1. Introduction
Predator–prey interactions in the fossil record can offer unique and crucial insights into the ecology of extinct organisms and the trophic structure of their ancient ecosystems across deep time. Despite their overall abundance in the Jurassic, the role of ammonites as prey for vertebrate consumers is poorly documented and largely speculative. Although predation on ammonites by both vertebrate and invertebrate predators did occur (Lehman, Reference Lehman1976; Kase et al. Reference Kase, Johnson, Seilacher and Boyce1988; Klompmaker et al. Reference Klompmaker, Waljaard and Fraaije2009; Hoffman & Keup, Reference Hoffman, Keupp, Klug, Korn, de Baets, Kruta and Mapes2015), identifying the perpetrator with certainty is problematical as usually only traces of damaged or fragmented conchs are preserved (Martill, Reference Martill1990). Marine reptiles are often considered as potential ammonite predators (Kauffman & Kesling, Reference Kauffman and Kessling1960; Wetzel, Reference Wetzel1960; Kauffman, Reference Kauffman2004; Hoffman & Keup, Reference Hoffman, Keupp, Klug, Korn, de Baets, Kruta and Mapes2015; Gale et al. Reference Gale, Kennedy and Martill2017), although bony fishes are frequently overlooked in this role. Martill (Reference Martill1990) and Richter (Reference Richter2009) described bite traces indirectly attributed to durophagous actinopterygians on few Middle Jurassic ammonites from England and Germany. However, more direct evidence (e.g. ammonite remains inside a fish’s gut contents) has yet to be described, shedding doubt over the trophic relationship between ammonites and actinopterygian fishes. A single hybodontiform shark tooth found in association with a ‘punctured’ ammonite from the Late Jurassic of France (Vullo, Reference Vullo2011) represents the only direct evidence for a chondrichthyian feeding on ammonites.
Pachycormidae is a diverse Mid- to Late Mesozoic lineage of stem-teleost fishes ranging in size from 0.3 to 15 m (Wenz, Reference Wenz1968; Martill, Reference Martill1988; Liston, Reference Liston, Arratia and Tintori2004; Friedman et al. Reference Friedman, Shimada, Martin, Everhart, Liston, Maltese and Triebold2010). Pachycormid diets and feeding strategies are poorly known: gut contents are known for few taxa and most of these observations are anecdotally based only on a single individual. Early Jurassic genera have been described as teuthophagous (Přikryl et al. Reference Přikryl, Košták, Mazuch and Milkuláš2012; Cooper & Maxwell, Reference Cooper and Maxwell2022) or consuming both soft-bodied coleoids and small actinopterygians (Aldinger, Reference Aldinger1965; Cooper et al. Reference Cooper, Giles, Young and Maxwell2022); gastric contents in Middle and Late Jurassic species consist exclusively of small teleosteans (Vetter, Reference Vetter1881; Viohl, Reference Viohl and Boucot1990; Martill, Reference Martill, Martill and Hudson1991; Lambers, Reference Lambers1992; Liston, Reference Liston, Arratia, Schultze and Wilson2008; Maxwell et al. Reference Maxwell, Lambers, López-Arbarello and Schweigert2020). Ammonites were previously theorized to have contributed to the diets of some pachycormids, although no direct evidence has been published (Wild, Reference Wild1994; Cooper & Maxwell, Reference Cooper and Maxwell2022). Here, we report a specimen of Pachycormus macropterus (de Blainville, Reference Blainville and de Blainville1818) from the Early Jurassic of Germany, representing the first direct evidence of an actinopterygian fish consuming a Mesozoic ammonoid.
2. Materials and methods
Specimen SMNS 52472 was collected in 1977 from the Fischer Quarry in Zell unter Aichelberg, Germany (Fig. 1). It originates from the early Toarcian (Lower Jurassic) Posidonienschiefer Formation (top of bed number ϵII4; exaratum Subzone; Riegraf et al. Reference Riegraf, Werner and Lörcher1984) and is referred to the basal asthenocormine pachycormiform, Pachycormus macropterus (de Blainville, Reference Blainville and de Blainville1818; Lehman, Reference Lehman1949; Mainwaring, Reference Mainwaring1978; Cawley et al. Reference Cawley, Kriwet, Klug and Benton2018; Dobson, Reference Dobson2019; Cooper et al. Reference Cooper, Giles, Young and Maxwell2022).

