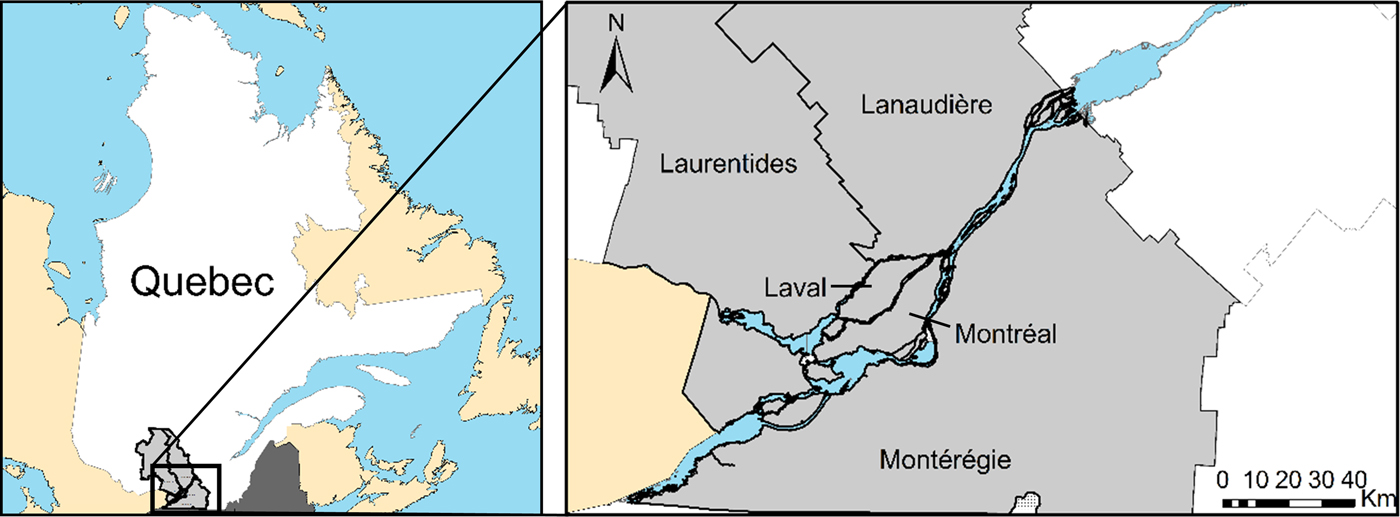
Fig. 1. Study area.
Sampling
Sampling was conducted in the context of a broader serological survey for various arboviruses [Reference Rocheleau21]. A total of 1627 dogs were first sampled in 2013 by veterinarians from 89 veterinary clinics distributed through the study area. In 2014, all adult humans living in the same household as a dog sampled in 2013 were contacted by phone and asked to participate in a human serosurvey. A total of 485 persons agreed to participate and were included for blood sampling. The sample size required to detect a statistically significant odds ratio of 2 between two independent groups of equal size for expected overall CSGV seroprevalence estimates between 15% and 20% was ranging between 344 and 416, given an alpha of 0.05 and statistical power of 0.80 (SAS Power and Sample size, SAS Institute Inc., Cary, NC, USA). Thus, a subsample of 400 humans was randomly selected for CSGV testing. These humans lived in 321 different households. The sera from all dogs (314) living in the same households as these 400 human participants were then tested for CSGV antibodies. In order to achieve a sample size with sufficient statistical power, an additional 88 dogs, randomly selected from our previous study, were also included for serology to reach a total of 402 dogs tested [Reference Rocheleau21]. Only one human per household was then randomly selected for inclusion in the statistical models to avoid clustering. Each of the selected dogs came from a different household and all tested dogs were included in the statistical models. All these dogs’ sera were collected between May and October 2013. Adult dogs (born before November 2012) were sampled mainly in June and juvenile dogs (born after November 2012 and assumed not to have been exposed to mosquitoes before the sampling period) were sampled from July to October in participating veterinary clinics.
Laboratory analysis
The detailed protocol for serological testing was described in Rocheleau et al. [Reference Rocheleau21]. Briefly, samples were screened for antibodies against JCV and SHV by a competitive ELISA (cELISA) developed at the National Microbiology Laboratory (NML) of the Public Health Agency of Canada. All canine and a subset of human CSGV seropositive samples were then tested by a plaque reduction neutralisation test (PRNT) to assess the proportion of antibodies that were specific to JCV or SHV. Overall, 80% and 84% of the sera that tested positive on the cELISA were confirmed to be positive when tested by PRNT in humans and dogs, respectively. However, more than 25% of the sera that were positive on the PRNT could not be specifically assigned to JCV or SHV, which may have been due to previous exposures to both viruses [Reference Rocheleau21]. For the current study, a positive cELISA result was used for classifying a subject as seropositive.
