Introduction
Leishmania is an eukaryotic intracellular protozoan parasite that causes a broad spectrum of human diseases referred to as leishmaniasis, which currently affect about 12 million people and threaten more than 350 million globally (WHO, 2010). Of the three main pathological forms of the disease – cutaneous, mucocutaneous and visceral – the visceral form caused by L. infantum (in South America and in the Mediterranean) and L. donovani (India and parts of Europe and Africa) is the most severe. If left untreated, visceral leishmaniasis has a high fatality rate, ranking next to malaria as the deadliest protozoan human disease (Torres-Guerrero et al., Reference Torres-Guerrero, Quintanilla-Cedillo, Ruiz-Esmenjaud and Arenas2017). The life cycle of Leishmania parasites alternates between insect vectors and mammalian hosts, with intra-species transfer occurring through sand fly bites. Parasites in the promastigote phase are elongated in shape and have long, easily distinguishable flagella, whereas those in the amastigote phase are more spherical and have almost indistinguishable flagella. The transition from the flagellated, non-virulent promastigote insect stages to the rounded, virulent amastigote forms that replicate inside phagolysosomes of mammalian macrophages is critical for Leishmania virulence (Killick-Kendrick, Reference Killick-Kendrick1990), and remains the ‘holy-grail’ for the identification of virulence factors and therapeutic targets. The ability to successfully induce promastigote to amastigote differentiation in axenic culture by mimicking some of the intra-phagolysosomal conditions inside host macrophages (namely, low pH and elevated temperature) has greatly aided the understanding of cellular changes that accompany parasite differentiation (Bates et al., Reference Bates, Robertson, Tetley and Coombs1992; Zilberstein and Shapira, Reference Zilberstein and Shapira1994; Barak et al., Reference Barak, Amin-Spector, Gerliak, Goyard, Holland and Zilberstein2005). However, these temperature-/pH-dependent protocols for axenic differentiation and culture of amastigote forms have been difficult to adapt for many Leishmania species, including isolates of the clinically important L. infantum and L. donovani visceralizing species. Although the successful conversion of L. chagasi (same as L. infantum) promastigotes to amastigote forms in axenic culture was previously reported (Hsiao et al., Reference Hsiao, Yao, Storlie, Donelson and Wilson2008), several published methods for differentiating L. infantum promastigotes into amastigotes in axenic culture involved a pH shift that varied between 5.5 and 6.5 and a temperature elevation that ranged from 26 to 37 °C (Sereno and Lemesre, Reference Sereno and Lemesre1997; Somanna et al., Reference Somanna, Mundodi and Gedamu2002), and most studies lacked a demonstration that the parasites retained viability under these conditions. Sudden changes in pH and temperature have been reported to result in loss of Leishmania viability as a result of a high reactive oxygen species (ROS) surge (Alzate et al., Reference Alzate, Alvarez-Barrientos, Gonzalez and Jimenez-Ruiz2006, Reference Alzate, Arias, Moreno-Mateos, Alvarez-Barrientos and Jimenez-Ruiz2007).
In spite of the general perception that ROS radicals are largely harmful and deleterious for cells, it has become increasingly clear that low concentrations of ROS such as superoxide and hydrogen peroxide (H2O2) are essential for maintaining homoeostasis and for cell fate determination (Tsukagoshi et al., Reference Tsukagoshi, Busch and Benfey2010; Tatapudy et al., Reference Tatapudy, Aloisio, Barber and Nystul2017). The role of ROS molecules, particularly H2O2, as a differentiation signal has been demonstrated in many eukaryotic cells (Marinho et al., Reference Marinho, Real, Cyrne, Soares and Antunes2014; Sies, Reference Sies2014; Lennicke et al., Reference Lennicke, Rahn, Lichtenfels, Wessjohann and Seliger2015), including the non-visceralizing Leishmania species L. amazonensis (Mittra et al., Reference Mittra, Cortez, Haydock, Ramasamy, Myler and Andrews2013, Reference Mittra, Laranjeira-Silva, Miguel, Perrone Bezerra de Menezes and Andrews2017). H2O2-mediated signalling in L. amazonensis has been shown to play a critical role in the development of infectivity for bone-marrow-derived macrophages or mice, by promoting promastigote to amastigote differentiation independently of a shift in pH and temperature (Mittra et al., Reference Mittra, Cortez, Haydock, Ramasamy, Myler and Andrews2013). Successful differentiation into amastigotes form was observed under conditions that promote conversion of mitochondria-generated superoxide radicals into H2O2 by the iron-dependent superoxide dismutase (SODA), through a process that can occur metabolically or via iron depletion from the culture medium (which results in an increase in iron uptake by upregulating transporters). Axenic differentiation into amastigotes was also achieved by directly treating L. amazonensis promastigotes with H2O2 or sub-lethal concentrations of menadione, a drug that generates mitochondrial superoxide (Mittra et al., Reference Mittra, Cortez, Haydock, Ramasamy, Myler and Andrews2013).
In this study, we examined if H2O2-mediated signalling is also involved in triggering amastigote differentiation of other Leishmania species, in particularly the medically important visceralizing species. Our study was inspired by a previous study that reported significant enhancement of virulence in mice following treatment of L. chagasi (now designated as L. infantum) promastigotes with sub-lethal levels of menadione or H2O2 (Wilson et al., Reference Wilson, Andersen and Britigan1994). In light of the more recent findings that ROS triggers the differentiation of infective amastigotes in L. amazonensis, we hypothesized that the increase in virulence reported by Wilson et al. (Reference Wilson, Andersen and Britigan1994) in L. infantum might be explained by a similar mechanism. To test this hypothesis, we compared the efficacy of the conventional pH/temperature-mediated differentiation protocol to treatments that induce ROS signalling. We found that both H2O2 and menadione at specific concentrations induce the promastigote to amastigote morphological transition, which is marked by loss of the long flagella and reorganization into rounded forms at 24 h following treatment. Expression of amastigote-specific genes, including the gene encoding A2, is also upregulated by exposure to the ROS-generating agents. Thus, our findings provide important mechanistic insight into the previously reported oxidative stress-induced enhancement of L. infantum virulence (Wilson et al., Reference Wilson, Andersen and Britigan1994), and demonstrate that the previously described role of ROS in regulating the development of virulent amastigote forms (Mittra et al., Reference Mittra, Cortez, Haydock, Ramasamy, Myler and Andrews2013) can be applied more broadly to include the visceralizing species L. infantum and L. donovani, which cause very severe forms of human disease.
Materials and methods
Primers
All primers used in this study were designed based on Leishmania species-specific genomic sequences from TriTrypDB (http://tritrypdb.org/tritrypdb/). Primer efficiency was calculated by performing quantitative PCR after serial dilution of the template genomic DNA and calculating the efficiency using the Bio-Rad CFX manager programme. All primers were within an acceptable range for qPCR (90–110% as recommended by the manufacturer). See online Supplementary Table S1 for sequences and primer efficiency.
Parasites
Leishmania infantum (Brazil isolate) or L. donovani (India isolate, MHOM/IN/83/Mongi-142) promastigotes were obtained from Dr David Sacks (NIH) and cultured in vitro at 26 °C in M199 growth media containing 40 mm HEPES, pH 7.4 and supplemented with 20% heat-inactivated fetal bovine serum (FBS), 0.1% hemin (25 mg mL−1 in 50% triethanolamine), 0.1 mm adenine, pH 7.5, 0.0001% vol/vol biotin, 5 mm L-glutamine and 100 U mL−1 penicillin/0.1 mg mL−1 streptomycin. Axenic amastigote differentiation was induced by centrifuging log phase promastigotes (between 1–5 × 107 parasites mL−1) at 2000 × g and resuspending them in amastigote media at 36 °C at an initial concentration of 5 × 106 cells mL−1. Axenic amastigote media consists of M199 media supplemented with 0.25% glucose, 0.5% trypticase, 40 mm Na succinate, 0.00025% hemin, 0.1 mm adenine, 1% L-glutamine and 100 U/mL penicillin/0.1 mg mL−1 streptomycin and 20% FBS at a pH of 5.2.