Figure 1. Locality map and simplified stratigraphic log of the Posidonienschiefer Formation at Holzmaden. (a) Map of Germany with the state of Baden-Württemberg highlighted. (b) Extent of Posidonienschiefer formation (Upper Lias) outcrops in Baden-Württemberg with the area of Holzmaden indicated by a star. (c) Simplified map of the Holzmaden area. A star indicates the collection locality of SMNS 52472. (d) Simplified stratigraphic log of the Posidonienschiefer Formation in the Holzmaden area. Pachycormus macropterus is distributed between beds ϵII3 – ϵIII (serpentinum – bifrons Zones) with the collection horizon of SMNS 52472 indicated. Redrawn and modified from Cooper et al. (Reference Cooper, Giles, Young and Maxwell2022) with biostratigraphy based on Riegraf et al. (Reference Riegraf, Werner and Lörcher1984) and Maxwell et al. (Reference Maxwell, Cooper, Mujal, Miedema, Serafini and Schweigert2022).
The specimen was prepared using the transfer method, whereby the original fossil has been embedded into a foreign slab of Posidonienschiefer matrix for improved stability. The skeleton is well-articulated and almost complete, with a total length of 850 mm (Fig. 2). Only the anal fin, pectoral fins and the rostrodermethmoid region of the skull are missing. A small wedge containing the posterior portion of the dorsal fin has been sculpted from plaster. The skull has been compacted dorsal-right laterally, with the skull roof and upper jaws exposed in dorsal view, the elements of the left side in external view and those of the right side in medial view or covered by the skull roof. The vertebral column and unpaired fins are exposed in left-lateral view.
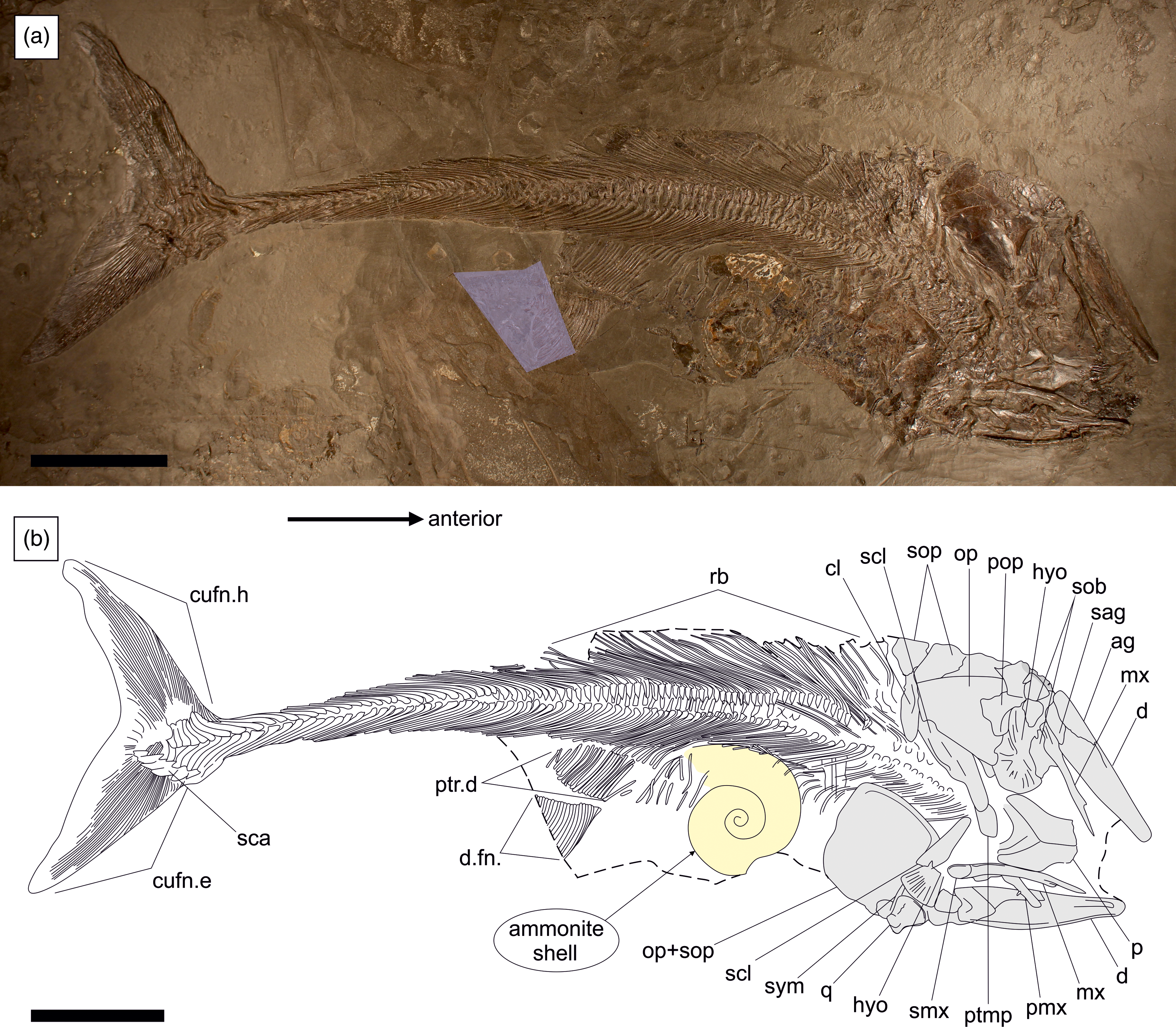