Risk factor data collection
Questionnaire
A pretested written questionnaire about factors potentially associated with exposure to mosquitoes was administered to each pet dog owner after blood collection from them and from their pet dog. Data sought in the questionnaire for humans included the address of each participant at time of sampling and information about age, sex, ethnical origin, outdoor professional activities, education, average time spent outside daily during summer at home and away from home in forested, agricultural, urban or suburban areas, average time spent daily with the dog which participated in the canine serological survey, average number of mosquito bites per week, use of protective clothing to prevent mosquito bites, use of mosquito repellents, number of mosquitoes noticed inside the house weekly and presence of pools of standing water on their property or on the neighbours’ properties. Data acquired in the questionnaire administered to owners about their dogs included the address of the property where each of the dogs lived at time of sampling and information about age, sex, breed, hair length, use of mosquito repellent, flea medication or insecticide, time spent outside daily, presence and size of pools of standing water on the property and time spent outside the municipality of residence during the mosquito season.
Land cover data
Land cover classification was obtained from the Ecoforest Inventory System of the Québec Ministry of Forests, Fauna and Parks (Ministère de la forêt, de la faune et des parcs – MFFP), updated in 2013 (Fig. 2a). For each human and each dog, land cover variables were calculated as the percentage of the area covered either by hardwood, coniferous or mixed forest, developed (urban, suburban or industrial), agricultural or disturbed land, and surface water or wetlands inside a circular area of 2 km radius centred on the house location. This buffer radius was based on average dispersal distances for some mosquito vector species [Reference Service23].
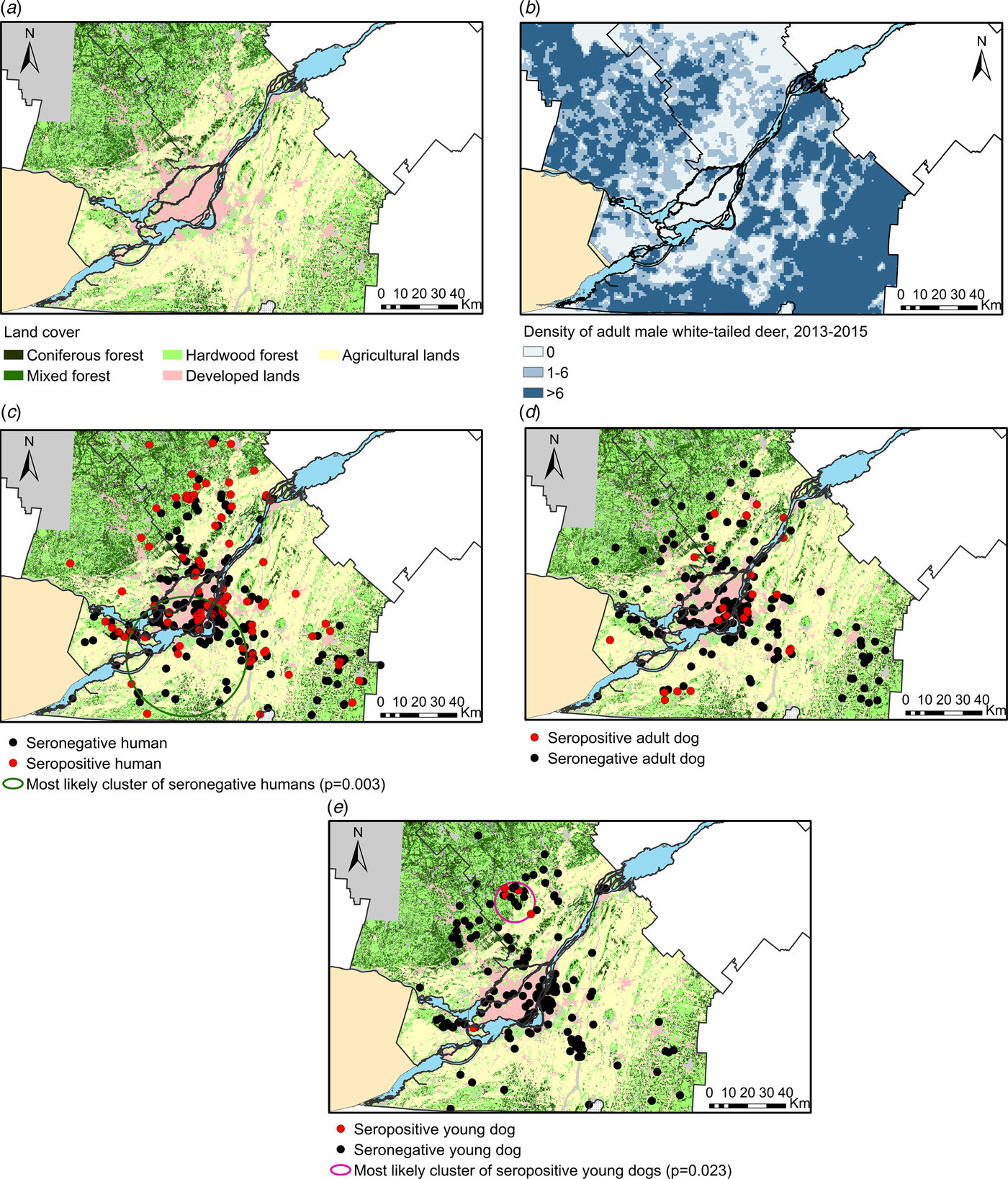
Fig. 2. Geographical distribution of land cover types and study subjects, Québec, Canada, 2013–2014. (a) Main land covers distribution; (b) Density of adult white-tailed deer (number of harvested adult male white-tailed deer (O. virginianus) per 2 km radius area) across the study area for the 2013–2015 period; (c–e) Sampling distribution of participating humans, adult dogs and juvenile dogs and most likely clusters of seropositive or seronegative subjects (no statistically significant clusters were identified in adult dogs).