ROS treatment
Promastigotes in the log phase of growth were seeded in media containing either H2O2 (diluted with sterile water) or menadione (Sigma-Aldrich, St. Louis, MO, USA; diluted with DMSO), at an initial concentration of 5 × 106 cells mL−1. Menadione is a drug that causes accumulation of superoxide in the mitochondria through redox cycling. Cells that are denoted as a ‘no drug’ sample were treated with an equal volume of sterile water or DMSO, respectively. Samples were incubated in a total volume of 2 mL per sample at 26 °C.
Cell viability assays and determination of elongated/flagellated vs. rounded/non-flagellated cells
Cell viability was determined by staining with fluorescein diacetate (FDA) (Sigma-Aldrich, St. Louis, MO, USA), a membrane-permeable molecule that becomes fluorescent after being cleaved intracellularly in metabolically active cells (Sacks and Melby, Reference Sacks, Melby and Coligan2001). FDA was prepared at a stock concentration of 5 mg mL−1 in acetone. This stock was diluted in PBS to get a working concentration of 0.02 mg mL−1. Equal volumes of sample and a working stock of FDA were mixed and incubated for 5 min at room temperature. Ten microlitres of this FDA/cell mixture was loaded into a haemocytometer. Cells that fluoresced green were counted microscopically using 40× (0.75) objective of a Nikon Eclipse 200 microscope, and noted as being elongated/flagellated or rounded/non-flagellated based on the cell morphology and visibility of flagellum.
Fluorescence and scanning electron microscopy
DIC and FDA fluorescence images were acquired with a Nikon Eclipse Ti using a 40× (1.40) objective. After the various treatments, the parasites were washed twice with 1× PBS and resuspended in 100 µL of 1× PBS. An equal volume of FDA (0.02 mg mL−1) was added, followed by imaging.
Scanning electron microscopy (SEM) was performed as previously described (Mittra et al., Reference Mittra, Laranjeira-Silva, Perrone Bezerra de Menezes, Jensen, Michailowsky and Andrews2016). Briefly, 3 × 106 cells were centrifuged at 2000 × g for 5 min. Cells were resuspended in 3 mL of PBS+/+ (supplemented with calcium and magnesium), added to poly-L-lysine-coated coverslips and incubated for 15 min. After two washes with PBS+/+, samples were fixed in 2.5% (v/v) glutaraldehyde in 0.1 M sodium cacodylate buffer, pH 7.4 for 60 min and post-fixed with osmium tetroxide in the same buffer for 1 h at room temperature. After sputter coating with Au/Pd, the preparations were imaged using a variable pressure Hitachi SU3500 SEM.
Iron depletion and supplementation
Iron-depleted promastigote growth media was prepared as described before (Mittra et al., Reference Mittra, Cortez, Haydock, Ramasamy, Myler and Andrews2013). Iron-depleted FBS was generated by adding 10 mm ascorbic acid to 100 mL of FBS for 6 h at 37 °C, until the OD reading at 405 nm was reduced to 0.6–0.8. Following this, 5 g 100 mL−1 Chelex® (Bio-Rad) was added and stirred at 50 rpm for 3–4 h and then filtered through Whatman paper and dialysed (2000D cut-off) against 4 L of cooled, sterile PBS with three buffer changes every 6 h. Iron-depleted FBS was then filter sterilized (0.22 µm filter pore size) and stored at −20 °C. Iron-depleted media was generated in the same manner as culture media, except that iron-depleted FBS was used and hemin (another source of iron) was excluded. The media was stirred with 5 g 100 mL−1 Chelex® for 1 h at room temperature and filter sterilized. Iron supplementation was done by adding Fe-NTA at a concentration of 8 µ m.
Determination of gene expression by qPCR
Equal numbers of cells per biological replicate (1–5 × 107 cells) were spun down at 2000 × g for 5 min. Cells were washed with PBS twice and RNA was isolated using the RNeasy kit (QIAGEN, Hilden, Germany) as per the manufacturer's protocol. Both on-column and post-isolation RNase-free DNase (QIAGEN, Hilden, Germany) digestion were performed to ensure complete removal of any contaminating genomic DNA. Equal amounts of RNA for each biological set were used for cDNA synthesis (Bio-rad iScript). cDNA was diluted to 1 ng µL−1 concentrations for each sample and amplified with gene-specific primers using the Sybr Green master mix, according to the manufacturer's protocol. A CFX96 qPCR instrument (Bio-Rad, Hercules, CA, USA) was used and three technical replicates as well as a no template control were run with each plate. Primer efficiency was calculated (see online Supplementary Table S1) and gene expression was normalized to ubiquitin hydrolase, which is known to be expressed constitutively (Rochette et al., Reference Rochette, Raymond, Ubeda, Smith, Messier, Boisvert, Rigault, Corbeil, Ouellette and Papadopoulou2008; Depledge et al., Reference Depledge, Evans, Ivens, Aziz, Maroof, Kaye and Smith2009).
Results
Iron deprivation generates amastigote-like parasites more effectively than traditional methods
We tried several conditions for differentiating L. infantum amastigotes in axenic culture, all involving shifting of promastigotes in culture (pH 7.4/26 °C) to low pH/high temperature to mimic intra-macrophage conditions that are conducive for amastigote growth and replication (Sereno and Lemesre, Reference Sereno and Lemesre1997; Somanna et al., Reference Somanna, Mundodi and Gedamu2002). All the protocols we tried, which mainly differed in amastigote media composition with small variations in pH or temperature, were not efficient in generating viable amastigotes (data not shown) when viability was estimated using FDA staining assay (Sacks and Melby, Reference Sacks, Melby and Coligan2001). This variability between our results and those reported in the literature could be related to differences in the parasite strains used in different laboratories. Our best results were achieved using an amastigote media composition similar to that used for L. amazonensis (Huynh et al., Reference Huynh, Sacks and Andrews2006), but with 5.5 as final pH and incubation at 36 °C for culture growth. Transfer of log-phase promastigotes to amastigote culture conditions (36 °C/pH 5.5) for 24 h resulted in about 43% decrease in the number of FDA-stained viable cells, when compared with the 0 h time point (Fig. 1A). Microscopic examination showed that about 60% of the FDA-positive cells had lost their long flagella and developed the rounded, amastigote-like morphology (Fig. 1B). On the other hand, cells kept in promastigote growth conditions (26°C/pH 7.4) replicated normally, with about 4-fold increase in viable cell count between 0 and 24 h and showing no change in the flagellation pattern (Fig. 1A and C). Microscopic examination confirmed that L. infantum promastigotes maintained in promastigote culture conditions maintained their elongated, flagellated morphology and divided actively, while a shift to amastigote growth conditions caused cells to round up and lose visible flagella. However, a part of the cell population also showed increased darkening and loss of FDA staining, indicating loss of viability (Fig. 1D). This demonstrates the traditional low pH, high temperature shift method is not effective for generating viable axenic amastigotes of L. infantum, at least for the isolate used in our study.