Figure 2. SMNS 52472, Pachycormus macropterus (de Blainville, Reference Blainville and de Blainville1818) preserved in left-dorsolateral view with a large ammonite (cf. Eleganticeras sp.) inside the gut. (a) Overview of specimen with reconstructed sections highlighted. (b) Schematic line drawing. Ag = angular; cl = cleithrum; cufn.e = epaxial lobe of caudal fin; cufn.h = hypaxial lobe of caudal fin; d = dentary; d.fn = dorsal fin; hyo = hyomandibula; mx = maxilla; op = opercle; p = parietal; pmx = premaxilla; pop = preopercle; ptmp = posttemporal; ptr.d = pterygiophores of dorsal fin support; q = quadrate; rb = ribs; sag = surangular; sca = scaly caudal apparatus; scl = supracleithrum; sob = suborbital; sop = subopercle; smx = supramaxilla; sym = symplectic. Scale bar equals 100 mm.
The specimen was examined using a Leica light microscope and photographed with a Nikon Series DMC-FZ72 camera with compact 60X optical macro-lens. Measurements are defined based on Cooper and Maxwell (Reference Cooper and Maxwell2022, suppl. fig. 1), and anatomical nomenclature and homologies for the pachycormiform skeleton follow Mainwaring (Reference Mainwaring1978), Lambers (Reference Lambers1992) and Dobson (Reference Dobson2019). Parameters used to measure the ammonite shell in SMNS 52472 follow Bardin et al. (Reference Bardin, Rouget, Benzaggagh, Fürsich and Cecca2015).
To assess the relationship between maximum prey size and maximum gape size, we estimated maximum gape size using articular width as a proxy (Mihalitsis & Bellwood, Reference Mihalitsis and Bellwood2017). We divided the mandible length (45 mm) of an uncompacted specimen of Pachycormus macropterus (SMNS 4204) by the uncompacted articular width (40 mm) to produce a scaling ratio of 0.88. We then multiplied this scaling ratio by mandible length (measured between the anterior tip of the dentary and the posterior-most margin of the angular) of compacted and/or disarticulated specimens to provide an estimate for maximum gape size. For maximum prey size, we measured the maximum length and maximum depth/width of each prey taxon identified and used published literature for estimations of maximum body sizes where available (e.g. Teudopsis sp. – Doguzhaeva & Mutvei, Reference Doguzhaeva and Mutvei2003).
