Introduction
Less cost-prohibitive wearable technologies have made once rarefied digital possibilities for movement and computing (MOCO), especially arts-based practice and inquiry, widely accessible. In this article, we describe our work with musical and movement-responsive networked wearable sensors whose collective sonic response we design together with epistemically diverse participants to influence group improvisation dynamics. An early precedent is the artistic collaboration of John Cage and Merce Cunningham in Variations V, a piece in which photocells and the capacitive response of radio antennas are used to turn a stage into a collectively playable, continuously responsive instrument (Nyman, Reference Nyman1999). Networked wearable computing invigorates the possibilities for creating less spatially bounded and less individualistic, collaborative, room-scale digital musical instruments (DMIs). We explore this potential by utilizing statistical aggregates of orientation and movement across multiple wearable sensors for parameter mapping sonification and auditory augmented feedback. This work is broadly motivated by improvisatory and relational movement practices and technology-augmented dance (e.g., Aylward et al., Reference Aylward, Lovell and Paradiso2006; Naccarato and MacCallum, Reference Naccarato and MacCallum2016; Himberg et al., Reference Himberg, Laroche, Bigé, Buchkowski and Bachrach2018).
Through a hybrid of bottom-up participatory design, sonic augmentation of movement using wearable computing, and experiential research in group dynamics, we creatively explore how configurations of movement-responsive auditory feedback affect a sense of togetherness in ensembles. Participatory design is a well-known design approach that self-consciously engages with the milieu of industrial democracy. By involving future users of a technology with shaping design processes in beneficial ways, while also addressing ethical and political implications, participatory design magnifies questions of democracy and power in workplaces (Ehn, Reference Ehn, Schuler and Namioka1993). Similarly, our investigation of epistemically diverse collective creativity in educational environments moves toward symmetrizing power relations, potentiating more equitable and novel outcomes. A characteristic feature is the lack of well-articulated problems, since these only become concrete during the actual experiments. We arrive, in other words, in a multifaceted space with wearable instruments, a diverse set of actors, and an inchoate set of ideas and intuitions about what we want to achieve, but without a blueprint for how to proceed (Figure 1). The way forward, here, is a process of “co-design,” a term that invokes a design situation in which problems and solutions co-evolve through collaborative effort (Steen, Reference Steen2013). In such situations, where not even the problems are well-defined, abductive reasoning is employed, advancing by iteratively framing problems and potential solutions (Steen, Reference Steen2013). A technocritical dimension of our work, likewise, is to foster a participatory technoculture in which actors adapt wearable technologies through process-based “embodied sensemaking”—first-person experiences activating and enlivening the abductive process (Hummels and Van Dijk, Reference Hummels and Van Dijk2015). By contrast, a less critical and less embodied approach would tend to veil rather than expose the normative biases designed into wearables, to wit, measurement of productive but not “joyful” activity (Spiel et al., Reference Spiel, Kayali, Horvath, Penkler, Harrer, Sicart and Hammer2018). Technical objects do not just form a system of utility, but also generate sense and meaning as objects of culture. Thus, insofar as we combine academic research and artistic practice, our collective inquiry constitutes a “research-creation” event, an experimental approach that catalyzes the generation of new experiences and evolution of collective expression through diverse practices. As dancer and theorist Erin Manning notes, the knowledge this generates may be “extra-linguistic” and/or escape normative modes of inquiry (Manning, Reference Manning2016). Nevertheless, we will do our best to articulate this knowledge to the reader by recapitulating our process concretely, in order to remain critically attentive to those “material complexities of participation” that resist the pretense of a de facto participatory situation wherever an assemblage of diverse actors, epistemes, and techniques is brought together (Tanaka and Parkinson, Reference Tanaka, Parkinson and Emmerson2018). Bottom-up attention to process, in other words, acknowledges that participation is a contingent outcome whose success hinges on felicitous adaptation, exchange, and learning. There is no procedure to guarantee that success.

Figure 1. Top left: dancers instrumented with headband sensors. Bottom left: a non-instrumented dancer introducing a novel movement motif. Center: realization of a concise score with collectively playable wearable digital musical instruments (DMIs). Top right: second author (dancer and choreographer) in discussion with participants. Bottom right: she consults with the first author (managing sound design). Related videos can be found in an online Vimeo showcase (https://vimeo.com/showcase/8623644) and are individually referenced and linked throughout this article. (This collection is also permanently available at https://doi.org/10.5281/zenodo.5762453.)
Our use of the term “assemblage” derives from Deleuze and Guattari, frequently cited philosophers in qualitative research methodologies (e.g., Barrett and Bolt, Reference Barrett and Bolt2007; Koro-Ljungberg, Reference Koro-Ljungberg2015). Accordingly, an assemblage is a multiplicity of elements without a template, center, or hierarchy. Assemblages are never fixed; they continuously vary and evolve by making new connections (Deleuze and Guattari, Reference Deleuze and Guattari1987). In this article, we attempt to flesh out the concrete, fine, artistic and technical decisions that both shape, and are shaped by, these connections in our explorations of collective sonic interaction with wearables. We discuss our participatory approaches and distill principles for communities interested in this transdisciplinary research-creation. The two settings we recount are a series of four collocated workshops for dancers and a distance learning course for undergraduate students who explore embodied telematic togetherness using musical wearables. Given our collaboration with expert dancers—and the adept movement skills and embodied practices that they bring to the events, which includes the second author—we can further specify this work as a form “somatic connoisseurship” (Schiphorst, Reference Schiphorst2011). The rich movement histories and expertise of the dancers suffuse the workshops with tacit embodied knowledge. A rather different goal manifests in the wearable music class, which is to increase the corporeal engagement of nonspecialist students involved in distance learning by having them explore movement-driven sound, diminishing reliance on screen-based interactions. In both scenarios, we follow a pragmatic process-based approach attentive to phenomena emerging during these experiments, which reflects our commitment to “practice-situated” inquiry (Shotter, Reference Shotter, Simon and Chard2014). We address this approach in the following section before proceeding to descriptions of our experiences in the workshops and distance learning course. This paper extends a previous conference paper (Thorn et al., Reference Thorn, Willcox and Sha2020) on the wearable music workshops by introducing many new reflections and details. It also extends a conference paper published by the first author (Thorn, Reference Thorn2021b) on the telematic wearable music class by adding new details, distilling specific design principles, and drawing connections between the development of that course and the knowledge and techniques it inherited from the dance workshops.
Relational Experiential Inquiry in Movement Arts
Practice-Situated Inquiry
Practice-situated “systemic” inquiry begins in the turbulent flow of ongoing activity, without ideally prepared conditions, grounding definitions, strong theories, or firm predictions (Shotter, Reference Shotter, Simon and Chard2014). One does not cease to plan and strategize, but structures must be fluid enough to respond to unforeseen (im-pro-vised) possibilities, which may be highly significant and show up by chance or intuition. Within the complex entanglement of bodies and worlds, practice-situated approaches bracket hard distinctions between subjects and objects, leaving these as outcomes rather than presuppositions of experiments (James, Reference James1912). Attention is focused on the horizon of relation rather than vertical theory (theoria: “to behold”). In collective dance improvisation (CDI), leading and following are dynamical such that, in successful conditions, dancers describe synchronous continuity of moving and being moved (Sheets-Johnstone, Reference Sheets-Johnstone2011). “As we move together, the boundaries between moving and being moved blur” (Himberg et al., Reference Himberg, Laroche, Bigé, Buchkowski and Bachrach2018). In taking this phenomenological description at its word, which is out of keeping with linear scientific-grammatical categories of cause/effect and subject/object, practice-situated approaches become attuned to the emergence of novel experiences. This style of inquiry remains receptive to contingencies and unexpected detours, those forces “beyond” the experiments—tight boundaries around which we do not willfully design or practice, as when an observer of an ensemble event suddenly leaps in to resolve a perceived tension in CDI. This ethos is prominent in experiential and situated third wave HCI, which loosens up formerly strict designer-user dichotomies (Williams and Irani, Reference Williams and Irani2010).