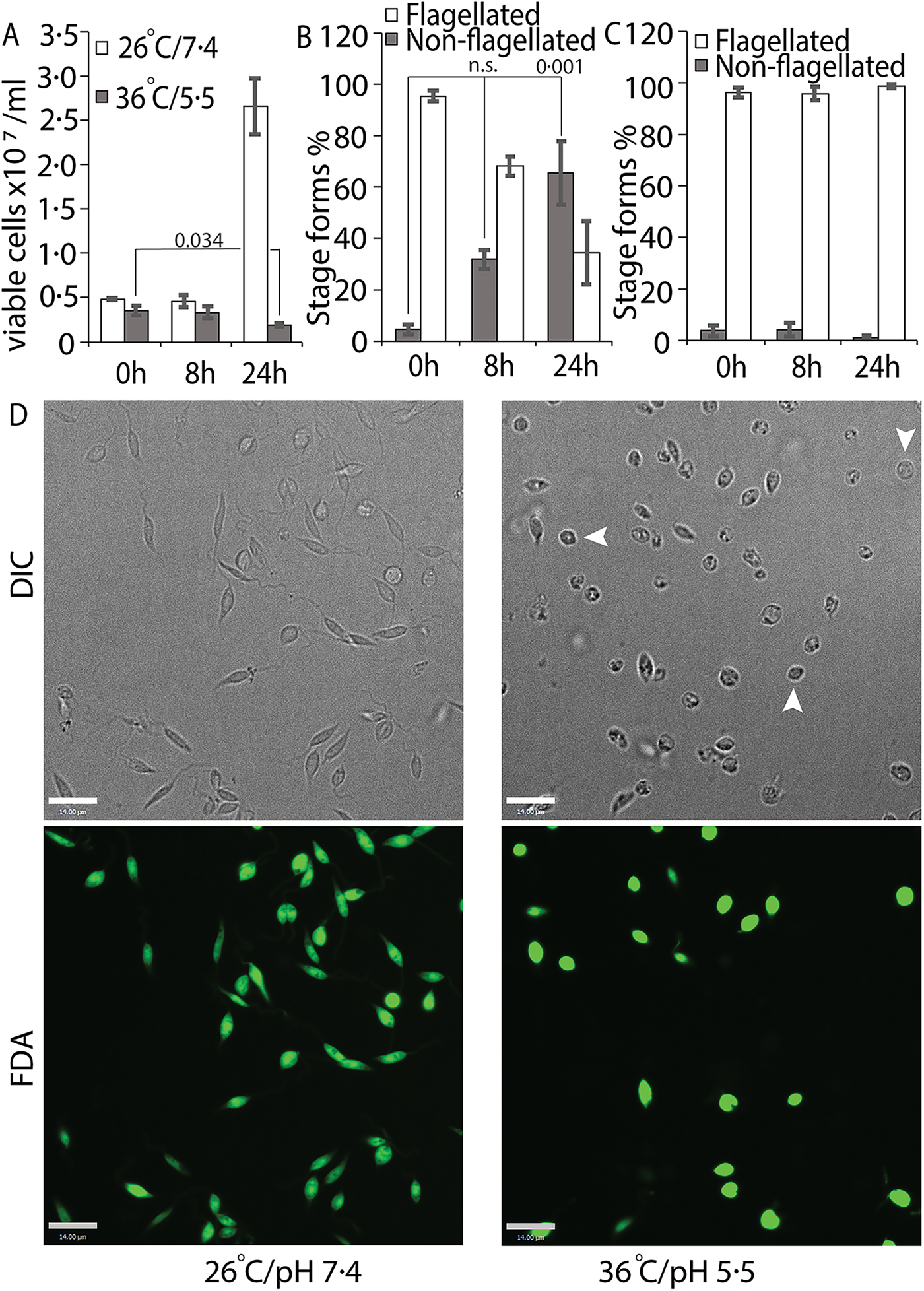
Fig. 1. Low-pH/high-temperature conditions do not efficiently generate L. infantum axenic amastigotes. Mid-log phase promastigotes were resuspended in either promastigote media (26 °C/pH 7.4) or amastigote media at (36 °C/ pH 5.5) at the 0 h time point. (A) Cells were stained with FDA at 0, 8 and 24 h, respectively, and the number of viable cells were enumerated by haemocytometer counting. Student's two-tailed t-test was used. (B) Viable cells in amastigote conditions (36 °C/pH5.5) and (C) viable cells in promastigote medium (26 °C/pH 7.4) were microscopically examined for possession of elongated flagellum. A one-way ANOVA with Dunnett's post-hoc test was performed, with 0 h (in amastigote media) values as controls. (A–C) represent the mean of four independent biological replicates (n = 4). Error bars represent standard error of measurement. P values are indicated in the figure. n.s. indicates changes were not statistically significant. (D) Representative images showing the morphology (DIC) and viability (FDA fluorescence) of parasites in promastigote (26 °C/pH 7.4) and amastigote culture (36 °C/pH 5.5) conditions. Bar = 14 µ m. Viable amastigote-like parasites are indicated by arrows.
We also transferred L. infantum promastigotes from regular culture media to iron-depleted media, and monitored changes in growth rate and cell morphology (loss of elongated flagella in particular) for several days. As previously reported, the transfer of L. amazonensis promastigotes growing in regular promastigote media to iron-depleted media is effective in initiating the amastigote stage differentiation process, through a ROS signalling pathway (Mittra et al., Reference Mittra, Cortez, Haydock, Ramasamy, Myler and Andrews2013, Reference Mittra, Laranjeira-Silva, Perrone Bezerra de Menezes, Jensen, Michailowsky and Andrews2016, Reference Mittra, Laranjeira-Silva, Miguel, Perrone Bezerra de Menezes and Andrews2017). Similarly, transfer to iron-depleted media lowered the growth rate of L. infantum promastigotes (Fig. 2A) and induced the appearance of distinct amastigote-like morphology on day 4, with approximately 70% of the population becoming non-flagellated by day 5 (Fig. 2B and C). Positive staining with the viability dye FDA confirmed that there was no significant loss of viability under these conditions (Fig. 2B). As previously reported for L. amazonensis (Mittra et al., Reference Mittra, Cortez, Haydock, Ramasamy, Myler and Andrews2013), add-back of iron (8 µ m of Fe-NTA) to the iron-depleted media promoted promastigote growth to levels similar to regular media, and reduced the number of rounded/non-flagellated forms on days 4 and 5 of culture. This result strongly suggests that the iron-dependent, ROS-mediated amastigote differentiation signalling pathway previously described for L. amazonensis might also be functional in visceral leishmaniasis species.