Institutional abbreviations. HAUFF, Urwelt Museum Hauff, Holzmaden, Germany; IGP, Institute of Geology and Palaeontology, Charles University, Prague, Czech Republic; NHMUK, The Natural History Museum London, London, UK; SMNS, Staatliches Museum für Naturkunde Stuttgart, Stuttgart, Germany.
3. Results
3.a. Placement of the ammonite shell
The ammonite conch (cf. Eleganticeras sp.) measures 95 mm in maximum width (Bardin et al. Reference Bardin, Rouget, Benzaggagh, Fürsich and Cecca2015). It is positioned just dorsal to the vertebral column of the fish, midway between the skull and dorsal fin, with the aperture of the shell orientated dorsoposterior to the axial skeleton. During compaction, the venter of the body chamber pushed into and deformed the distal margins of the neural spines anterior to the dorsal fin, causing several of them to break, with the fragments contorted along the ammonite shell margin. At least ten undeformed neural spines are visible underneath the ammonite’s body chamber in epirelief. Minor preparation-related breakage towards the rear of the body chamber exposes the distal end of a neural spine underneath the shell, and underneath the bone are the right-lateral body scales. The two rows of supraneurals are perfectly articulated dorsal to the neurals. Although in articulation, the second row of supraneurals overlies the upper (external) surface of the shell. The external face of the ammonite is also partially overlain by the squamation of the fish of the dorsal-left lateral side (Fig. 3). The presence of bones and scales both above and below the shell, in combination with the degree of articulation of the Pachycormus skeleton, confirms that the ammonite is situated inside of the fish’s body, thus excluding chance association or taphonomic distortion as explanations for its inclusion in the gut. A hypothetical scenario whereby the fish landed on top of or the shell was somehow washed inside of a partially rotting fish carcass lying on the sea floor is unmerited, especially when considering the preservation quality of the aragonite (see section 3.b.).

Figure 3. Details of prey ammonite inside the gut of SMNS 52472. (a) Photograph of ammonite conch preserved inside the digestive tract of Pachycormus. (b) Line drawing illustrating the ammonite and overlying elements of the skeleton. Bones represented with dashed lines are underneath the ammonite; those with solid lines are either beside or overlaying the shell. Notice the breakage of the neurals (nur) and the overlying second row of supraneurals (snur(2)); the first row supraneurals (snur(1)) are underneath the ammonite. Abdominal scales cover the external surface of both the bones and the ammonite shell. Scale bar equals 50 mm.
The placement of the shell is interpreted to result from compaction of the skeleton in a left-dorsolateral direction. The fish carcass, lying dorsoventrally in the sediment with a slight inclination towards the right side, upon burial became compacted, crushing the head dorsoventrally but the postcranium laterodorsally. The tough exterior and structural rigidity of the ammonite conch in the gut, relative to the softer and more ductile nature of the fish’s abdominal cavity, caused the shell to shift in the same direction as the axial skeleton, such that it partially penetrated between the unmineralized neural spine – supraneural articulation (SupFig. S1). This would somewhat account for why the supraneurals are perfectly articulated on the top side, but some of the neural spines underneath the shell have fractured with this passive movement of the shell. The presence of telescope fractures cross-cutting the oxycone shell (Fig. 3b) indicates that this movement occurred early in the compaction process (sensu Seilacher et al. Reference Seilacher, Andalib, Dietl and Gocht1976). The ammonite shell was therefore irrefutably ingested by the Pachycormus individual while the fish was alive and strongly supports a true ammonite–actinopterygian trophic relationship.