White-tailed deer densities
Given that white-tailed deer are expected to be the main amplifying hosts of JCV in southern Québec, the geographical location of harvest sites of adult male white-tailed deer (Odocoileus virginianus) during the sport hunting seasons 2013 to 2015 were provided by the Québec Ministry of Forests, Fauna and Parks (Fig. 2b). The number of white-tailed deer harvested within a 2 km radius from each participant's house was taken as a proxy for white-tailed deer density in the area surrounding each residence [Reference Daigle24, Reference Norton25].
Statistical analyses
Logistic regression was used to model CSGV seropositivity as the outcome variable according to individual and environmental factors in humans and dogs (adults and juvenile combined). Pairwise correlations among variables of interest were assessed by the Pearson correlation coefficient (r) for continuous risk factors and by the Phi coefficient for categorical risk factors. When correlation coefficients were over 0.7, one of the two correlated risk factors was selected on the basis of greatest biological relevance. Univariate regression was used to model unconditional associations between each of the remaining risk factors and the CSVG seropositivity as the outcome variable. For continuous risk factors, the assumption of linearity was assessed by plotting a loess smooth curve against the Pearson's residuals of the univariate models. If the assumption of linearity was rejected, risk factors were categorised according to their quartile values and, when biologically relevant, adjacent categories that were not significantly different (P < 0.05) from each other were merged. However, when categorisation of a continuous environmental risk factor was required in dogs, the same categories as those used in humans for this risk factor were chosen to allow for comparisons between outputs from models using data from humans and dogs. All risk factors showing an association with the outcome variable at P < 0.20 were included in a full multivariable model which was refined by a stepwise backward process with a cut-off at P > 0.05 for rejection. Two-way interactions between the risk factors of the final models were tested one at a time when deemed biologically relevant. Models’ predictive ability and fit were assessed by the area under the ROC curve and by the Hosmer and Lemeshow fit statistic, respectively.
Spatial scan statistics (SaTScan v.9.3.1) [Reference Kulldorff26] were used to explore potential high risk and low-risk geographical clusters of seropositive subjects. The most likely statistically significant clusters were mapped in ArcGIS 10.2.2 (ESRI, Redlands, CA, USA). The scan statistics were based on a Bernouilli distribution with a maximum cluster size representing 50% of the total sample. Cluster analyses were conducted separately on the human and dog datasets. Given that the uncertainty about the geographical location of exposure was expected to be proportional to the age of sampled subjects, we also conducted a cluster analysis including only juvenile dogs to maximise the geographical precision of the analysis for the year 2013.
The study protocol in dogs was approved by the Committee for ethical animal use of the Université de Montréal (protocol 12-Rech-1698) and the study protocol in humans was approved by the Committee for ethical health research of the same university (protocol 13-130-CERES-D).
Results
Correlation analysis revealed that time spent outside weekly by human participants was highly correlated with time they spent weekly with their dog (r = 0.77). Mixed forest was also highly correlated with coniferous forest and hardwood forest (r = 0.71 and 0.60, respectively). Hence, the variables ‘Time spent with the dog weekly’ and ‘Mixed forest’ were excluded from the regression analysis.
Results of univariate regressions for individual variables are presented in Tables 1 and 2 in humans and dogs, respectively. In both species, the amount of time spent outside daily was positively associated with seropositivity. In humans, being male, being frequently bitten by mosquitoes, frequently observing mosquitoes inside the house and spending more than 2.5 h weekly away from home within suburban areas were also positively associated with seropositivity. In dogs, large breeds and dogs 1-year-old or more had higher odds of seropositivity.