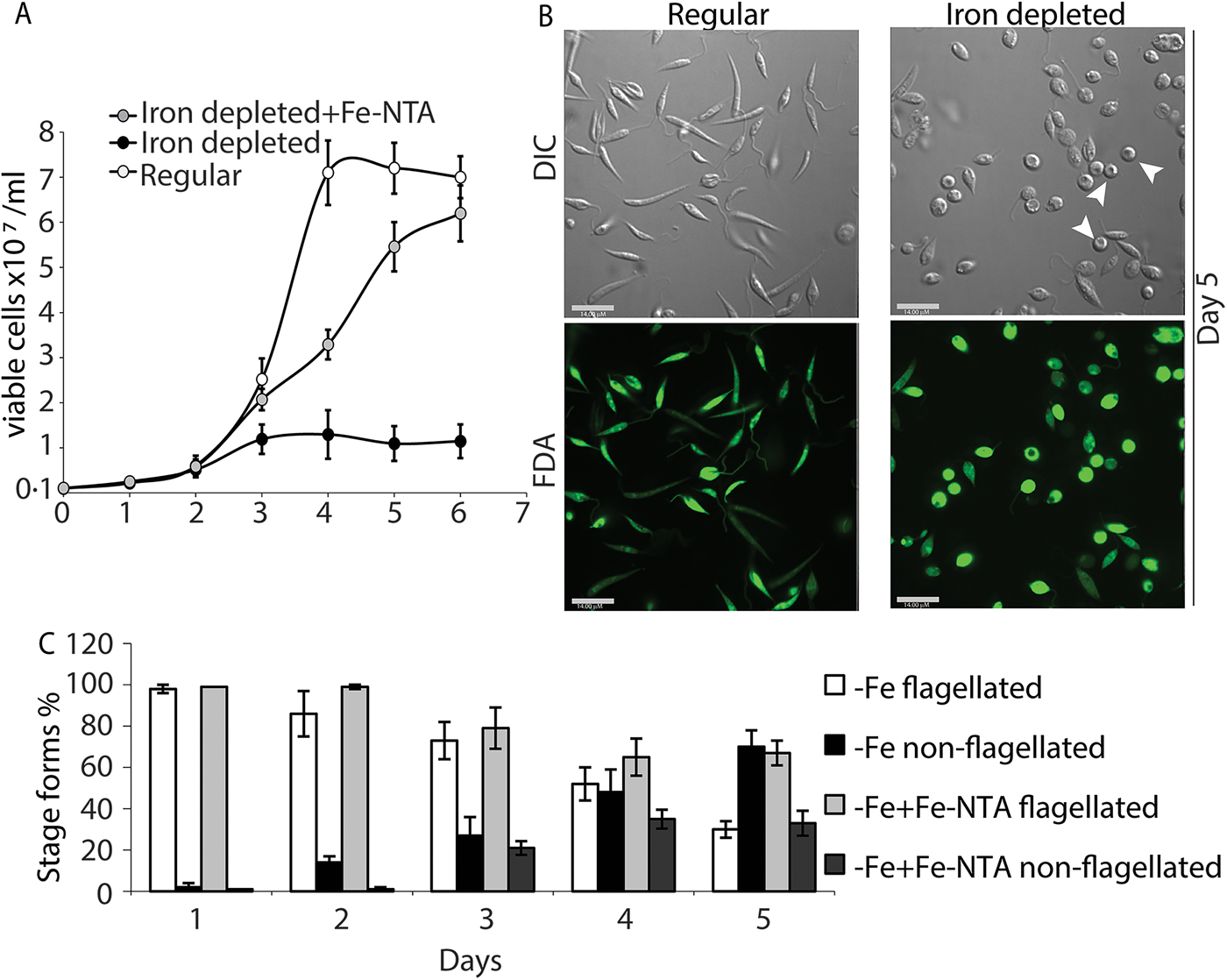
Fig. 2. Iron deprivation triggers differentiation into amastigote-like forms. Promastigotes from log-phase cultures were resuspended in regular medium, iron-depleted medium or iron-depleted medium supplemented with 8 µ m Fe-NTA at 1 × 106 cells mL−1 initial concentration. (A) Numbers of viable cells (stained with FDA) were counted over 6 days. (B) Representative images showing morphology (DIC) and viability (FDA) of parasites in regular or iron-depleted media on the fifth day of culture. Bar = 14 µ m. (C) Graphical representation showing percentage of flagellated and non-flagellated parasite forms in L. infantum cultures under iron-depleted and iron-replete conditions over time. The data represent the mean ± standard error of three independent experiments. Viable amastigote-like parasites are indicated by arrows.
Exposure of L. infantum to ROS causes them to differentiate into an amastigote-like form
To directly test the potential role of ROS signalling in initiating L. infantum amastigote differentiation, we treated log-phase promastigotes cultured in regular iron-containing media (20% FBS) with an incremental concentration gradient of H2O2. Viable cells were counted at the 24 h time point following the treatment, which corresponded with the highest incidence of morphological alterations. The appearance of rounded/non-flagellated forms was less prominent at later time points. As H2O2 is rapidly degraded by catalase present in the FBS component of the parasite growth media, the loss of effectivity after 24 h is not surprising. Based on our previous findings with L. amazonensis, if H2O2 is a signal for promastigote to amastigote differentiation, we expected promastigotes to lose their elongated flagella, assume an amastigote-like rounded morphology and exhibit a decreased doubling time at the optimal H2O2 concentration. We also expected that overtreatment would shift the amastigote differentiation signal to signals for apoptosis or necrosis. Thus, this treatment with a gradient would determine the ideal conditions for morphological change and viability. Consistent with the stated hypothesis, the treatment caused an arrestment of cell division and eventually viability in L. infantum, commensurate with the concentration of H2O2 added (Fig. 3A). As the number of viable cells decreased, the number of non-flagellated cells increased as a function of the concentration of H2O2 (Fig. 3B). Parasites treated with no drug or 100 µ m H2O2 did not exhibit a significant change in morphology – retained their flagella, elongated shape and divided at the normal promastigote rate (12 h doubling time). Doublets of head-to-head joined cells, representing actively dividing Leishmania promastigotes, were visible under both conditions (no drug or 100 µ m H2O2). Based on both viability and morphological change, the optimal concentration of H2O2 to induce L. infantum amastigote differentiation appeared to be 200 µ m. At this concentration, approximately 42% of the parasite population had lost their elongated flagella and rounded up into an amastigote-like form, indicating successful initiation of differentiation, without any loss in viability. The majority of the parasites treated with 250 µ m H2O2 showed a distinct amastigote-like phenotype, but also showed decreased viability (compared with the 0 h time point), with cell-clumping becoming more apparent. Higher concentrations of H2O2 (300 and 500 µ m) resulted in almost 100% cell death at 24 h following treatment (Fig. 3C).