Intentionality in Relational Time-based Arts
Dance and music are time-based performative artistic traditions with a long historical affinity. Both are embodied, participatory, and relational practices. In the case of music:
[Music] is not a unidirectional process, with participants entraining to a particular individual who is the time-keeper; it is likely to involve a process of continuous reciprocal adaptation of the periods and phases of the sounds and actions produced… with each participant continually switching between leading and following each other. (Cross, Reference Cross2014).
Contact improvisation, likewise, which is a style of dance that grew out of the zeitgeist of the 1960s, is a dramatic and rich relational practice:
Contact improvisation is most frequently performed as a duet, in silence, with dancers supporting each other’s weight while in motion. Unlike wrestlers, who exert their strength to control a partner, contact improvisers use momentum to move in concert with a partner’s weight, rolling, suspending, lurching together. They often yield rather than resist, using their arms to assist and support but seldom to manipulate. Interest lies in the ongoing flow of energy… (Novack, Reference Novack1990)
In contact improvisation, the rolling point between bodies cannot be reduced to an aggregate of partners’ intentions. The cognitivist overtones of the word “intention,” suggesting reflexive mental planning prior to initiating an action, produce an unremarkable description of the ongoing flow in CDI, music, and other collective improvisatory practices (Angelino, Reference Angelino2018; Angelino, Reference Angelino2020). Noncognitivist formulae for embodied intentionality, such as “directing-itself-towards” (Heidegger, Reference Heidegger1985) or “motor intentionality” (Merleau-Ponty, Reference Merleau-Ponty1962),Footnote 1 are more experientially faithful descriptions of CDI: these formulae imply that one need go no further than movement tout court to ascertain embodied intention.Footnote 2 In CDI, the upshot is that the ensemble experience of togetherness is “kinaesthetic” (Himberg et al., Reference Himberg, Laroche, Bigé, Buchkowski and Bachrach2018). Experiments using hypercomplex signal correlation and probabilistic measures of entropy have been explored to test this “connectedness” empirically (Krzyzaniak et al., Reference Krzyzaniak, Anirudh, Venkataraman, Turaga and Wei2015). This approach links to precedent work in social psychology connecting movement coordination and sociality, which observes that “interactions often have an affective dimension in the sense that we can feel varying degrees of connection with the other” (De Jaegher and Di Paolo, Reference De Jaegher and Di Paolo2007). In our workshopping, “connectedness” or “togetherness” are not so much phenomena we seek to measure as ones we desire to enact, a corollary of our process-based approach. By way of example, consider the remark from one of our workshop participants that the sound generation associated with group orientation variance allowed her to “feel connected” in a way that made her rely less on her eyes. Through the process of familiarizing herself with a sonic response—but also co-designing that response with others while learning and moving with them over extended periods of time—the networked wearable DMIs allowed this dancer (and others) to attain a rather different sort of connectedness through bottom-up experiential approaches and embodied learning.
Collective Enactive Learning
To make better sense of what this dancer is expressing, some remarks on the enactive (and earlier autopoietic) noncognitivist approaches to thought informing our design strategies should prove helpful. According to this approach, a living system enacts a “world,” a cognitive architecture, by means of its ongoing continuous activity (Varela et al., Reference Varela, Thompson and Rosch2017). Cognition is tantamount to the process of life itself, “immanent in matter at all levels” (Capra and Luisi, Reference Capra and Luisi2014). In our workshops, the upshot is that the musical wearables adorning the bodies of the participants acquire such a function, just as refined sensory organs do in being shaped by a phylogenetic history of environmental coupling (Capra and Luisi, Reference Capra and Luisi2014). These architectures resemble “a patchwork of subnetworks assembled by a complex process of tinkering, rather than a system that results from some clean, unified design” (Varela et al., Reference Varela, Thompson and Rosch2017). The ongoing processes of refinement we pursue in developing these instruments abductively, through participation and without a priori models, actualizes a key affordance of real-time media processing with interpreted software programming environments, namely that one must no longer contend with a delay between composition and sonic actualization (Thorn and Sha, Reference Thorn and Sha2019; Thorn, Reference Thorn2021a). Rather, continuous reshaping of a body of code through trial-and-error tinkering refines and symmetrizes the sensorimotor dynamics of embodied action and perception, which are undifferentiated in the enactive approach. DMIs can be built on the fly without models, leveraging participatory dynamics to create a situation of collective enactive learning. Hence, the previously mentioned dancer could allude to the development of a collective sensorium, as it were, as a processual outcome of participatory workshopping with real-time, abductively constructed wearable DMIs. Abductive reasoning, likewise, is the mode of critical learning taking place in transdisciplinary computational creativity (Filimowicz and Tzankova, Reference Filimowicz and Tzankova2017). This design space addresses an aperture in the scientific literature being incipiently explored by a variety of MOCO researchers and practitioners bridging the artistry of interactive dance systems with scientific interest in movement sonification for sensorimotor learning (see Bevilacqua et al., Reference Bevilacqua, Boyer, Françoise, Houix, Susini, Roby-Brami and Hanneton2016; Giomi, Reference Giomi2020 for concise summaries of this research space).
Design of Special Hardware and Software Kits for Collectively Playable Wearable Music
The multifaceted term “workshop” suggests a range of diverse activities, including discussion, exchange, interaction, as well as do-it-yourself (DIY) communities and citizen participation (Jo et al., Reference Jo, Parkinson and Tanaka2013). Following precedent work in collective musical workshopping, we envisioned hosting workshops with movement-responsive musical wearables for expert dancers using aggregate sensing with rich and animating musical responses. This interest grew out of our previous collaborations combining sound and dance, and from our discussions about building more symmetrical relations among coding, choreography, dance, and musicianship. This work continues a line of research in responsive performance environments for dance with a long and formative history at our home institution, Arizona State University (Lovell and Mitchell, Reference Lovell and Mitchell1995).
Wearable Sensor Kit
The first author developed a wireless sensor kit based on a development board from Adafruit and an inertial measurement unit (IMU) from Bosch (BNO055) with 9 degrees of freedom (DOF) and on-board sensor fusion.Footnote 3 The boards are powered with 400 or 500 mAh lithium polymer batteries and connect as clients to a high-performance wireless router tethered to a laptop using wired ethernet. The microcontrollers transmit Open Sound Control (OSC) formattedFootnote 4 User Datagram Protocol (UDP) packets via Wi-Fi to the host server at a rate of 100 Hz, the full data rate capability of the Bosch IMU (Figure 2).

Figure 2. Left: the bespoke sensor kit. Center bottom: the first author using one of these sensors on his wrist, and (center top) the second author doing the same while dancing at the Intelligent Stage at Arizona State University. Right: some of the sensor placements we explored in the workshops.
Software Strategies for Collectively Playable Wearable Music
The host server runs an Ableton Live (AL) session and bespoke Max for Live (M4L) devices designed by the first author for synthesis, mapping, and aggregate statistical processing. AL is a popular commercial audio software capable of running as a standalone digital audio workstation or in tandem with an embedded version of the interpreted programming environment Max/MSP, an ambient data-flow software that has been the lingua franca of real-time media processing for several decades.Footnote 5 The real-time audio processing effects available in AL can be combined with the lower-level and more adaptable analog-modeled visual signal processing of Max/MSP, which are programmed as individual abstractions or “patches” that wrap various methods of scaling, ramping, interpolating, and/or utilities for time-series analysis, event detection, random sequences, and so forth.
We have found it to be difficult to design a collectively playable instrument from the ground up, but easy and efficient to adapt an individually playable instrument to collective playability—and quick if appropriate tools are available to perform the conversion. This is the primary thrust of the new utilities we present here. Prior to the workshops, the first author developed a set of bespoke M4L patches for quick construction of individually and/or collectively playable wearable DMIs.Footnote 6 The primary M4L patch used for this, a parameter mapping patch that maps streams of sensor data to audio synthesis, requires initial selection of a wearable sensor (associated with a UDP port) and IMU DOF or related feature (Figure 3). The control signal can be shaped with a variety of arithmetic or logical operations. An individually playable instrument is then quickly converted to collective playability by replacing the selection of a single IMU DOF with a statistical measure of the same feature aggregated across two or more IMUs, e.g., by exchanging the pitch of sensor n, normalized to a range of −1 to 1, with the coefficient of variance of pitch among a group of sensors normalized to the same range. This mechanism mitigates the jaggedness of real-time digital composing, the “detachment” or “disruption in flow” that characterizes the intervallic and tedious nature of DMI development (Magnusson, Reference Magnusson2009).