Table 1. Results from univariable logistic regression modelling seropositivity to CSGV associated with individual factors in 321 humans from Québec, Canada, 2014
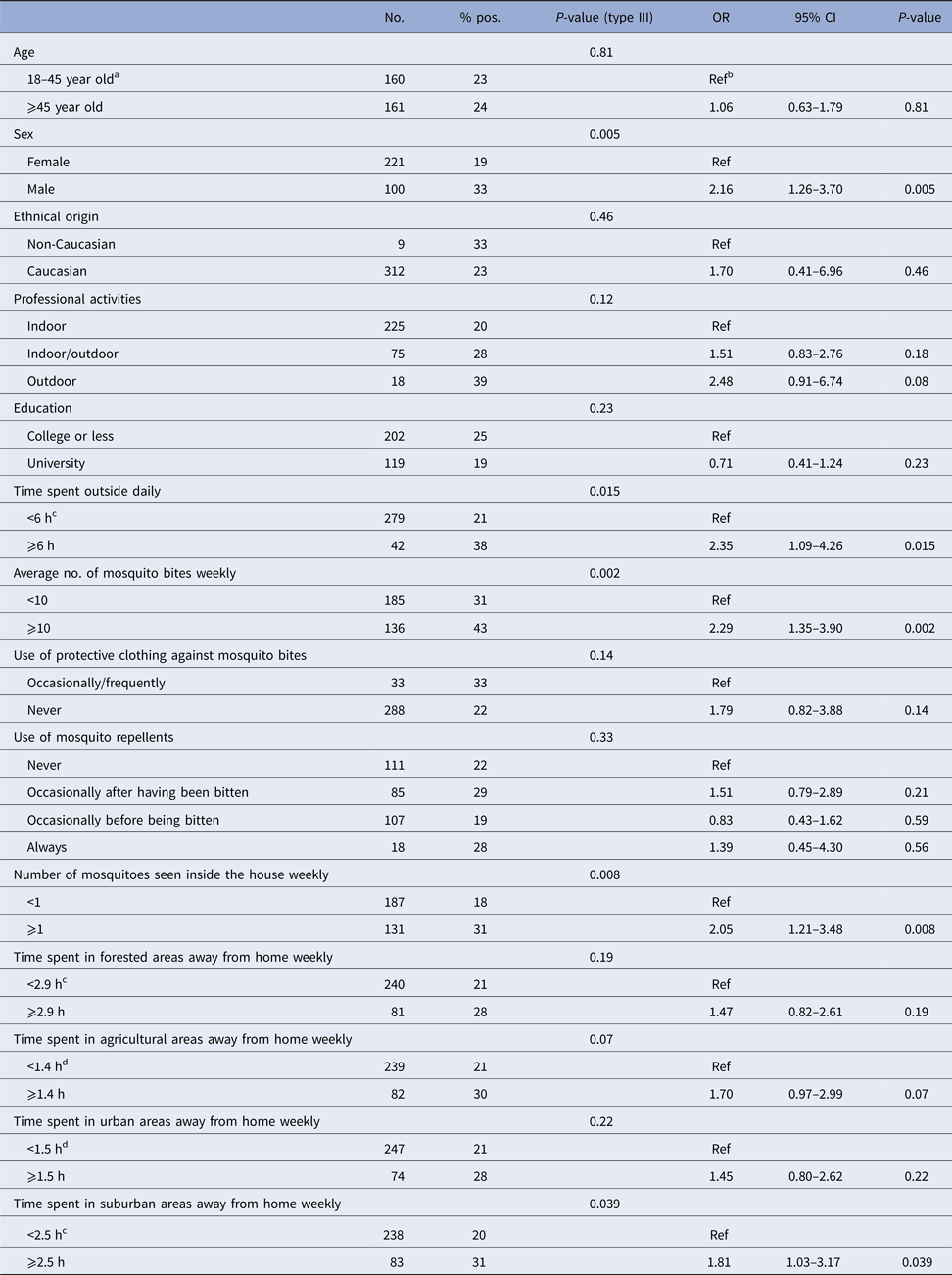
a Median as category cut-off.
b Reference category.
c The first three quartiles were grouped as no statistically significant difference was observed between these categories.
d The first three quartiles were grouped as the median value was 0.