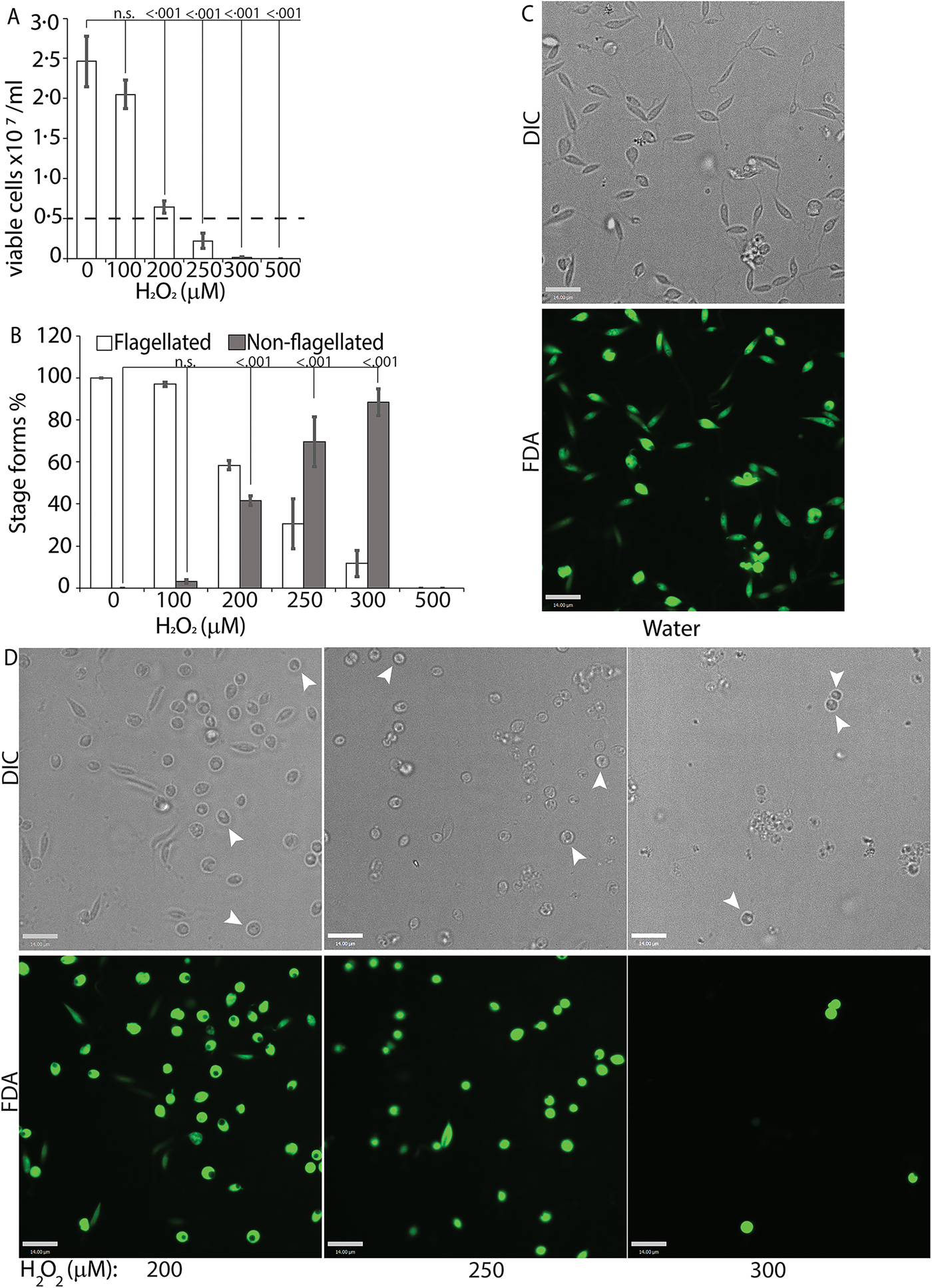
Fig. 3. H2O2 at optimal concentration serves as a signal for differentiation. Mid-log phase WT promastigotes resuspended at a concentration of 5 × 106 cells mL−1 in regular medium were treated with water (0) or 100, 200, 300, 400 and 500 µ m H2O2, respectively. (A) Cells were stained with FDA and viability was assessed by counting live cells with a haemocytometer. Starting cell concentration (5 × 106 cells mL−1) is indicated by the broken line. A one-way ANOVA with Dunnett's post-hoc test was performed, with 24 h untreated as control. (B) Live cells with and without a flagellum were enumerated. A one-way ANOVA with Dunnett's post-hoc test was performed, with 24 h untreated values as the control. Results represent the mean of four independent biological replicates (n = 4). Error bars represent standard error of measurement. P values are indicated in the figure. (C) and (D) Representative images showing the morphology (DIC) and viability (FDA fluorescence) of parasites treated with water or increasing concentrations of H2O2. Bar = 14 µ m. Viable amastigote-like parasites are indicated by arrows.
While H2O2 treatments represented an exogenous source of ROS signal, we also treated L. infantum promastigotes with varying concentrations of menadione, a mitochondrial superoxide-generating drug, to mimic metabolic mitochondrial ROS signals generated through the electron transport chain (ETC) activity (Loor et al., Reference Loor, Kondapalli, Schriewer, Chandel, Vanden Hoek and Schumacker2010). Menadione, a redox cycler, induces superoxide production by transferring electrons from the ETC to available oxygen molecules, which are then converted to H2O2 by the mitochondrial SODA (for Leishmania spp.). Log-phase L. infantum promastigotes cultured in regular media (20% FBS) were treated with a gradient of menadione concentrations, and cell viability was determined using FDA staining at 24 h after treatment. Treatment of promastigotes with DMSO alone (no drug), or 1 µm of menadione did not cause any marked change in viability or morphology (Fig. 4A and B), as the cells remained elongated and divided actively. The optimal condition was achieved at a menadione concentration between 3 and 5 µ m. Starting with 3 µ m menadione, parasite growth rate was slowed down and around 21% of the cells lost the elongated flagella and exhibited amastigote-like morphology. At 5 µ m menadione concentration, there was a complete cessation of cell division, with >80% of the parasites losing their elongated flagella and rounding up. No significant loss in viability (as compared with the 0 h time point) was detected under these conditions, even though low amounts of cell clumping was present in some of the replicate samples. Parasite populations treated with 7.5 and 10 µ m of menadione showed complete loss of elongated flagella, but cell clumping became more apparent (Fig. 4C). These data clearly demonstrate the effectivity of ROS-generating treatments in initiating L. infantum amastigote differentiation, and is in accordance with our previous reports of menadione-induced promastigote to amastigote differentiation in L. amazonensis (Mittra et al., Reference Mittra, Cortez, Haydock, Ramasamy, Myler and Andrews2013, Reference Mittra, Laranjeira-Silva, Perrone Bezerra de Menezes, Jensen, Michailowsky and Andrews2016, Reference Mittra, Laranjeira-Silva, Miguel, Perrone Bezerra de Menezes and Andrews2017).

Fig. 4. Menadione-generated mitochondrial ROS induces differentiation. Mid-log phase WT promastigotes were resuspended at a concentration of 5 × 106 cells mL−1 in regular medium and treated with DMSO (0), or, 1, 3, 5, 7.5 and 10 µ m menadione, respectively, for 24 h. (A) Cells were stained with FDA and live cells were counted with a haemocytometer. Starting cell concentration (5 × 106 cells mL−1) is indicated by the broken line. A one-way ANOVA with Dunnett's post-hoc test was performed, with 24 h untreated values as the control. (B) Live cells with and without a flagellum were enumerated. A one-way ANOVA with Dunnett's post-hoc test was performed, with 24 h untreated as the control column. (A–B) Our results represent the mean of three independent biological replicates (n = 3). Error bars represent standard error of measurement. P values are indicated in the figure. (C) and (D) Representative images showing the morphology (DIC) and viability (FDA fluorescence) of parasites treated with DMSO or with increasing concentrations of menadione. Bars = 14 µm. Viable amastigote-like parasites are indicated by arrows.
SEM was used to confirm that the morphological changes observed under DIC microscopy were consistent with that of an amastigote form. Leishmania infantum was treated with either agent, 200 µ m H2O2 or 5 µ m menadione. As expected, untreated cells remained elongated and flagellated, while parasites exposed to either H2O2 or menadione experienced a loss of long flagella, cell rounding and a decrease in size, all hallmarks of the amastigote form (Fig. 5).
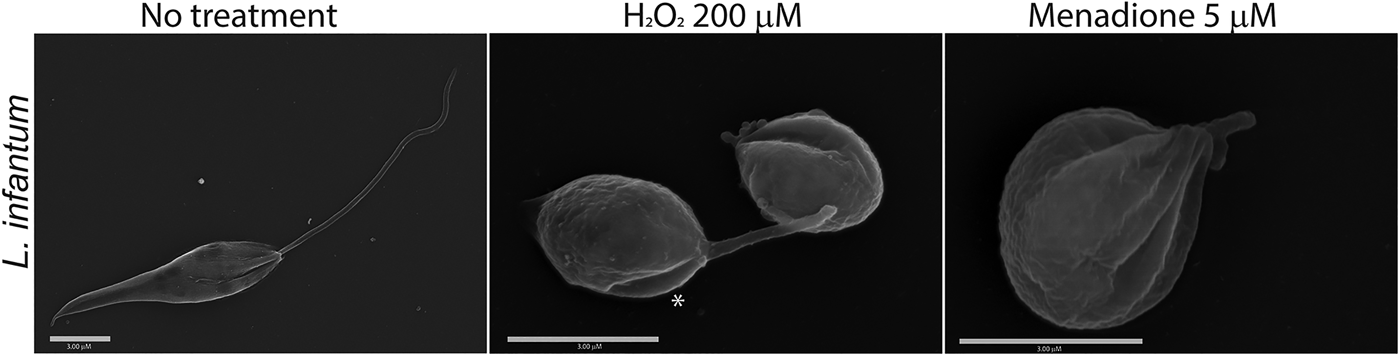
Fig. 5. H2O2 and menadione induce amastigote-like morphological changes in L. infantum. Scanning electron micrographs showing L. infantum promastigotes in regular medium that were subjected to treatment with no drug, 200 µ m H2O2 or 5 µ m menadione. Bars = 3 µm. The asterisk indicates parasites in the process of differentiation.