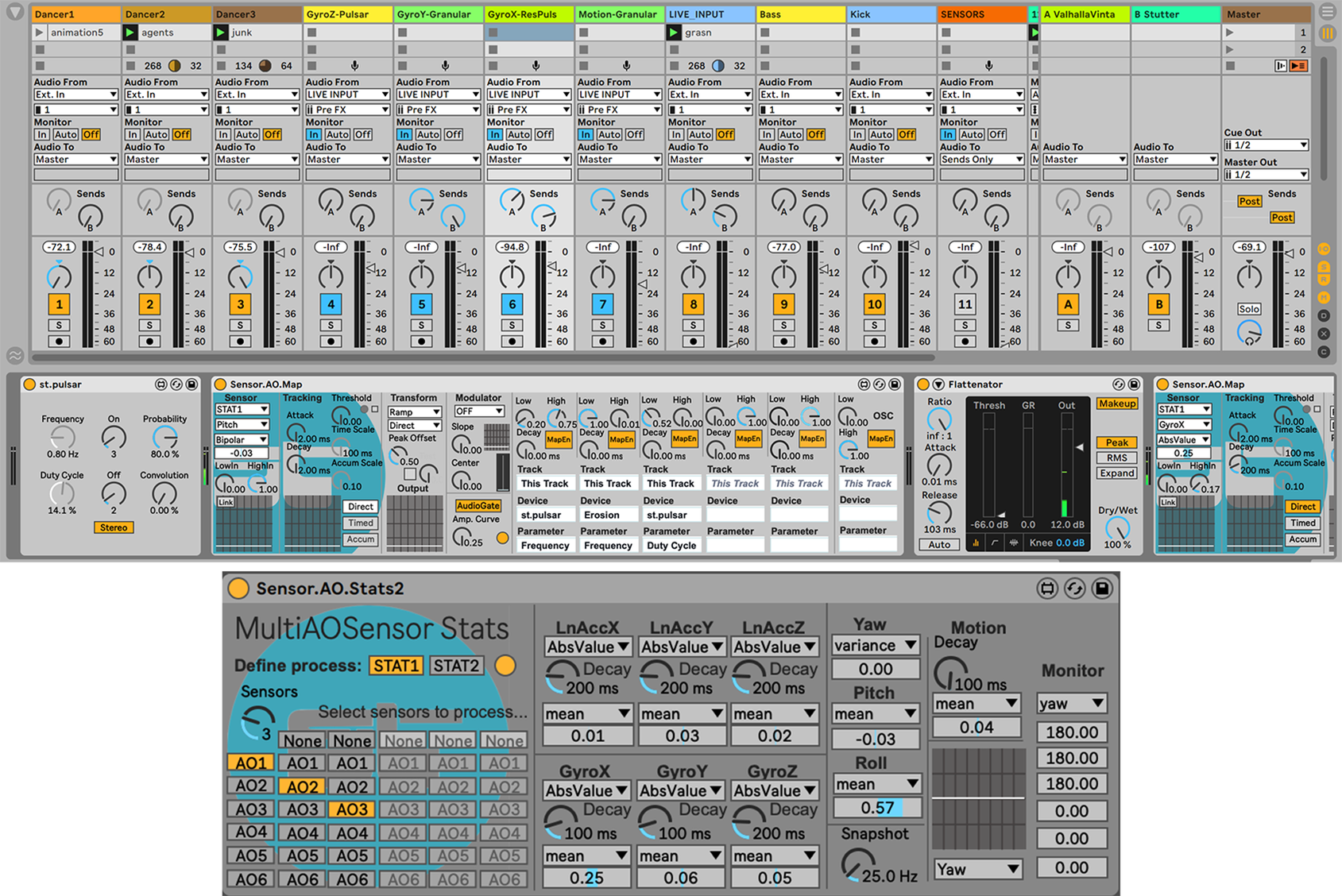
Figure 3. Top: an Ableton Live (AL) session with embedded Max for Live (M4L) patches. Vertical columns represent a bank of input channels as would be found on a traditional audio mixing console, with each channel providing amplitude gain, stereo panning, and routing options. The first three channels (Dancer1/Dancer2/Dancer3) contain pulsar synthesizers coupled to individual sensors worn by three dancers. Additional channels receive audio input from various synthesizers driven by collective mappings. In this figure, the channel Gyro-X-ResPuls is selected, revealing a corresponding audio signal chain in the lower third of the window. The signal chain moves from left to right, starting with a bespoke pulsar synthesizer followed by an instance of the specialized M4L mapping patch (Sensor.AO.Map) designed by the first author. In this case, an aggregate statistical feature, the mean pitch of the three inertial measurement units (IMUs), is selected and mapped to the frequency and duty cycle of the pulsar synthesizer, as well as an “erosion” effect (a short delay line modulated with filtered noise) that is placed further down the signal chain (clipped offscreen in this figure). Bottom: the M4L statistics patch designed by the first author, which allows aggregates of sensor streams to be defined and evaluated statistically and subsequently used as control signals by mapping patches.
Using a visual interface, the mapping patch makes available a variety of basic signal processing operations, such as scaling and clipping to remove noise and define dynamic range, as well as timers, accumulators, attack and decay envelopes, and functions such as inversion or other wavetables to creatively reshape and texture the control signal. This signal can be convolved with pitch, roll, or yaw angle, sensitizing to a region of movement via adjustment of a center peak and sensitivity breadth.Footnote 7 These simple operations present legible and potent transformations for workshop actors. Multiple mapping patches can quickly be instantiated in AL, allowing selection of additional features, signal transformations, and parameter mappings. In our design, the control signal from the mapping patch can be mapped to multiple parameters across the entire AL session, including global parameters in the master audio bus or signal chains associated with individual wearable sensors, which mitigates some of the linear design ontology of AL, a corollary of being modeled as a traditional audio mixing console.
In a typical use scenario, a mapping patch is used to couple 3D acceleration magnitude to the amplitude gain of an audio processing chain, which mimics the fundamental physics of an acoustic instrument by preserving aspects of energy continuity, while one or more additional patches are instantiated as modulation controls. This “supra-instrumental” approach, by which different gestural functions are abstracted from analysis of acoustic musical instruments—particularly gestures of “excitation,” “modulation,” or “selection” (Cadoz, Reference Cadoz2009)—is a useful starting point for building new DMIs in this otherwise highly abstract design space. Figure 3 shows the fully developed AL/M4L session that developed and crystallized over the course of our four workshops.
Strategies for Collective Mappings
Real-time windowed cross-covariance for correlated motion tracking of dancers is a well-researched technique (Aylward et al., Reference Aylward, Lovell and Paradiso2006; Krzyzaniak et al., Reference Krzyzaniak, Anirudh, Venkataraman, Turaga and Wei2015). Decisions about window size create a tradeoff between the maximum time separation among the correlations and windowing latency. Similarly, the M4L patch we built for statistical analysis of data from multiple sensors defines a temporal region of simultaneity by applying an adjustable decay envelope to individual sensor streams prior to the aggregated statistical evaluation (Figure 3). From the perspective of our processual and practice-situated approach, the windowing tradeoff defining what counts as “simultaneous” is less a theoretical issue than a practical one we approach experimentally/experientially to investigate how modulation of the decay envelope affects the CDI dynamics. Simple statistical operations such as minimum, maximum, mean, skewness, variance, or circular variance for yaw angle, can be selected and mapped with this patch. Other operations are available prior to the decay envelope (Figures 3 and 4). Several statistical patches can be used simultaneously if multiple modes of processing the same sensor stream are needed, or to define and process different aggregate configurations of data streams.

Figure 4. This diagram shows the signal flow between the mapping and statistics patches (shown in Figure 3).
Wearable Music Workshopping for Expert Dancers
We now proceed to our description of the workshopping events themselves. During these events, we worked with seven dancers altogether, inclusive of the second author. Four events took place over the course of 2 weeks with 3–5 dancers attending each session. The authors attended all sessions. The other dancers were current or former students of the second author’s university courses, but not all of them had previously worked with each other. Workshop sessions lasted between 90 and 120 min, a constraint mostly imposed by the physical strenuousness for the dancers.