Table 2. Results from univariable logistic regression modelling seropositivity to CSGV associated with individual factors in 402 dogs from Québec, Canada, 2013
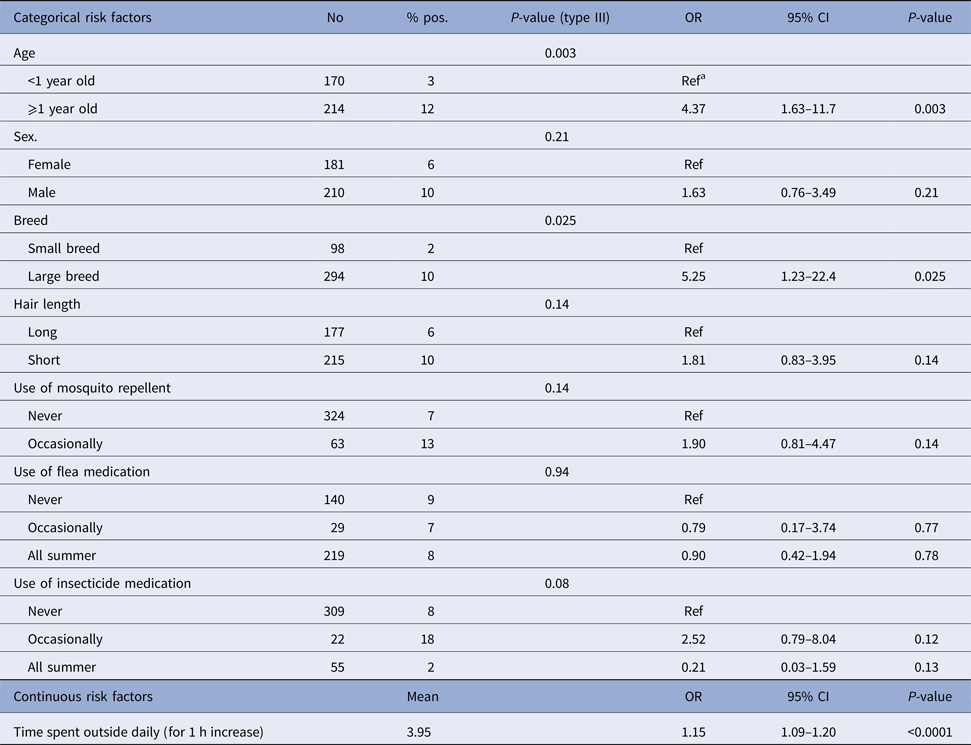
a Reference category.
Results of univariate regressions are presented in Tables 3 and 4 for environmental risk factors in humans and dogs, respectively. In both species, living in or nearby a forested area (specifically hardwood forests in humans and coniferous forests in dogs) and higher densities of white-tailed deer were positively associated with seropositivity. In both humans and dogs, the developed land was identified as a lower risk environment. The presence of pools of standing water on the property was positively associated with seropositivity in humans while living on or nearby agricultural land was positively associated with seropositivity in dogs.
Table 3. Results from univariable logistic regression modelling seropositivity to CSGV associated with environmental factors in humans from Québec, Canada, 2014
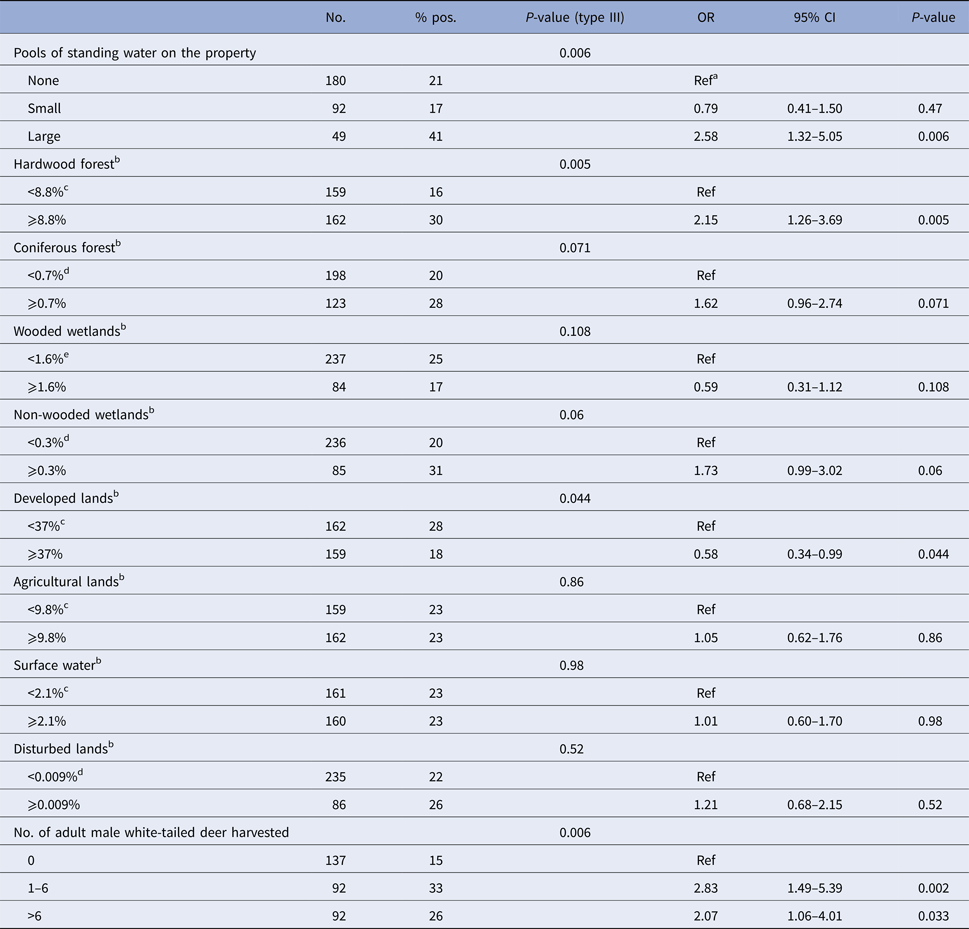
a Reference category.
b Percentage of the area covered by each land cover within a radius of 2 km radius centred on the house.
c Median as category cut-off.
d The first three quartiles were grouped as the median value was 0.
e The first three quartiles were grouped as no statistically significant difference was observed between these categories.