Amastigote stage-specific gene transcripts are upregulated following ROS treatment or shift to low-pH/high-temperature conditions
To confirm if ROS-induced amastigote-like morphological transition of L. infantum promastigotes is orchestrated through genetic controls, we compared changes in expression levels of several stage-specific transcripts at 24 h following exposure to H2O2 or menadione by quantitative RT-PCR (qRT-PCR). Steady-state transcript levels for A2, a protein exclusively expressed during the amastigote stage of visceral Leishmania strains like L. infantum, but barely detectable in promastigote form (Zhang et al., Reference Zhang, Charest, Ghedin and Matlashewski1996; Zhang and Matlashewski, Reference Zhang and Matlashewski1997) were compared between untreated cells and cells treated with either 200 µ m H2O2 (Fig. 6A) or 3 µ m menadione (Fig. 6B), as parasite health and viability was uncompromised at these concentrations. Upregulation of A2 transcript levels was detected in H2O2 and menadione-treated cells (>3- and >2-folds, respectively). Orthologues of AML1 and AML2, the ‘amastin-like’ genes known to be upregulated during amastigote differentiation (Lahav et al., Reference Lahav, Sivam, Volpin, Ronen, Tsigankov, Green, Holland, Kuzyk, Borchers, Zilberstein and Myler2011; Mittra et al., Reference Mittra, Cortez, Haydock, Ramasamy, Myler and Andrews2013), also showed a relative increase in transcript levels but was not statistically significant. No noticeable change in transcript levels for ATG8 was noted. Notably, an increased transcript level for this autophagy gene was observed in L. amazonensis under low iron conditions and following menadione treatment, but not with H2O2 (Mittra et al., Reference Mittra, Cortez, Haydock, Ramasamy, Myler and Andrews2013). Although we noticed a trend in gene expression changes similar to what occurs during differentiation of the amastigote stage, the changes were not significant in the samples treated with 3 µ m menadione. This is likely due to the fact that this lower drug concentration was not sufficient to initiate differentiation. However, when L. infantum promastigotes were treated with a higher concentration of menadione (10 µm), all amastigote stage-specific genes tested showed considerable upregulation (Fig. 7) with the exception of AML1. As expected, transcript levels for the para-flagellar rod protein (Pr2C) were downregulated, consistent with the loss of the elongated flagella typical of promastigotes.

Fig. 6. Both ROS and low-pH/high-temperature-induced differentiation result in upregulated amastigote-specific genes but differ in p27 dependence. Mid-log phase L. infantum promastigotes were resuspended at a concentration of 5 × 106 cells mL−1 in regular medium and treated with either 200 µ m H2O2 (A) or 3 µ m menadione (B) for 24 h, or resuspended in amastigote media at 36 °C for 8 h (C). Changes in expression levels of genes associated with amastigote differentiation were determined by qRT-PCR using RNA isolated from cells collected at the indicated time points. Fold changes were expressed relative to water as control for H2O2-treated cells, (A) DMSO as control for menadione-treated cells and (B) cells in promastigote media (26 °C/pH7.4) as control for transfer to amastigote media (pH 5.5) at 36 °C (C). (D) Determination of changes in expression levels of mitochondrial COX-associated gene p27. Results are representative of four biological replicates for H2O2 treatment, three biological replicates for menadione treatment and shift to amastigote conditions, respectively. Error bars represent standard error of measurement. Student's two-tailed t-test was used to assess significance. P values are indicated in figure.
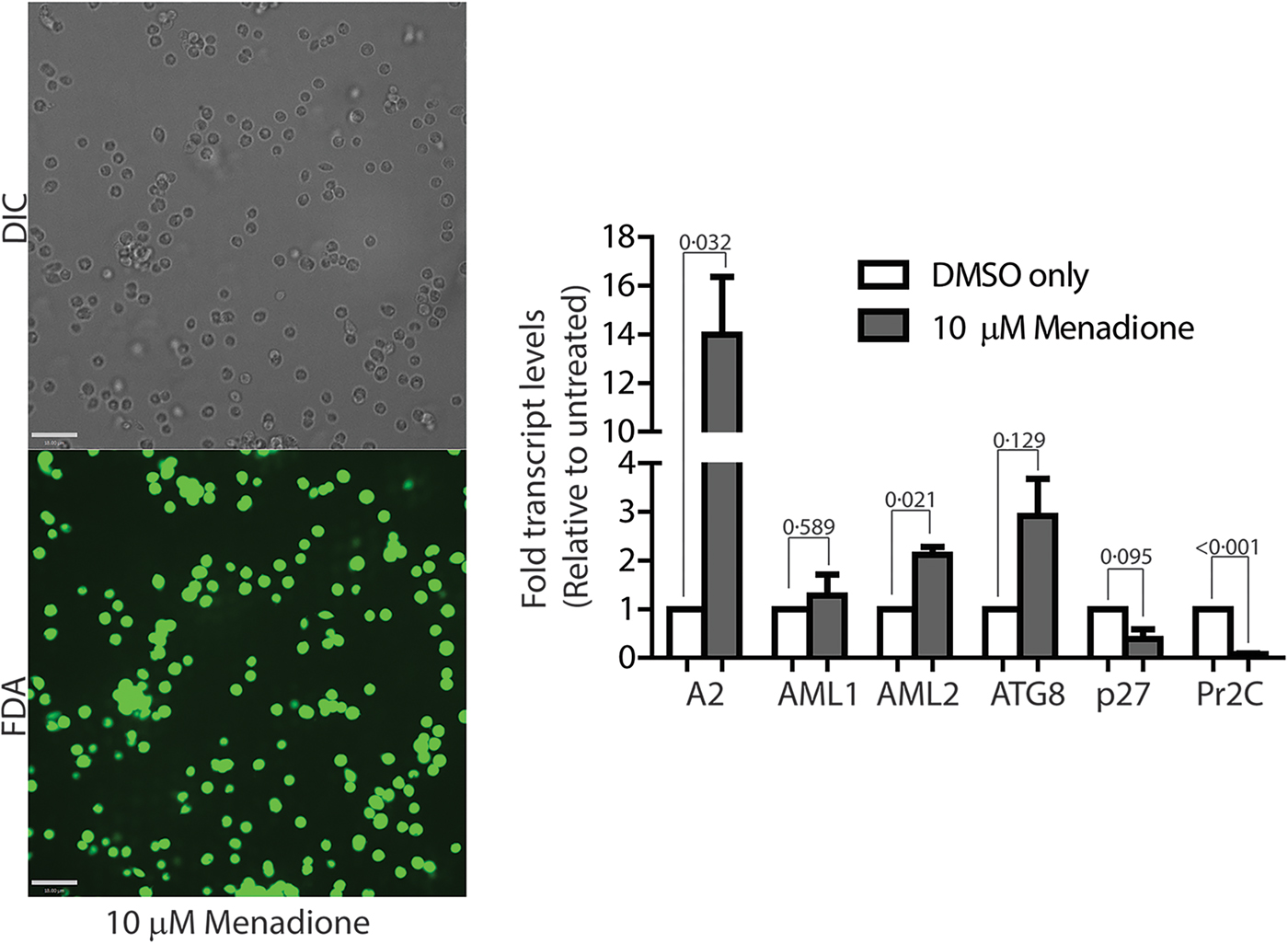
Fig. 7. Leishmania infantum parasites treated with 10 µm menadione fully differentiate into amastigotes. DIC microscopy and FDA staining confirms that parasites treated with 10 µ m menadione are fully differentiated, with some cell clumping. All amastigote markers, with the noted exception of p27, are upregulated and the promastigote marker, Pr2C, is downregulated. Experiments consist of three biological replicates assayed in triplicate. Error bars represent standard error measurement. Bar = 14 µm. Student's two-tailed t-test was used to assess significance. P values are indicated in figure.