3.b. Ammonite preservation and timing of ingestion
Ammonite preservation in the Posidonienschiefer Formation is notoriously unfavourable. Fossil conchs are flattened and largely dissolved, leaving behind only the semi-transparent periostracal films and rare minute patches of original and partially replaced shell fragments (Seilacher et al. Reference Seilacher, Andalib, Dietl and Gocht1976; Kauffman, Reference Kauffman1978; Schmid-Röhl & Röhl, Reference Schmid-Röhl and Röhl2003; Muscente et al. Reference Muscente, Vinnes, Sinha, Schiffbauer, Maxwell, Schweigert and Martindale2023). The ammonite associated with SMNS 52472 represents a significant exception to this rule, as it preserves large areas of the original shell and retains a nacreous lustre (Fig. 3), allowing us to infer the presence of aragonite (Muscente et al. Reference Muscente, Vinnes, Sinha, Schiffbauer, Maxwell, Schweigert and Martindale2023). This is the only known ammonite from the formation to retain this degree of aragonitic preservation, likely the result of the conch being sealed within the fish’s gut prior to burial and thereby protecting the original shell material from external chemical dissolution. All of the chance-associated ammonite shells in the surrounding matrix have been extensively dissolved, with only their inorganic periostracum preserved.
The periostracum, external to the aragonite shell, is a strongly contrasting orange-brown colour. The incompletely preserved aragonitic layer is beige-white to cream grey and is more extensively preserved on the body chamber and the dorsoanterior portion of the phragmocone. This layer is rough and patchy, with external surfaces showing small angular pits coupled with cross-cutting scratches and grooves. This damage is not the result of preparation, since equivalent traces are not seen in either the periostracum or surrounding matrix; instead, they most plausibly represent etching traces from the fish’s stomach acid. The limited degree of etching suggests that the shell underwent only a very brief period of digestion. The ammonite must have been ingested immediately prior to the fish’s death, perhaps within only hours or minutes depending on the corrosive properties in the gastric acids of Pachycormus.
Aptychi are absent in SMNS 52472, indicating that the shell was likely vacant at the time of ingestion. The ammonite was likely in an advanced stage of decomposition at the point of ingestion by Pachycormus, with the air-filled chambers in the phragmocone maintaining the shell’s buoyancy in the water column post-mortem. Alternatively, the aptychi may have dislodged while inside of the gut but are now entirely overlain by the bones in the axial skeleton. However, given that the ammonite was ingested shortly before the fish’s death, the likelihood of the soft tissues slipping out of the shell while inside of the gut is unlikely.
3.c. Dietary ecology of Pachycormus spp
Preserved gut contents are rare in Pachycormus, particularly among larger individuals despite the presence of amorphous phosphatic cololites (Table 1). The most readily observed constituent of the gut is the teudopseid coleoid, Teudopsis sp. due to its well-mineralized and diagnostic gladii (e.g. Doguzhaeva & Mutvei, Reference Doguzhaeva and Mutvei2003), whereas only a single specimen preserves remains of the loligosepiid coleoid Loligosepia aalensis (Hauff-D-Pac-1; SupFig. S2). Loligosepia and Teudopsis do not possess hooklets (Fuchs & Weis, Reference Fuchs and Weis2008; Fuchs et al. Reference Fuchs, Keupp and Schweigert2013) meaning that once the delicate mantle and gladius have been digested, there are no remaining recognizable elements left to pass into the intestine. Examples of gladii and proostraca in the gut of Pachycormus therefore represent rare final meals, ingested shortly before death. Abundant in a few specimens are isolated hooklets of the belemnoid coleoid Clarkeiteuthis conocauda, which, due to their durable inorganic nature, are more resistant to the effects of gastric corrosion allowing them to form accumulations in the gut, even after the rest of the animal has been fully digested (Přikryl et al. Reference Přikryl, Košták, Mazuch and Milkuláš2012). The large ammonite shell inside the gut of SMNS 52472 is therefore a unique occurrence.
Table 1. Gut contents in Pachycormus spp.

HL, head length; ML, mandible length; SL, standard length. ‘?’ denotes an unobtainable measurement, whereas ‘n/a’ is used for missing anatomy and/or unspecified data from previous studies.