Task-based Movement for Ensemble Thinking
To guide the workshops, we found inspiration in the toolkit developed by choreographer Nina Martin for group improvisation, “ensemble thinking” (ET) (Martin, Reference Martin2007). According to Martin, the goal of ET is to create “a performance language wherein creative choices made by the individual performer can be understood and acted on by the group” (Martin, Reference Martin2007). Through group exercises, the system trains dancers into awareness of the divergence between their “microlevel” personal movement vocabularies (“composing in the kinosphere”) and “macrolevel” compositional concerns in group improvisation—simultaneous awareness of what one is doing with one’s body and where one is in time and space (Martin, Reference Martin2007). This awareness is difficult to develop and maintain, especially with larger groups. ET uses exercises that promote simple decision making in the large group setting, facilitating quick, agile responses to the dynamic of emergent leading and following. We found additional motivation in the approach outlined by the Paris-based ICI research group, whose constituents investigate CDI experientially (Himberg et al., Reference Himberg, Laroche, Bigé, Buchkowski and Bachrach2018). ICI’s design principles for the phenomenological study of togetherness include open-endedness of movement sessions to enrich the first-person perspective and emphasize process, minimization of visual/verbal support to highlight and activate kinaesthetic experience, and “elicitation in the shifts in the patterns of behaviors”—nonlinear, chaotic bifurcations experienced as qualitative shifts in affective dynamics (Himberg et al., Reference Himberg, Laroche, Bigé, Buchkowski and Bachrach2018). A prosaic and straightforward example is the spontaneous concurrence that an open-ended improvisation is reaching an endpoint for the group (Figure 5).

Figure 5. Still frames from a durational score (from left to right, top to bottom) showing (a) emergence of leading (b) followed by reintegration, (c) a novel motif followed by call and response, (d) introduction of a second motif, (e) and introduction of a third motif leading to (f) group unison movement. The video can be viewed at https://vimeo.com/374805505.
The workshopping structure was conceived and adapted by the second author, who initiated each session using a guided check-in to promote relational awareness, followed by periods of play/discussion using the networked wearable sensors, and exploration of various ad hoc scores she generated on the fly. We employ the term “score” here loosely to indicate a set of instructions that delimit the movement content of an improvisation and influence its evolution, typically by prescribing physical tasks to be completed (Buckwalter, Reference Buckwalter2010). “Straitjacketing” the movement vocabularies of dancers to limit their personal movement vocabularies not only attunes the dancers to one another, but also brings more awareness of the media response to their individual and collective movements and bodily orientations. The second author blended phases of open play, informal and more formal discussion, and use of durational or concise scores according to the didactic needs she perceived. (These terms are defined in Table 1, with examples.) Durational scores tend to evolve through several emergent phase changes. Macrolevel awareness is facilitated in durational scores by lack of predetermined cues indicating an ending, with spontaneous collective awareness emerging to conclude the event. Durational scores, moreover, are emphatically loose: the prescribed tasks and awareness exercises are lightly held ideas, proposed yet open to deviation by unanticipated intervening forces. Thus, despite the prescriptive structural dimensions of these scores, they remain open in a way that supports a focus on process. The waves of learning that build up through multiple iterations and cycles of open play, discussion, and durational scores, prepare dancers for a culminating “concise” score that is time-limited and more resolutely task-oriented, since it assumes that dancers have developed broad macrolevel relational awareness of the group, as well as an understanding of the sensing and media response features (Figure 6). This heightened awareness of time and task fulfillment during concise scores has a compositional purpose: it requires dancers to make quick and agile improvisatory decisions that realize these tasks before reintegrating into the group.
Table 1. Fluid workshopping methodology


Figure 6. This diagram depicts the dynamic evolution of our workshopping qua structured improvisation: phases of proliferation and pruning, as well as parallel cycles of instrument adaptation and waves of learning by participants, mark iterative cycles of play, discussion, and exploration of durational scores. As in improvisation, there is a felt energy in the room vis-à-vis the learning and cohesion that have taken place, suggesting the potential of consolidation in a culminating concise score.
Progression of Sound Synthesis and Mapping Techniques
The first author sketched a collectively playable DMI that he brought to the first workshop as initial material. This instrument uses a variety of particle synthesizers that include pulsar, granular, and glisson instruments (Roads, Reference Roads2004).Footnote 8 Particle synthesis is especially useful in new DMI development for students without traditional musico-theoretical training, due to its thematization of physical features less freighted with music theory (e.g., density/spatiality vs. notes/harmony). Granular synthesis generates sound by indexing an audio buffer that is granularly dispersed by synchronous or asynchronous clocking of randomly selected playback positions, grain durations, sampling-rate alterations, or other algorithmically determined transformations. Alternatively, live audio input can be recorded into a circular buffer that is granulated and periodically overwritten with new audio input. In the basic instrument prepared for these workshops, extensive use of the circular technique was made: an 18-s audio loop composed by the first author, embedded with shifting musical harmonies, is continuously looped and played into a short circular buffer referenced by multiple granulators. Features of these granulators are in turn activated and modulated by the wearable sensors in real-time in synchrony with the playback of the loop, which gives compelling musical consistency, material variation, and forward propulsion. The loop can be replaced with a different sound file, preserving the formal structure of the instrument while immediately transforming its material and connotative qualities. Since the varieties of particle synthesis used in this instrument evoke ebullient, percolating, and continuously evolving sonic textures, we named it mare vaporum, “sea of vapors,” after the eponymous sea on Mars.
As we discovered, however, initiating a workshop with collective mappings is mystifying for the participants. It is much more skillful to begin with individual voicings that demonstrate clear relationships between individuals’ movements and sound. We quickly maneuvered to such voicings by coupling three pulsar synthesizers to the 3D acceleration magnitude of the three individual sensors, differentially spatializing them within the multichannel sound stage to improve learnability of the kinesics.Footnote 9 Pulsar synthesis was selected due to its clear control structure for manipulating density and spectral brightness, as well as its continuous rhythmic and versatile timbral affordances. In acoustic instruments, spectral complexity and amplitude tend to be highly correlated, thus making for an adept design choice in inceptive DMI designs. Thus, coupling and scaling the 3D acceleration magnitude to amplitude, frequency, and duty cycle parameters of the pulsar synthesizer quickly generates a compelling and intuitively playable wearable DMI.
Once the dancers became familiar with the response and sensitivity of the individual sensors and pulsar synthesizers, we began experimenting with richer and more intricate instruments and collective translations. These instruments progressed through phases of adaptation and transformation, a process diagrammed in Figure 6. Omitted in this figure is the orthogonality of these processes, the gradual convergence of the different expertise domains as the participants become more attuned to the technical practices of the others. Moreover, the workshop itself has the character of a structured improvisation marked by periods of proliferation and pruning, a trial-and-error dynamic in which potentialities of the assemblage are teased out by assessing the outcomes of off-the-cuff experiments and seizing on the most palpable and expressive structures (Angelino, Reference Angelino2018). Proliferation proceeds rapidly as new ideas and mappings are quickly implemented and explored, blending experiments with both individual and collective mappings. “Pruning” indicates processes of selection as well as refinement and exfoliation. This dynamic plays out across multiple timescales and structural levels: not only at the level of the domains of individuals’ technical expertise (i.e., expressive movement, dance scores, sound mappings, and synthesis algorithms), but also vis-à-vis the 2 weeks of workshopping, which converges on a final concise score (described in Section 4.4).
To lay out this process as a sequence of steps goes against the grain of the dynamic they represent. Nevertheless, the following procedure captures aspects of the knowledge we acquired that can be brought to future workshopping events and research-creation:
-
1. Begin with some preliminary movement scores and mappings of movement to sound. Allow workshop participants to explore individual voicings.
-
2. Invite dancers to play with these preliminary voicings; discuss their experiences with them; vary, switch, and iterate voicings into more fleshed-out instruments mapping movement to sound.
-
3. Create a rich instrument that is individually playable, then exchange the individual mappings for the respective collective parameters.