Comparative analysis of transcript levels using L. infantum promastigotes induced to differentiate under low-pH/elevated temperature conditions showed a similar trend for A2, AML1, AML2 and ATG8 upregulation, while Pr2C was downregulated (Fig. 6C), even though RNA samples were isolated from cell samples collected at a much earlier time point (8 h, compared with 24 h). Analysis of 24 h samples was avoided because of the reduced viability at that time. A very interesting difference in the gene expression pattern induced by ROS exposure, compared with the conventional low pH/high temperature shift method for amastigote differentiation, was observed for transcript levels of the virulence-determining protein p27, which is an essential component of the active cytochrome c oxidase (COX) at the amastigote stage. Expression of p27 increases the overall ETC activity and promotes oxidative phosphorylation for ATP production, as the parasites shift towards a mitochondria-dependent mode of energy generation (Dey et al., Reference Dey, Meneses, Salotra, Kamhawi, Nakhasi and Duncan2010). A 6-fold upregulation of p27 expression was observed with the low-pH/high-temperature shift, whereas treatment with either 200 µm H2O2 or 10 µm menadione resulted in downregulation (Figs 6D and 7). This result suggests that while p27-mediated enhancement of ETC activity is necessary for generating superoxide during low-pH/high-temperature exposure, but its expression is not necessary and can be bypassed during treatment with the superoxide-inducing drug menadione or direct exposure to H2O2.
Leishmania donovani also differentiates into amastigotes in response to ROS treatment
To further validate our findings that ROS exposure can trigger amastigote differentiation and virulence in visceralizing Leishmania species, we tested L. donovani, the causative agent of the highly lethal kala-azar. Leishmania donovani promastigotes in culture (pH7.4; 26 °C temperature) were treated with either 200 µ m H2O2 or 5 µ m menadione, exactly as described earlier. Both H2O2 and menadione treatments resulted in attenuation of cell division (compare 24 h carrier treatments as control to 24 h H2O2 or menadione-treated samples) but did not affect overall viability of the parasites in culture (compare 0 h carrier treatments as control with 24 h H2O2 or menadione-treated samples), was confirmed by FDA staining (Fig. 8A, B and E). A significant increase in the number of non-flagellated cells, consistent with promastigote to amastigote transition, was also observed in promastigote cultures 24 h following H2O2 or menadione treatment (Fig. 8C–E), similar to that seen with L. infantum. SEM examination of parasites clearly showed loss of the elongated flagellar structure, reduction in body length and appearance of amastigote-like morphology following both ROS treatments (Fig. 8F). Collectively, our results identify ROS-mediated signalling as a critical step for differentiation and virulence development in visceralizing Leishmania species.
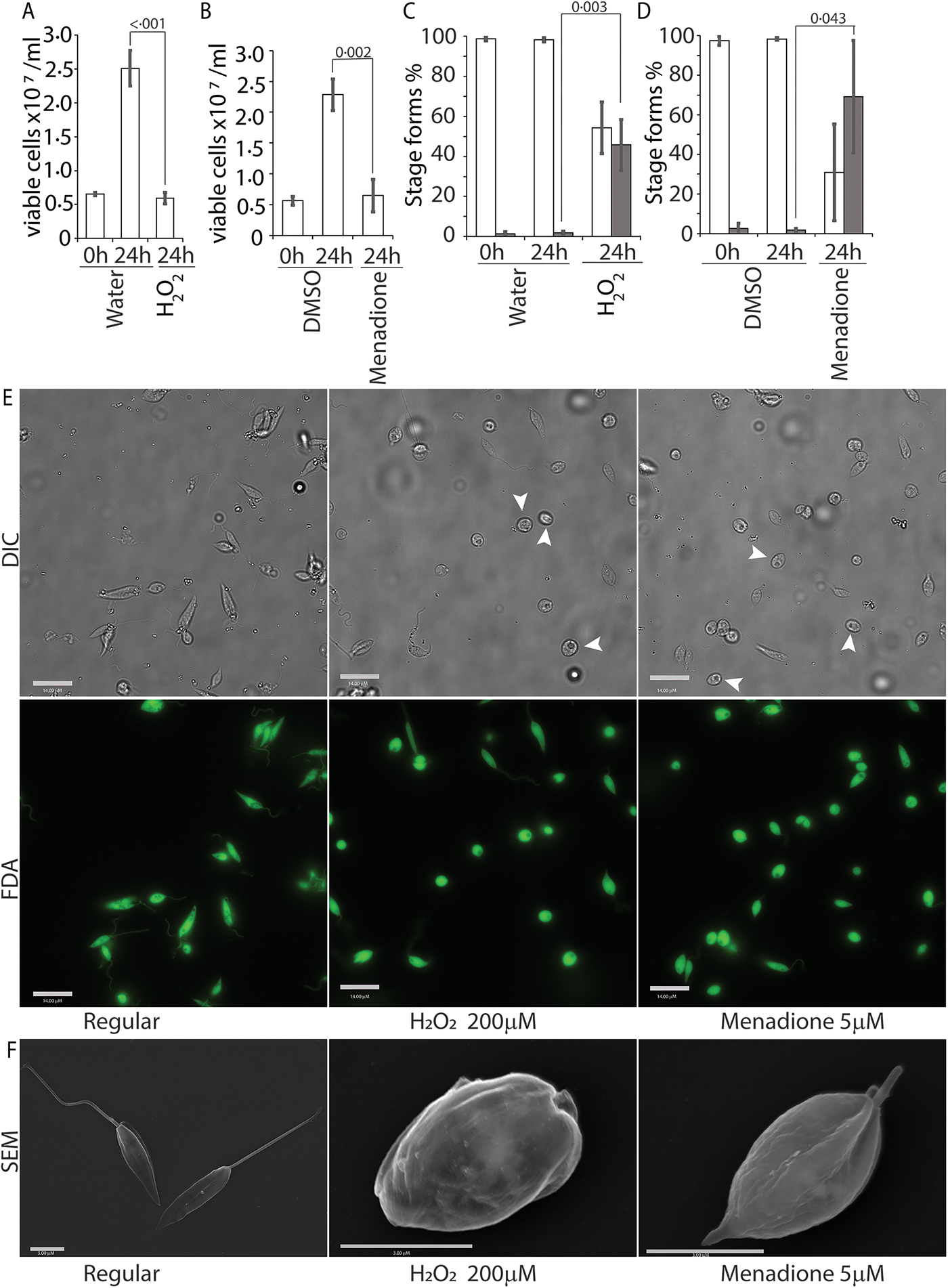
Fig. 8. ROS triggers amastigote differentiation in L. donovani. Mid-log-phase L. donovani promastigotes were resuspended at a concentration of 5 × 106 cells mL−1 and treated with either just the carrier (water or DMSO), 200 µ m H2O2 or 5 µ m menadione. Figures (A) and (B) represent live cell counts determined with the FDA assay using a haemocytometer for H2O2 and menadione treatments, respectively. Figures (C) and (D) represent an enumeration of flagellated (white columns) and non-flagellated cells (dark columns) for H2O2 and menadione treatments. Data in A–D represent the mean of three independent biological replicates (n = 3). Error bars represent the standard error of measurement. Student's two-tailed t-test was used in all panels. P values are indicated in the figure. (E) Representative images showing the morphology (DIC) and viability (FDA fluorescence) of parasites in regular promastigote culture and cultures treated with 200 µ m H2O2 or 5 µ m menadione. Bar = 14 µ m. Viable amastigote-like parasites are indicated by arrows. (F) Scanning electron micrographs depicting morphology of representative cells in regular media or treated with H2O2 or menadione. Bar = 3 µ m.