Table 4. Results from univariate logistic regression modelling seropositivity to CSGV associated with environmental factors in dogs from Québec, Canada, 2013
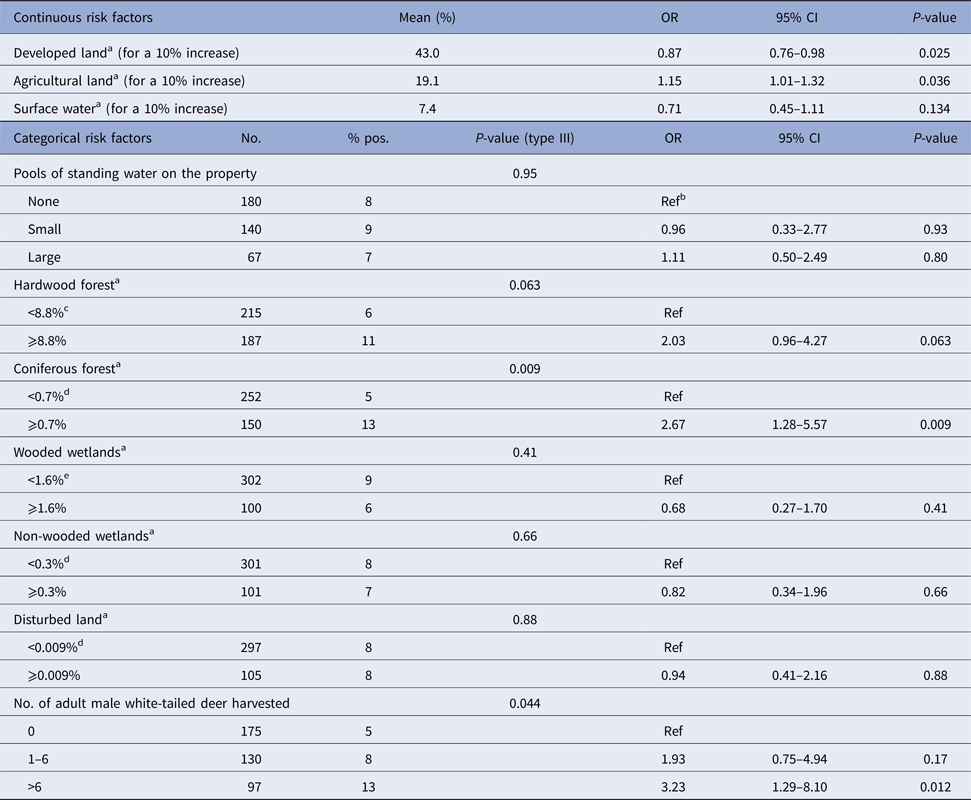
a Percentage of the area covered by each land cover within a radius of 2 km radius centred on the house.
b Reference category.
c Median as category cut-off.
d The first three quartiles were grouped as the median value was 0.
e The first three quartiles were grouped as no statistically significant difference was observed between these categories.
Results of the final multivariable models are presented in Table 5 for humans and in Table 6 for dogs. In humans, males had higher odds of seropositivity than females (OR 2.03, P = 0.016). The density of white-tailed deer was kept as a risk factor in the multivariable model. However, only the middle category (1–6 deer in the area surrounding the house) compared to the absence of deer had a positive and statistically significant association with seropositivity (OR 2.50, P = 0.009). There was a statistically significant interaction (P = 0.008) between the weekly number of mosquito bites and the presence of hardwood forest around the house. When controlling for the other variables in the multivariable model, the odds of being seropositive was 4.44 times higher in highly forested areas compared with less forested areas in people reporting more than 10 mosquito bites weekly (P = 0.004). There was no significant difference between highly forested areas compared to less forested areas in people reporting less than 10 mosquito bites per week (OR 0.99, P = 0.98). Moreover, the odds of being seropositive was 3.88 times higher in people reporting more than 10 mosquito bites weekly and living in highly forested areas (P = 0.0006). This odds ratio was not statistically significant in people living in less forested areas (OR 0.70, P = 0.48). In dogs, the amount of time spent outside daily (OR 1.15 for each increment of 1 h spent outside daily, P < 0.0001) and coniferous forests around the house (OR 2.39, P = 0.04) were associated with higher odds of exposure to CSGV in the multivariable model. Dogs 1-year-old or more also had higher odds of seropositivity than dogs less than 1-year-old (OR 5.62, P = 0.002). The area under the ROC curve (AUC) was 0.73 and the Hosmer and Lemeshow (HL) P-value was 0.84 for the multivariable model in humans. The AUC was 0.84 and the HL P-value was 0.75 for the multivariable model in dogs. These statistics suggested an acceptable predictive ability and fit for both models.