Piscivory is rare in Pachycormus. Prey fishes predominantly comprise the small teleost Leptolepis spp. (Table 1). Two Pachycormus specimens from Normandy (NHMUK PV OR 32425; NHMUK PV OR 32427) preserve a small conspecific inside of the gut (Woodward, Reference Woodward1895), suggesting opportunistic cannibalism (Table 1). The majority of prey fishes were ingested head-first (SupFig. S3D), whereas coleoid prey were ingested longitudinally, always posterior-first.
3.d. Gape size in Pachycormus
Piscivorous fishes are often considered to be gape-limited, implying that mouth size is the main factor limiting prey size. We defined maximum gape in bony fishes as the articular width between the two opposing rami of the lower jaw (Mihalitsis & Bellwood, Reference Mihalitsis and Bellwood2017), and maximum prey size as the maximum body depth of the largest prey ingested by a successful feed, standardized to the predator’s standard length (Table 1).
Maximum gape size ranges in Pachycormus from 26 mm (SMNS 87771 = 160 mm SL) to over 126 mm (SMNS 18189 = 840 mm SL) (Table 1), increasing with weak negative allometry (log gape = 0.95(log SL) −0.67). The estimated gape of SMNS 52472 (111 mm ML) is greater than the maximum diameter of the ingested ammonite shell (95 mm), confirming that this fish was physically capable of swallowing the shell whole in a horizontal orientation. Given that the ammonite is only slightly smaller than the maximum gape of the fish, we infer that the shell was intentionally ingested, rather than as bycatch, because Pachycormus had to physically expand its mouth almost fully in order to capture and process this unusually large item. An alternative hypothesis whereby the shell was accidentally swallowed during attempted capture of a different prey item is highly unlikely.
4. Discussion
4.a. Dietary ontogeny in Pachycormus
Piscivory appears to be restricted to smaller Pachycormus individuals; prey fish are rarely observed in specimens larger than 270 mm SL (Table 1). Larger individuals solely display a teuthophagous diet, with gut contents composed of a variety of soft-bodied coleoids (Table 1) and an apparent preference for vampyropods is consistent with the observed rarity of gut contents in fishes of this size. The shift in dietary preference from piscivory to teuthophagy in Pachycormus is correlated with increasing gape. Specimens of Leptolepis spp. have a maximum body depth of 26 mm (SC pers. obs.), meaning that even the smallest individuals of Pachycormus were capable of swallowing large (≤160 mm TL) Leptolepis. By contrast, vampyropod and Loligosepia coleoids are generally much wider (≥45 mm mantle width) and therefore hypothetically were only accessible to larger individuals of Pachycormus. Almost all examples of coleoid prey show the gladii orientated longitudinally in the gut and always posterior-first. In these cases, the coleoids’ total length exceeds the maximum gape size potential, meaning that the prey, once captured, could only be ingested longitudinally in order to fit through the mouth. Our data suggest that Pachycormus was an obligate piscivore as a juvenile and became facultatively teuthophagous at standard lengths ≥300 mm. Unlike Saurostomus and Germanostomus, which frequently consumed loligosepiid and belemnoid coleoids (Cooper & Maxwell, Reference Cooper and Maxwell2022; Cooper et al. Reference Cooper, Giles, Young and Maxwell2022), teudopseid vampyropods were more common prey items for Pachycormus (Table 1). The ammonite in the gut of SMNS 52472 represents a unique exception to the soft-bodied coleoid diet of similar-sized Pachycormus individuals.
4.b. Palaeoecological significance and evidence of fatal consumption
Osteichthyian bite traces on ammonites have not been reported in the Posidonienschiefer. Occasionally, small actinopterygians (Dapedium and Pholidophorus) are found preserved trapped inside of an ammonite body chamber (Jäger, Reference Jäger1990; Fraaye & Jäger, Reference Fraaye and Jäger1995); although whether these fishes were scavenging on the rotting ammonite, feeding on smaller scavengers or seeking refuge remains undetermined (Fraaye & Jäger, Reference Fraaye and Jäger1995; Jäger, Reference Jäger2005). Wild (Reference Wild1994) reported alleged ammonite remains inside the gut of Saurostomus esocinus; however, these remains represent fragments of the diplobeliid squid Clarkeiteuthis conocauda (Cooper & Maxwell, Reference Cooper and Maxwell2022). An immature S. esocinus with a small (2 mm diameter) ammonite larva mixed among larger prey likely represents unintentional bycatch, rather than evidence for Saurostomus feeding on zooplankton (Cooper & Maxwell, Reference Cooper and Maxwell2022; fig. 13). A similar unintentional ingestion scenario for SMNS 52472 is implausible, given the diameter of the ammonite relative to the predator’s gape size; therefore, SMNS 52472 represents the first irrefutable direct evidence of an actinopterygian intentionally consuming an ammonite.