Discussion
There has long been evidence for an interplay between ROS and Leishmania infections. On their initial encounter with host macrophages, invading pathogens can be exposed to a macrophage-mediated ROS burst that acts as a first line of innate immune defence. Leishmania, however, has developed a unique ‘Trojan-horse’ mechanism for muting this ROS burst, gaining entry inside and colonize macrophages (Murray, Reference Murray1981). Successful invasion depends on the ability of invading parasites to upregulate their anti-oxidant defence system – as evidenced by increased expression of SODA and peroxidases during infective metacyclic promastigote and subsequent amastigote stages. Priming of the anti-oxidative system primarily occurs via increased metabolic oxidative stress and is considered as key for virulence development (Alzate et al., Reference Alzate, Arias, Moreno-Mateos, Alvarez-Barrientos and Jimenez-Ruiz2007). Notably, a role for subtle changes in ROS levels in controlling cell fate has been demonstrated, particularly for the H2O2-induced triggering of promastigote to amastigote differentiation (Mittra et al., Reference Mittra, Laranjeira-Silva, Miguel, Perrone Bezerra de Menezes and Andrews2017). The molecular targets of H2O2 and other details of this signalling cascade remain to be elucidated, but the effect of ROS on integral differentiation processes such as autophagy is well established (Williams et al., Reference Williams, Woods, Juliano, Mottram and Coombs2009; Mittra et al., Reference Mittra, Cortez, Haydock, Ramasamy, Myler and Andrews2013).
A role of mitochondria-generated superoxide and H2O2 in determining virulence have also been demonstrated in L. amazonensis (Mittra et al., Reference Mittra, Cortez, Haydock, Ramasamy, Myler and Andrews2013, Reference Mittra, Laranjeira-Silva, Perrone Bezerra de Menezes, Jensen, Michailowsky and Andrews2016, Reference Mittra, Laranjeira-Silva, Miguel, Perrone Bezerra de Menezes and Andrews2017). Superoxide production occurs through mitochondrial ETC activity and is enhanced under stress. Thus, the available evidence indicates that the ability of L. amazonensis to regulate the surge in superoxide levels, through enzymatic conversion into H2O2 enables differentiation of promastigotes into amastigotes in axenic culture. Previous studies also showed that conditions that induce mitochondrial stress and ROS production in promastigotes, namely iron depletion or transfer to low pH/elevated temperature, also efficiently generate axenic amastigotes in L. amazonensis. Direct implication of ROS in such stress-related differentiation mechanism comes from direct exposure to H2O2 or menadione, which also promoted amastigote differentiation and virulence development in those studies. Here we showed that when subjected to similar conditions of stress including iron depletion, L. infantum and L. donovani show upregulation of amastigote stage-specific transcripts, downregulation of promastigote-specific transcripts and a clear transition into the amastigote morphology, thereby confirming that ROS-mediated regulation of virulence is also functional in visceralizing Leishmania strains.
Importantly, our findings also provide a mechanistic explanation for earlier reports of enhanced infectivity of L. infantum (at the time referred to as L. chagasi) promastigotes following treatment with ROS inducers. Parasites treated with H2O2 or menadione showed a dose-dependent increase in infectivity, compared with the DMSO-treated control, in assays quantifying the parasite load in the liver and spleen of mice (Wilson et al., Reference Wilson, Andersen and Britigan1994). Pre-exposure of the parasites to a sub-lethal oxidative stress resulted in increased viability and resistance to exposure to increasing concentrations of H2O2. However, no promastigote to amastigote morphological change associated with differentiation was reported under their experimental conditions. Interestingly, upregulation of the heat-shock protein hsp70 expression observed in that study suggested a possible link between traditional differentiation methods (heat shock) and ROS accumulation, a suggestion later confirmed by reports of mitochondrial hyper-polarization and associated increase in superoxide levels during heat shock of L. infantum promastigotes (Alzate et al., Reference Alzate, Alvarez-Barrientos, Gonzalez and Jimenez-Ruiz2006, Reference Alzate, Arias, Moreno-Mateos, Alvarez-Barrientos and Jimenez-Ruiz2007). Our results provide further evidence for roles of both ROS and heat shock in L. infantum differentiation. By carefully monitoring cell morphology and stage-specific gene expression during exposure to incremental concentrations of H2O2 and menadione, we have established that at optimal concentrations, both treatments are very effective in inducing promastigote to amastigote differentiation without a loss in viability. Our failure in repeated attempts to generate viable axenic amastigotes using the low pH/elevated temperature method described in earlier reports could possibly be ascribed to strain differences, which might respond differently to the variable pH and temperature conditions used in those studies (Sereno and Lemesre, Reference Sereno and Lemesre1997; Somanna et al., Reference Somanna, Mundodi and Gedamu2002). On the other hand, we did observe a morphological change and upregulation of amastigote-associated gene expression in L. infantum after 8 h of pH/temperature shift, consistent with initiation of the differentiation process. However, the marked loss in viability we observed under these conditions, which may have been overlooked in previous studies, might have resulted from oxidative damage, if the levels of ROS triggered by the pH/temperature stress were high enough to overwhelm the parasite's anti-oxidative machinery.
An additional finding that emerged from this study is the inverse trend in p27 expression observed between pH/temperature-mediated and ROS-induced differentiation. This observation reveals a difference between the two processes in their dependence on mitochondrial ETC for the generation of superoxide radicals. While p27-mediated upregulation of COX activity is necessary for enhancing ETC function, to meet the increasing demand for energy production in metacyclic promastigote and amastigote stages (Dey et al., Reference Dey, Meneses, Salotra, Kamhawi, Nakhasi and Duncan2010; Saunders et al., Reference Saunders, Ng, Kloehn, Chambers, Ng and McConville2014), it also serves as a potent source of mitochondrial superoxide that is critical for initiating the ROS signalling cascade. Direct treatment of promastigotes with H2O2 or the superoxide generating drug menadione, on the other hand, bypasses that requirement. In fact, the downregulation of p27 expression observed with higher concentrations of menadione may represent a regulatory mechanism to control ROS levels, by slowing down ETC activity.
Taken together, our findings significantly expand the importance of the H2O2 signalling pathway in promoting Leishmania virulence, by demonstrating the effectiveness of this pathway for triggering amastigote differentiation in two clinically important visceralizing species. Our study also outlines a simple and effective method for generating axenic amastigotes in these Leishmania species without the need for stressors such as pH and temperature changes, and opens up the possibility for exploring anti-oxidants as anti-leishmanial therapeutic agents.
Supplementary material
The supplementary material for this article can be found at https://doi.org/10.1017/pao.2018.15.
Acknowledgements
We would like to thank Jason Hauzel and Amrita Sarkar for their expert assistance in carrying out experiments.
Financial support
This work was supported by the National Institutes of Health grant R01 AI067979 to NWA. YK was supported by an undergraduate research grant from the Howard Hughes Medical Institute to the University of Maryland.
Conflict of interest
None.
Ethical standards
Not applicable.