Table 5. Final multivariable logistic model for seropositivity to California serogroup viruses associated with individual and environmental factors in humans from Québec, Canada, 2014
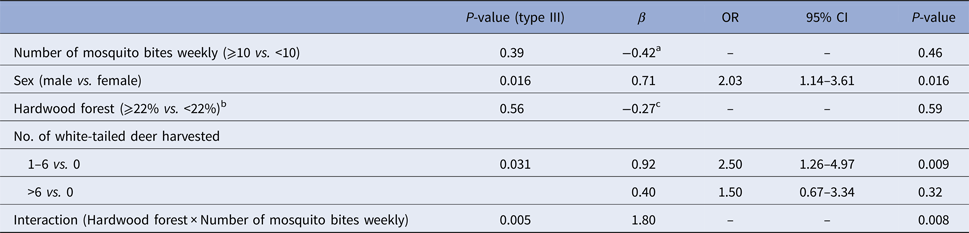
a Coefficient value computed with Hardwood forest<22%.
b Percentage of the area covered by each land cover within a radius of 2 km radius centred on the house.
c Coefficient value computed with Number of mosquito bites<10.
Table 6. Final multivariable logistic model for seropositivity to California serogroup viruses associated with individual and environmental factors in dogs from Québec, Canada, 2013
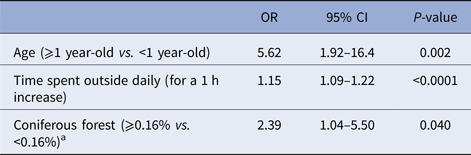
a Percentage of the area covered by each land cover within a radius of 2 km radius centred on the house.
When conducted on the whole sets of human or dog data, no statistically significant geographical clusters of seropositive subjects were identified. However, cluster analysis identified a cluster of seropositive juvenile dogs (P = 0.023), located in a mainly forested area of southern Lanaudière. According to PRNT results, the four seropositive juvenile dogs located within this cluster were all positive for JCV and negative for SHV. Blood samples were taken from these dogs between 16 July and 9 September 2013. A cluster of seronegative humans (P = 0.003) included some agricultural lands of Montérégie and a part of the city of Montréal. Clusters are presented in Figure 2.
Discussion
This study is the first to identify environmental factors responsible for the spatial variation in risk of CSGV infections in humans in Canada. Our results suggest that risk of infection in humans would be higher in hardwood forests while the risk in dogs would be higher in coniferous forests. Current biological knowledge on JCV and SHV suggests that forested areas would likely offer favourable breeding habitats for the main mosquito vector species responsible for CSGV transmission as well as for CSGV main reservoir hosts (deer, hares and woodland rodents). According to previous entomological studies, JCV and SHV are transmitted by a variety of Aedes (Ochlerotatus (Oc.)) species, as well as by Culiseta morsitans, Culiseta melanura, Anopheles punctipennis and Coquilletidia perturbans [Reference Goff, Whitney and Drebot14, Reference Andreadis27–Reference Rust32]. Snow-melt mosquitoes have been previously identified as the most important vectors for JCV and some authors identified Oc. canadensis as being highly competent for JCV [Reference Andreadis29, Reference Rust32]. This vector, which takes a large proportion of its blood meals from humans and white-tailed deer, is widely distributed across forested areas of southern Québec and larvae of this species preferentially develop in acid soils of coniferous forests [Reference Maire and Aubin31, Reference Rust32]. The larvae of the other competent non-aedine mosquito vector species usually develop in swamps and bogs which, in Québec, are predominantly associated with forested land cover [Reference Crans33]. Hence, these vector distributions seem consistent with the spatial distribution of risk in humans and dogs. On the other hand, the geographical distribution of some Québec-endemic mosquito vectors of West Nile virus, which are known to be less competent for CSGV transmission (e.g. Ae. vexans, which is found in abundance in alluvial plains and agricultural lands, and Cx. pipiens, which is abundant in agricultural, urban and suburban areas), seems consistent with the areas of lower risk identified in the current study [Reference Artsob34, Reference Rocheleau35].
Grimstad et al. previously reported a correlation between white-tailed deer densities and seroprevalence for JCV in humans [Reference Grimstad36]. Although our results suggested that white-tailed deer density may be associated with risk of seropositivity, deer density was not linearly associated with seropositivity in humans and some areas with very high densities of deer, such as the south-eastern part of the study area, did not consistently correlate with areas of higher seropositivity (see Table 5 and Fig. 2). Moreover, despite the relatively high proportion of seropositive sera specifically assigned to JCV in dogs, the density of white-tailed deer was not retained in multivariable models in dogs, suggesting that factors other than deer density may be more dominantly influencing transmission of this virus to dogs. Snowshoe hare are inhabitants of the boreal forests and would be particularly abundant in the coniferous understory of the boreal forest [Reference Litvaitis, Sherburne and Bissonette37]. Hence, their distribution, although not precisely characterised in the context of this study, would also be consistent with the environmental risk factors identified here [Reference Litvaitis, Sherburne and Bissonette37].