The absence of aptychi indicates that the ammonite associated with SMNS 52472 was likely in an advanced stage of decomposition or was empty at the moment of ingestion, with the air-filled chambers in the phragmocone maintaining the buoyancy of the shell in the water column. The motion of the floating shell, perhaps mimicking a struggling coleoid or smaller fish, as well as the glimmering nacreous lustre on the externa may had attracted the predator’s attention. Alternatively, the Pachycormus individual may had been scavenging the partial rotting ammonite creature when the shell became caught in the mouth and then swallowed. In this scenario, we surmise that Pachycormus did not actively hunt ammonites, rather opportunistically scavenged them. Regardless of the method of consumption, SMNS 52472 evidently represents a case of accidental ingestion of an ammonite conch.
Ingestion of the ammonite shell was undoubtedly fatal. The large size of the conch relative to the stomach, combined with the shell’s rigid and brittle structure, would have exerted physiological constraints on the fish’s ability to successfully process the ammonite meal. The morphology of the ammonite is highly divergent from the softer, more ductile properties of prey fish and soft-bodied coleoids, which would have been easily compressed by muscular contraction and moved through the digestive tract during peristalsis. As a result, the shell may have become stuck in the foregut, causing the fish the choke and drown, or if passed successfully into the gut, the large shell likely blocked the narrow entrance to the midgut intestine (see Cooper et al. Reference Cooper, Giles, Young and Maxwell2022, figs 9, 10), resulting in death by congestion or even possibly perforating the stomach wall resulting in internal bleeding. The skeleton shows no evidence of external traumas such as bite traces; the short time frame between ammonite ingestion and death of the consumer – as indicated by the shell’s remarkable preservation – strongly implies that the ammonite inside of SMNS 52472 was directly responsible for the fish’s death.
5. Conclusions
SMNS 52472 represents the first direct evidence of an actinopterygian fish consuming an ammonite. The large size of the prey suggests that either (1) the shell was attacked as result of mistaken identity or (2) the fish was feeding on the rotting ammonite creature when the shell was accidentally swallowed. The ingestion of the ammonite was almost certainly fatal with SMNS 52472 representing the first documented case for a ‘fatal last meal’ in a pachycormid fish. A review of gut contents in Pachycormus reveals a trend of dietary shift with increased ontogeny, with juveniles being obligate piscivores eventually shifting to facultative teuthophagy in adults. Previous hypotheses on the potential for ammonites to contribute to the diets of bony fishes are strongly supported with new direct evidence. The significance of which reveals a uniquely crucial insight into the recondite and complex trophic relationship between actinopterygian fishes and ammonoid cephalopods in the Mesozoic.
Supplementary material
The supplementary material for this article can be found at [https://doi.org/10.1017/S0016756823000456].
Acknowledgements
The authors thank Anna Krahl (GPIT), Emma Bernard (NHMUK), Rolf and Franziska Hauff (HAUFF), Adriana López-Arbarello (BSPG), Julien Kimmig and Dennis Grabow (SMNK) for collections access. Jack Norton (University of Portsmouth) accompanied SLAC on fieldwork in Ohmden. We thank the reviewers (David Martill, Adriana López-Arbarello and third anonymous individual) for their helpful and constructive comments which greatly improved the quality of the final manuscript.
Financial support
This research was supported with funding by DFG grant MA 4693/7-1 to Erin Maxwell.
Competing interests
The authors declare none.