-
4. Provide more open sets of movement guidelines for the participants to work and improvise with. The instrument behavior can be modified ad hoc. The collective can intensify the felt experience of the improvisation by gradually adding new sounds and sensitivity to different movements. Learnability of the kinesics can be balanced with dynamic modulation of the instrument.
Wearable IMU Placement
One obstacle we encountered during the workshops is the surplus attention drawn to the wearable sensors and consequent congestion of group improvisations. Despite their diminutive size, wearable sensors somatically freight the bodies of the participants by drawing awareness to the location of the sensor, altering the first-person kinaesthetic experience of the body (Höök, Reference Höök2018). We experimented with placing sensors on the foot, head, and shoulder. Distal placement strongly choreographs movement. Placement atop the head using a headband, for instance, encourages dancers to initiate movement there. Observing this, the second author spontaneously joined the group as a noninstrumented dancer who could relieve this congested movement by corporeally proposing less sensor-centric movement motifs. In technology-augmented dance, noninstrumented dancers can make choices or introduce motifs not driven by the presence or position of the sensor on the body or sonic response to movement. Dancers wearing sensors are relieved by this new space for choices that are less wearable-focused yet still wearable-influenced—they become receptive to “exterior” influences that may catalyze new intensifications (refer to the video at https://vimeo.com/373915236 for an assemblage of instrumented and noninstrumented dancers). Prior to the third workshop, we experimented with placement atop the right shoulder near the neck, which proved successful for the purpose of promoting unreserved full-body motion rather than intentional movement from the sensor in the form of distal initiation and limb-specific gesture. Proximal rather than distal placement of the wearable sensor is a more agnostic way of instrumenting dancers, and we propose that there are somatic reasons for this based on an understanding of fundamental core-distal connectivity, whereby by the navel “functions as a primitive center of control” (Bainbridge Cohen, Reference Bainbridge Cohen1993).Footnote 10
Culminating “Concise” Score and Collective Instrument mare vaporum
Over the course of four workshops, we progressively developed a coherent and nuanced collectively playable instrument (Table 2 summarizes its features). Since no dancer attended all the workshops (typically two of the four, apart from the continuous presence of the second author), this created an interesting dynamic, as dancers would return to an instrument that had developed further. The culminating event of each workshop is the realization of a concise score. In the final workshop, the dancers called this score “Pillow Score” due to their use of large pillows we found in the black-box theater space and incorporated as enriching compositional elements. During this final workshop, we also experimented with an unusual mapping that transformed the entire mare vaporum instrument, namely the coupling of group movement intensity (aggregate mean 3D acceleration magnitude) to diminishing amplitude and increased lowpass filtering of the entire instrument. This was easily implemented by adding a mapping patch and lowpass filter to the master audio bus in AL. As a result, a narrower range of movement activates the original parameter mappings, while excessive movement attenuates the response of the entire instrument, including individual mappings. Modification of the decay parameter of this inverted envelope, the rate at which it bounces back to an unattenuated state, strongly conditions the movement of the dancers by determining how gradually the instrument becomes sonically enlivened again (refer to the video at https://vimeo.com/393185524 for initial exploration of a shorter rebound, and to https://vimeo.com/375157582 for excerpts from “Pillow Score” showing a much slower rebound). This greatly intensifies macrolevel awareness: anyone who moves too much blunts the sensitivity of the instrument for everyone else, which instantiates a distinctive economy of collective intention and attention.
Table 2. Mare vaporum instrument

Below, we reproduce the second author’s instructions for the culminating concise score in the last workshop, “Pillow Score.” Tasks may occur in any order. As with durational scores, these are baseline instructions that dancers may ultimately choose to ignore or vary, their purpose being to generate a lexicon of motifs that orient dancers throughout the event by conditioning, but not determining, its concrete unfolding. This openness allows the actors to exercise their own artistry by following these motifs or generating novel ones on the fly. Likewise, emphasis on the concision of the score is more about conditioning the vigor and pace of activity and artistic decisions—the “felt time” of the event experienced by the body (Wittman, Reference Wittman2016)—and less about predefining a firm and objective time constraint. Instructions to embellish or revel in different tasks generate excitement and encourage introduction of novel movement motifs.
“Pillow Score” (three dancers)
-
1. A dancer is covered in pillows. The other dancers enter one by one.
-
2. The dancer emerges from the pillows.
-
3. Dancers revel in the activity of clearing pillows from the stage.
-
4. Dancers find and engage in moments of unison.
-
5. Dancers indulge in sensor play.
-
6. Two solo moments and one duet moment are allowed. Movement energy is vigorous in solos but more restrained in duets.Footnote 11
Collaborative Telematic Wearable Music (Remote Course)
Practice-Situated Telematic Research and Collaboration
The first author consolidated materials and approaches from the workshops with dancers to prepare a distance learning wearable music course he taught in Spring 2021. The course explored networked, wearable, movement-responsive instruments to shore up and remediate the lack of shared social and tangible affordances in virtual classrooms. This work echoes precedent work in sonic interaction in mixed-reality environments using spatial affordances (e.g., Wozniewski et al., Reference Wozniewski, Settel, Cooperstock, Franinovic and Serafin2013) but is a unique approach. In the context of telematic learning, collaborative wearable music also presents an opportunity to address fatigue from tedious foveal/screen-based interaction, a problem taken up by other mixed-reality techniques (e.g., Montpellier et al., Reference Montpellier2015). Can we interact without a screen using sound and rudimentary wearable sensors? Can these foster a sense of telematic togetherness by facilitating more spontaneous and embodied interactions? What is the minimum signal needed to feel connected, and how scalable are the techniques? We reviewed telematic sonic interaction design and reflected on problems with remote and augmented collaborative learning (Akçayır and Akçayır, Reference Akçayır and Akçayır2017).Footnote 12 Due to the pandemic, it was necessary to adopt off-the-shelf, inexpensive, agile tools that could be continuously adapted to different and evolving needs, rather than designing bespoke interfaces under more idealistic and controllable circumstances. One is hard-pressed to find circumstances more apropos than a global pandemic for practice-situated exploration of telematic, embodied togetherness using networked wearable media.
Wearable Music Course Design
The wearable music course is conceived for epistemically and culturally diverse undergraduate students. Five students were music majors, with 14 others coming from design, film, informatics, animation, and electrical engineering. The gender balance in the course was more favorable than is typical of an “electronic music” course, with 7 students identifying as female and 12 identifying as male. Wearables were recognized early on in affective computing as trenchantly affective adornments due to their intimacy with the body (Picard, Reference Picard2000). Designing and presenting the course around the experiential poetics of “wearable music” perhaps makes this research-creation more appealing to many students, not only by suggesting playful interaction rather than mastery, but also because of the body-centric metaphor, which potentiates a discourse around electronic and digital music contrasting with its normatively gendered and combative machinic tropes (e.g., of “triggers,” “controllers,” “commands,” “bangs”) (Rodgers, Reference Rodgers2010). In the course, students acquire skills while working on individual projects, then explore collectively playable instruments later in the semester. The course is divided into four modules comprising two class sessions for 3 to 4 weeks each.Footnote 13 This structure is outlined in Table 3.
Table 3. Wearable music curriculum

Abbreviations: IDE, integrated development environment; API, application programming environment.