The cluster of seropositive juvenile dogs identified in Southern Lanaudière was located in an area covered with agricultural lands and hardwood, mixed and coniferous forests where white-tailed deer densities were high. This cluster and the date of sampling of the seropositive juvenile dogs located within this cluster suggest that sustained and focalised JCV activity may occur and can be detected relatively early during summer in Quebec. The cluster of seronegative humans located in the greater Montreal area is consistent with lower CSGV activity in urban/suburban areas.
As suggested by the interaction term in the multivariable model in humans, high contact rates with mosquitoes would increase the risk of infection but particularly in forested areas. Thus, seropositivity to CSGV in humans could result from the joint effect of individual behaviours favouring contact with competent vectors in people living within specific high-risk environments. However, given that human-mosquito contact rates are also determined by mosquito species characteristics and feeding behaviour [Reference Rust32, Reference Chalvet-Monfray, Sabatier and Bicout38], it may also be possible that this interaction results from the higher aggressiveness of some specific competent vector species breeding in forested environments. This hypothesis would suggest the potential existence of some ‘super spreader’ vector species and would be worth considering in future entomological studies on CSGV. Seropositivity in humans was higher in males compared to females. This finding is consistent with the higher incidence of reported JCV clinical cases in males in the USA and has also been reported in other locations of Canada [Reference Grimstad36, Reference Pastula39].
As discussed in Rocheleau et al., the sensitivity and specificity of the cELISA used for classifying study subjects as seropositive or seronegative were not formally assessed in the context of the current study although validation studies performed indicated good correlation with the PRNT assay [Reference Rocheleau21]. Given that none of the individual or environmental factors modelled in this study were expected to be associated with, or to affect the performance of, the cELISA, any misclassification bias related to an imperfect sensitivity or specificity are expected to be non-differential and to bias the estimated associations towards the null. As discussed in Rocheleau et al., sampled humans and dogs were not randomly selected and the proportion of seropositive subjects may not be generalisable to Quebec's human and dog populations [Reference Rocheleau21]. However, the main factors usually affecting exposure to arboviruses were controlled for in the statistical models. Hence, we believe that the probability that the reported associations could have been confounded by unmeasured extraneous factors should be relatively low. Moreover, considering that clinical diseases related to CSGV were very rarely diagnosed in Quebec at the time of sampling and considering that participants were unaware of their serological status at the time they completed the questionnaire, we should expect that the reported associations have not been affected by a differential recall bias.
Although a greater proportion of seropositive subjects were encountered in or nearby forested areas, some seropositive subjects lived in agricultural, suburban and urban areas. This wide distribution may be related to various biological and behavioural factors. CSGV can be transmitted from an infected female vector to its progeny by transovarial transmission [Reference Turell, Reeves and Hardy40] which could possibly be responsible for a progressive geographical drift of infected vector populations away from the habitat of amplifying hosts. National, provincial or municipal parks found in the close vicinity of urban or suburban areas also support focal but abundant deer populations. Also, some hosts such as squirrels and other small mammals, which may amplify SHV, are commonly found in urban and suburban areas. Moreover, given that CSGV has been active for decades in the study area [Reference Artsob34], seroconversion may have occurred at any time in the past, in areas distant from the house location of participants, at time of the study. However, based on our previously published data on the cohort of dogs included in this study [Reference Rocheleau35], 61% of the sampled dogs had never left their municipality of residence, suggesting that exposure misclassification may be relatively limited in dogs [Reference Rocheleau35].
This study suggests that CSGV transmission is widespread in southern Quebec, a finding which is consistent with studies on CSGV in other regions of North America and is likely due to the ubiquity of reservoir hosts and vectors in many parts of the region. An increased awareness of the potential infection with CSGV viruses in patients presenting with symptoms of encephalitis and a history of frequent exposure to mosquito bites in forested areas of Québec would be warranted. Of importance is the identification of woodland habitats (vs. agricultural land and suburban environments) as risk environments for exposure to CSGV, which is the opposite to what has been found in Quebec and/or other parts of North America for WNV [Reference Andreadis27, Reference Turell28, Reference Rocheleau35]. Therefore, the risk from mosquito-borne arboviruses is present in many different habitats, but the precise type of risk varies with habitat. The use of personal protective behaviours could be promoted as an efficient approach for risk mitigation in populations living in forested as well as agricultural and suburban environments.
Acknowledgements
We thank all veterinarians and animal health technicians who contributed to sampling and all participants involved in this study. We also thank Caroline Doré who coordinated the human sampling. We also thank Kristina Dimitrova and Nicole Barairo at the NML for performing some of the serological assays. Funding for this study was provided by the Public health agency of Canada.