Low-Cost Off-the-Shelf Wearables
Students acquired two M5Stick-C wearablesFootnote 14—an off-the-shelf, inexpensive IoT platform—along with a wireless portable router, the TP-Link N300, which they used as an access point. Acceleration, angular velocity, orientation, and battery voltage readings are sent as OSC-formatted UDP strings and received in Max/MSP. The sensor is small and embeddable, with a built-in 80 mAh or 120 mAh lithium polymer battery, thin-film transistor (TFT) screen, and MPU6886 6-DOF IMU.Footnote 15
Wearable Music and Felt Time
As in the workshops with dancers, granular synthesis is fundamental to the sound design component of the course, along with physical modelling synthesis (simple excitation/resonance models), arpeggiating control of wavetable synthesis, and streaming input from students’ YouTube or Spotify playlists modulated with real-time audio effects from physical activity. Appreciation of atmospheric and rhythmical aspects of music (Böhme, Reference Böhme2017) are favored over western musico-theoretical analysis to create an inclusive classroom emphatic of “felt experience” (Gendlin, Reference Gendlin1997). As with the mare vaporum instrument used in the workshops, cogent musical samples selected by students are parsed into short loops to create rich starting material encoding musical consistency and forward-impetus. Students reviewed empirical work evaluating the effect of musical agency on perception of physically strenuous performance (Fritz et al., Reference Fritz2013) as well as research on time perception as integration of bodily signals (Wittman, Reference Wittman2016). Taking this awareness into practice, students were encouraged to experiment with relations between time and movement, not only to modulate their perceptions of existing performance practices, but to invent new ones—musical wings, “wind chimes” earrings, or recovery of “lost” sounds through movement, are examples of enchanting projects students created (Figure 7). As a technical foundation for this work, students are shown how to accumulate 3D acceleration magnitude to drive the looping playback head position of a granulator, producing a granular time-stretching effect.Footnote 16 For further inspiration, they watched documentation videos from the wearable music workshops, observing the experiments with the “inverted” mapping applied to the master audio bus of the mare vaporum instrument and the potent effect it had on the improvisation dynamics by modulating movement intensity and augmenting macrolevel awareness. This work lays a technical and experiential foundation for exploration of collectively playable wearable instruments later in the class.

Figure 7. Students worked on individual wearable/movement/sound projects throughout the course. While preparing their final projects, we used class time to explore collectively playable instruments and surplus time for open labs. This figure shows a variety of creative final projects (from left to right, top to bottom): sonification of wings with two sensors, a project for encouraging fitness, a game in which users collectively search for hidden sounds, sonification of writing/drawing, movement-responsive “wind chimes” earrings, musical gloves, wearable augmentation of guitar playing, an automatic timing device for holding and changing yoga and stretching poses, and a student’s young relatives augmenting ballet poses and dance with wearable music.
Jump-Starting Rich Explorations Using Parameter Interpolation
Wearable DMIs should render spectrally rich sound with meaningful physics. In this article, we have emphasized that DMIs become highly refined in evolving through exploratory abductive tinkering. To jump-start rich explorations in the wearable music class, students are provided a Max/MSP patch containing an encapsulation of a granular synthesizer exposing high-level features such as density, playback speed, and grain duration, along with spatial reverberation, lowpass filtering, and amplitude tremolo effects. Altogether, this offers a rich and parametrically dense suite of compelling tools for sound generation. An abstraction available in Max/MSP, pattr, can be used to quickly store and interpolate among unique parameter configurations. By mapping a sensor feature to the interpolation parameter, students can rapidly prototype wearable DMIs that implement a rich “one-to-many” mapping. As in the workshops, this design tool centers attention on experiential inquiry—the technical details can be fleshed out later, after student interest is piqued Figure 8.
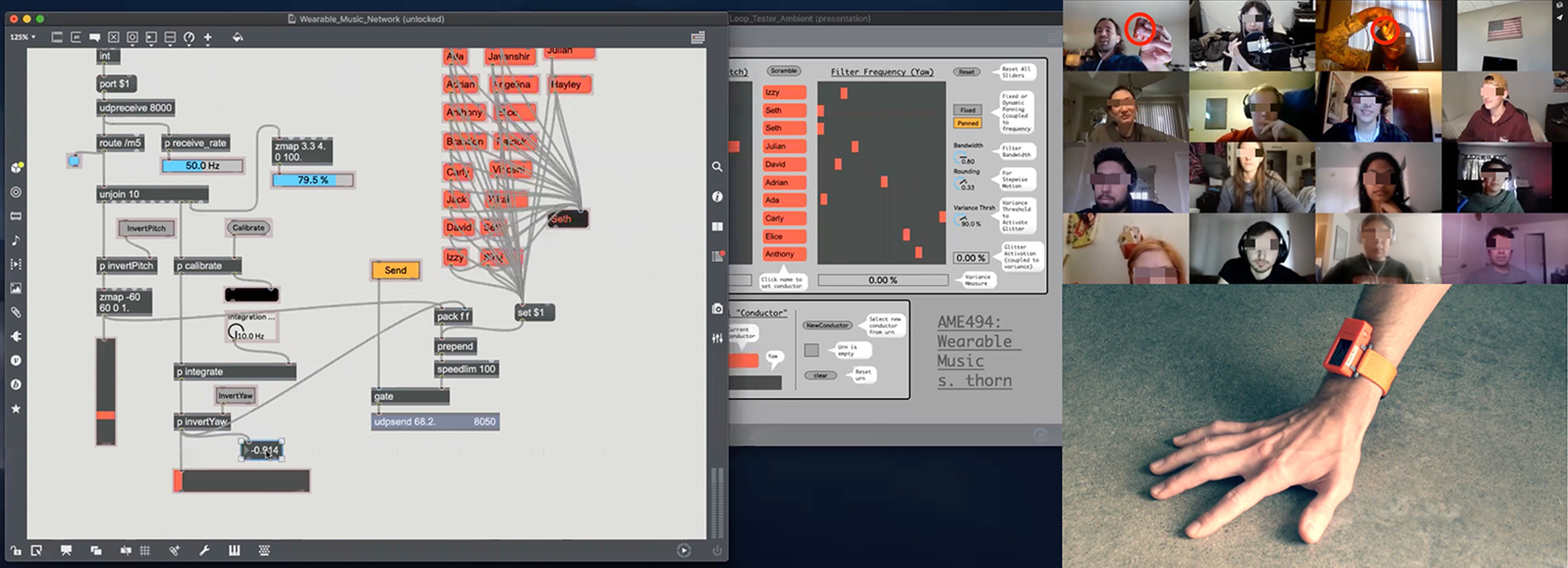
Figure 8. Left: screenshot of a Zoom session showing live editing of a Max/MSP patch in class to change the group’s instrument (see the video at https://vimeo.com/571056944). Top Right: zoom session showing first author and student “pouring water” back and forth between coupled wearable sensors. Bottom right: video still of a wearable digital musical instrument (DMI) demonstration shown to students using the pattr abstraction example patch (see Section 5.5; related video can be viewed at https://vimeo.com/571058528).
Relational-Experiential Media: Dyadic Instruments
We designate interactions involving two sensors as “dyadic,” reserving the term “collaborative” for the different interaction dynamics manifesting with DMIs involving three or more sensors. To introduce the unit on relational and telematic media, students were shown how to work with data from two sensors to calculate angle divergence, as well as integration of angular velocity to track the “winding” of one sensor in relation to another. The latter can control the resonant frequency of a virtual elastic band stretching between the sensors by implementing a simple Karplus-strong algorithm.Footnote 17 Students then experiment with replacing one of the sensors with remote user input whose unanticipated activity may spur fresh design thinking by subverting expectations–often humorously–and intensifying affective engagement and discussion. While we explored peer-to-peer connectivity initially, blocked ports or other obstacles made this less feasible. Instead, collaborative telematic sessions are hosted centrally during class time by the instructor using a simple client-server model: students bounce OSC-formatted UDP packets from their wearables to the host server and receive broadcasted audio feedback via the Zoom video conferencing platform. The network topology is shown in Figure 9.

Figure 9. Network and audio processing topology of a simple entrainment instrument we explored.
To provide a foundation for considering the relational dynamics of dyadic interactions, students reviewed a minimalist perceptual crossing experiment conducted by Auvray, Lenay, and Stewart, which explores recognition of intentional behavior by two isolated subjects whose movements are to be differentiated from static objects and moving lures (Auvray et al., Reference Auvray, Lenay and Stewart2009)Footnote 18 This experiment eloquently reflects our interest in understanding minimum signals of connectedness. We imitated it by exploring remote entanglement of two networked wearable sensors whose pitch angles are compared using an inequality operation. Logical transitions generate an audible “click.” We embellished this by using different sounds for rising/falling logical transitions, such as different musical tones or resonant lowpass filtered noise sweeps that glide up or down in the direction of the transition. To enrich this model, we explored continuous “brushing” that replaces the discrete inequality calculation: absolute difference of the pitch angle of two sensors is inverted and scaled to create a region of sensitivity reaching a maximum value when the pitch angles are equivalent. This value scales the amplitude of clicks generated when activity occurs within the sensitive region. Subsequently, students learn rudimentary physical modelling synthesis by using these unit impulses to excite a bank of tuned resonant filters.
Students found these models conceptually intriguing in terms of the perceptual, networking, and synthesis concepts they introduce, but disorienting as an interaction paradigm. The multiple degrees of freedom of the IMU create mystifying kinesics for the students, leading to feelings of unpredictable responsiveness. As an alternative, we conceived of a relational physical model in which two remote sensors continuously “touch,” as it were, reminiscent of contact improvisation dynamics. Students were able to implement this idea using the physical modelling principles they had previously learned: one remote sensor performs an excitatory function (transient clicks) while a second performs a modulatory function that changes the resonant properties of the resonant filter bank. Students found this to be a more favorable interaction paradigm, so we extended it into other experiments, such as a 64-band frequency-domain equalizer that can be shaped with yaw and pitch angles affecting band selection and gain, respectively. The more continuous affordances of these interactive designs yield a better “grip” on the interaction, which we found to be prerequisite to any meaningful experience of telematic togetherness.
Relational-Experiential Media: Collaborative Instruments
The “Global String” installation by Atau Tanaka demonstrates an intuitive conception of a resonant corpus shared between remote locations with continuous responsiveness, such that any number of individuals may collectively strike and shake the instrument (Tanaka and Bongers, Reference Tanaka and Bongers2002). We explored a similar idea by dividing the class into two groups on opposing sides of a “tug of war” game. Angular velocity of the z-axis of the gyroscope from all networked sensors is integrated using an accumulator running on the host server mapped to one or more audio synthesis parameters. As one group tries to drive the accumulated value higher, the other attempts to pull it lower. As an interaction paradigm, this is a helpful model to start with, insofar as individuals retain a sense of efficacy in the interaction as the accumulated value moves above or below zero—even as the number of participants is scaled up—whereas this is often not the case in larger group interactions. On the other hand, this interaction affords little relational awareness of how one is involved with the others. In other words, we experienced the same tradeoff between knowing what one is doing and where one is that afflicts CDI. It was helpful for students to consider the crossover with CDI approaches. This allowed us to begin articulating critical affordances for success in collaborative telematic wearable music.
As we learned from choreographer Nina Martin, the complexity of large groups tends to overwhelm the efforts of individual actors, multiplying the need for simplicity in improvisations (Martin, Reference Martin2007). To explore this problem, we used simple models that we progressively scaled up by increasing the number of participants. For instance, we explored entrainment as a potential solution to the latency richness of telematic music. Students run Max/MSP patches that calculate the median of a sliding window of time intervals between IMU zero-crossings. As a participant moves the sensor back-and-forth in a periodic manner, the windowed median is sent to a host server that generates responsive sound by applying amplitude tremolo Footnote 19 to an individual filter within a tuned filter bank. Multiple students may be involved, with each remote sensor coupled to an individual tremolo rate of a different filter (Figure 9 shows this instrument topology). We discovered that, as individuals entrain the system, the system entrains them due to the strong sonic feedback signal, circumventing the latency problem. As the number of participants is scaled up, however, the bandwidth of the filters must decrease as new filters are added, and it becomes harder to perceive any one individual’s effect on the instrument—such is the zero-sum economy of spectral bandwidth. Another problem with this instrument’s design is the pre-schematization: individual sensors are rigidly coupled to different filters, a rather different paradigm than Tanaka’s string or Cage and Cunningham’s epochal responsive theatrical stage. At this point, the students in the class have accumulated enough experience that we can begin to articulate some guiding principles for collaborative telematic wearable music. Our first principle is:
-
1. Unschematized interaction: instruments should be adaptable to changing numbers of participants and continuously responsive to gesture and unanticipated forms of play.
We tried implementing this principle by redesigning an entrainment-based DMI that could accommodate varying numbers of participants without requiring rescaling of the instrument (e.g., filter bandwidths or other parameters). On the host server, a sound file is played back at 16 different sampling rates according to a three-note ascending scale (fourth, fifth, and octave), creating 16 individual but collectively harmonious “voices.” Amplitude tremolo is applied to each voice individually at intervals set according to a subharmonic ratio starting from 1 Hz, continuing by increments of 0.25–4.75 Hz. Students transmit the same periodic zero-crossing information as before, with the host server now rounding these values to the subharmonic intervals. These packets are routed into leaky counters coupled to individual voices, increasing their amplitude gain as packets accumulate. The idea is that sustained periodic movement of the sensor will excite the corresponding “resonant” frequency of the instrument, while amplitude tremolo provides entrainment feedback. Importantly, any number of students may participate at any moment, fulfilling our first design principle. While presenting an intriguing conceptual model alloying entrainment and resonance, it was difficult for students to perceive a relation between the entrainment and the sound generation. The situation became intractable when more students were involved. One of the difficulties is the narrow bandwidth of the individual “resonators,” which are narrowly spaced by 0.25 Hz intervals. This makes it challenging to deliberately sustain activation of a particular voice due to the complicated structure and slow response to students’ movements. Although we abandoned this model in the class to move on to other experiments, a simpler instantiation with fewer voices and wider bandwidths may be a good starting point for developing a better instrument. In any case, this led us to formulate a second principle, already well known in telematic music:
-
2. Accommodation of latency-rich networks: interactions should be based less on precise timing, perhaps by placing more emphasis on sensor orientation as input. However, entrainment paradigms may still work for simple interactions.
This second principle drew us to examine telematic orchestra projects. Musical works performed by these groups are commissioned to accommodate latency rich environments by requiring less precise timing (Rofe et al., Reference Rofe, Geelhoed and Hodsdon2017) and by construing network latency as a musical affordance—a propagation medium—rather than unequivocal privation (Chafe, Reference Chafe2009). As in traditional orchestras, a conductor helps the group to synchronize visually—even in concert halls, this visual aspect serves an important function in dealing with the latency of the auditory channel (Blesser and Salter, Reference Blesser and Salter2009). In our case, we adapted these features by designing a collaborative instrument requiring less precise timing, the central feature of which is use of an auditory conductor, which was suggested by a student.Footnote 20 The instrument generates an ambient soundscape based on granular synthesis of a short loop passing through a bank of 10 resonant bandpass filters with equal energy distribution across the audible spectrum. Q and gain parameters of the 10 filtersFootnote 21 are coupled to the pitch angle of individual remote sensors, while yaw angles of these sensors are coupled to linear panning and different preselected frequency ranges for each filter, manipulated using stepwise motion to enhance the feeling of “grip” via this latching. Among the 10 participants, one is selected to be the auditory conductor, with the sensor yaw and pitch angles additionally controlling the amplitude and panning of a spectrally distinctive voice that is created by pitch-shifting the generative sound down by one octave. Tremolo and lowpass filtering of this voice are also controlled by the orientation of the sensor to make it more dynamic and expressive. To hyperbolize the spatiality of this voice, binaural panning is used to help further differentiate it from the other voices by additional spatializing cues as students listen through their headphones. As the conductor voice moves, students are asked to track it with corresponding movements. As everyone moves across the stereo field, the spectral quality of the instrument changes as the filter frequencies are all pulled up or down in tandem. Finally, the variance of the yaw angles is calculated: as variance decreases, the gain of an additional voice is increased, which is generated by a synchronous granular signal capture buffer with playback pitched up several octaves by increasing the sampling-rate. (Refer to the video at https://vimeo.com/571056944 for documentation of the in-class development process, including student suggestions and feedback, on the fly adaptation, and collaborative playing. Note that not all students elected to have their camera feeds on).
Among the instruments we had previously tried—dyadic and collaborative paradigms alike—this one received the most favorable response from students, who expressed a general feeling of ensemble (“it feels really collaborative”—see Appendix A) and engaged with the instrument for a significant period. Our exploration of these collaborative instruments mirrors the use of durational scores in our dance workshops: there is a mixture of verbal and nonverbal exchange, implementation of small control changes, and exchange of individuals’ roles in the group. CDI manages collaborative complexity by using task-based scores that skillfully condition distributions of leading and following. Orchestras, by contrast, typically employ a fixed leading position and top-down organization. Our instrument explores spontaneous exchange of the leading auditory conductor position, bringing humor, differentiation, surprise, and anticipation to the collaboration. For instance, at one point the first author noticed that a student was either struggling to align with the others or had simply stopped paying attention. In response, this student was given the conductor role, a solution which also sharply reattuned him to the spatialized ensemble that was now following his movements. Despite the fixed coupling of sensors to individual voices, the relative success of this instrument suggests additional design principles derived from the discussion with students:
-
3. Leverage leading/following dynamics: to manage the complexity of increasing numbers of participants, employ this paradigm to generate feelings of collaboration. This makes the interaction more compelling by potentiating sudden playful transformations of the group dynamic, which also creates a sustaining element by making the interaction more engaging for longer periods of time.
-
4. Encourage macrolevel awareness: to facilitate this, clever parameter mappings can be devised that respond to measures of alignment, group synchrony, or other events. (Note the emphatic reaction to aggregate orientation variance sonification both here and in the dance workshops).
-
5. Rich sound: more intricate, composed, evolving soundscapes steered with higher-level parameter mappings make for more engaging instruments in collaborative telematic wearable music, where parameter mappings must be kept relatively simple to remain palpable and effective. Simple mappings do not require simple sounds, which will quickly lose novelty.
Further consideration might be given here vis-à-vis tradeoff of the auditory conducting position by algorithm: not merely by chance, but based on the contingent activity of the ensemble, perhaps by employing a game-like structure that further engages students’ interest and intention/attention. At the same time, a performative and expressive understanding of play discloses how the meaning of a game derives not from design intentions but from what players actually do (Sicart, Reference Sicart2011)—a good reason to adhere to our first design principle, which calls for less schematized instruments that refrain from embedding strong goals or interactive intentions. Task-based scores may be explored to furnish the leading/following dynamics and shore up macrolevel awareness, so that the instrument can remain less schematized and more continuously responsive and resonant. Continuing this line of thought—looking for ways beyond the algorithm to intensify a feeling of collective togetherness—we can add two additional design principles, the first of which we discovered fortuitously when a few students in the class were already streaming packets from their wearables prior to any speech or video streaming.
-
6. Prioritize the instrument: before initiating other forms of telematic engagement (video and speech), explore the instrument as the sole means of interaction. This has a remarkable attuning effect on the interaction, renewing the uncanniness of the telematic.Footnote 22
-
7. Encourage embodied interaction: if students are willing, have them place their sensors proximally rather than distally to encourage full-body movement. This enhances senses of spatiality/sociality and dampens fixation on manipulation of the sensor itself.
Conclusion
The instruments we explored in the wearable music class and workshops were engaging, in part, by virtue of the processes through which they were collectively enacted. Student reviews compiled at the end of the course suggest that this participatory dimension challenged their perception of the educational context in a favorable way. As one student wrote in the anonymous course reviews, “It felt like we were not in class, but were a think tank of sorts, which was very refreshing.” It is significant that fewer than a third of the students in the course were music majors, with most coming from other disciplines or academic departments, and that there were no prerequisites for technical competencies. The objection could be raised here that, considering the minimal criteria for enrollment, given the somewhat technically rigorous and specialist content of the course, how could the outcome be more than a “tokenistic creative agency”? (Tanaka and Parkinson, Reference Tanaka, Parkinson and Emmerson2018). An important factor is that the students had the benefit of 12 weeks of prerequisite projects and individual learning before reaching the final month of the course in which we explored collectively playable wearable DMIs together. Co-design does not always have the benefit of such drawn-out exchanges and learning. As for the dance workshops, a less sympathetic reader could emphasize that participation is thin vis-á-vis the sharing of technical competencies, but this overlooks the positive outcomes reported by the participants, while misplacing the emphasis on acquisition of preexisting technical competencies, whereas the salient generative dynamic in participatory design resides in the encounter of different tacit and embodied knowledges productive of new forms. Indeed, participatory design is sometimes viewed as a technique for drawing out tacit knowledge (Spinuzzi, Reference Spinuzzi2005). As design theorist Anne Balsamo writes cogently, “diversity among design participants is generative…because people embody different sets of assumptions” (Balsamo, Reference Balsamo2011). Here again, we invoke the notion of the rich and diverse, heterogenous “assemblage” paramount to both the MOCO ethos and transdisciplinary research-creation more generally. In the crisp synopsis of MOCO researcher Jan Schacher:
Research about movement-and-computing occurs at the intersection of several disciplines and perspectives. They meet and are mixed in ways that are less the result of deliberate choices and conscious engagement and are more contingent on the background, schooling, and practice of each actor. (Schacher, Reference Schacher2018)
Tacit embodied knowledge is the grist in the mill of practice-situated research. In the dance workshops and wearable music course, we built up collectively playable wearable instruments through co-design processes with epistemically diverse participants and movers in vibrant and complex assemblages. We deliberated verbally and corporeally, collaboratively tinkering with nuances in the signal processing, while observing how certain changes affect and vary how we play together and inform our sense of togetherness. In the process, we learned and adapted, orienting ourselves to novel feelings, sense, and sensations. With somatic and sonic finesse, we engaged with each other by proposing motifs with our bodies and with the new sounds those bodies produced, coupling movement to the sonic in ways that evoke friction, resistance, precarity, fickleness, pressure, or vibration. Although practice-situated research disfavors hard idealizations, explicitness, and discreteness, inasmuch as these would blunt the experiential phenomena, these inquiries are precisely experimental to the degree that the results they produce condition the dynamics of the collective action in reproducible ways (Sha, Reference Sha2016). In both the workshops with the dancers and wearable music course, we were able to elaborate designs for networked wearable DMIs that affect our sense of togetherness, yet we are only scratching the surface of those potentialities, and we hope that our experiences will prompt others to pick up from where we have left off.
In summary, our design process benefited from the enriching complexity of movement styles and histories brought by the various actors (dance, yoga, violin, and sports), their divergent epistemic cultures (engineering, performing arts, and computer science), as well as the material aspects of the physical or mixed-reality spaces (pillows, latency, and headphones), and the constraints of the wearable technologies themselves (their tendency to draw a surplus of attention, for instance)—material flows that surface and are followed by intuition and chance. This assemblage, the ecology of practices exemplary of MOCO research-creation, animates a design situation that is new. By drawing on different embodiments and divergent practices, there is fresh potential to steer away from well-worn and unobserved disciplinary grooves. Moreover, this way of doing things will not forego the possibility that a solution may emerge in advance of a problem, a salient aspect of the abductive process. A fine example is the experiment with the collective “inverted” instrument, which surprised us in its manner of delicately attuning movers’ intention/attention to the others. There are certainly no acoustic instruments that get quieter the more intensively they are played—this is precisely the enchantment afforded by the DMI design space and process. The skillful intuition (and chance) involved in that development not only comes from the generative collision of practices, but from the incremental refinements enabled by lower-level signal processing and abductive trial-and-error approaches responsive to movement tout court. In this way, we arrive at refined DMI designs, using networked wearables, by following practice-situated “intuition in action” (Deleuze and Guattari Reference Deleuze and Guattari1987).
Acknowledgments
The authors are grateful to the referees for their helpful suggestions. We also thank the Synthesis Center at Arizona State University for hosting our workshops, and the School of Arts, Media and Engineering at ASU for purchasing equipment for the students in the wearable music course. We thank Sha Xin Wei for his helpful advice during the dance workshops, and give much gratitude to John Mitchell, who introduced and us and inspired us, and who led pioneering work at our institution in the formative period of interactive dance research during the 1990s.
Funding Statement
The School of Arts, Media and Engineering funded purchase of materials for the wearable music class. Funding for development sensor kit in the workshops came from an internal seed grant for S.T. from the Herberger Institute for Design and the Arts at Arizona State University.
Competing Interests
The authors declare no competing interests exist.
Author Contributions
S.T. is responsible for software and hardware used in the workshops and taught the wearable music course. H.W. recruited dancers for the workshops and is responsible for the choreography.
Data Availability Statement
The full collection of supplementary videos can be viewed here: https://zenodo.org/record/5762453.
Appendix A
Verbatim excerpt of the Zoom chat log during a networked ensemble experiment.
