Background
Patients with aneurysmal subarachnoid hemorrhage (aSAH) are at risk of developing vasospasm and delayed cerebral ischemia. Reference Lang, Diehl and Mehdorn1–Reference Kumar, Shahripour and Harrigan4 Standard measurements of cerebral blood flow velocity with Transcranial Doppler (TCD) struggle to predict these complications, likely because standard TCD offers only a snapshot of a dynamic disease process. Increasing attention has been directed toward investigating the role of variability in cerebral blood flow velocity as a predictor of vasospasm in aSAH. Reference Soehle, Czosnyka, Chatfield, Hoeft and Peña5 Both linear and non-linear descriptors of variability have been investigated in this context. Reference Soehle, Czosnyka, Chatfield, Hoeft and Peña5 Linear metrics, such as standard deviations, averages, and total power spectra values, have shown limited predictive accuracy, likely as a consequence of the multiple factors that influence cerebral perfusion over time, including systemic blood pressure, respiratory rate, arterial content of carbon dioxide (PCO2), cardiac output, hematocrit, and cerebral metabolism. Reference Panerai, Carey and Potter6-Reference Rickards and Tzen8 Non-linear descriptors such as the use of fractal and chaotic characteristics of cerebral blood flow velocity may better predict vasospasm Reference Panerai7 though it is not clear which metrics might have the best predictive capabilities. Reference Soehle, Czosnyka, Chatfield, Hoeft and Peña5,Reference Keunen, Pijlman, Visée, Vliegen, Tavy and Stam9–Reference Ozturk, Arslan and Hardalac11 Moreover, it remains unclear if it is feasible to perform TCD recordings that would reliably generate linear and non-linear estimates of variability under routine clinical conditions. In this feasibility study, we sought to determine whether we could perform adequate TCD recordings to assess a series of linear and non-linear metrics of cerebral blood flow variability in patients with aSAH as well as in healthy controls. We also explored which metrics might have the ability to assess differences in variability between aSAH patients and healthy controls, and among patients who would go on to develop vasospasm and those who would not. We selected a comprehensive set of variability descriptors (see Supplementary Table S-1) in the time and frequency domains as well as non-linear indices of fractality and deterministic chaos that have been investigated previously. Reference Soehle, Czosnyka, Chatfield, Hoeft and Peña5,Reference Panerai7,Reference Van den Brule, Vinke, Van Loon, van der Hoeven and Hoedemaekers12–Reference Keunen, Vliegen, Stam and Tavy15
Methods
The study was approved by The Ottawa Health Science Network Research Ethics Board and conforms to the principles outlined in the Declaration of Helsinki. Our study was cross sectional, and it was guided according to the STROBE statement on observational studies. Written informed consent was obtained from participants or their substitute decision-makers.
Study Planning
We initially reviewed the available literature and identified a set of linear and non-linear metrics of variability (see Supplementary Table S-1). Reference Soehle, Czosnyka, Chatfield, Hoeft and Peña5,Reference Keunen, Vliegen, Stam and Tavy15–Reference Zhang, Zuckerman and Levine17 Linear analyses included traditional time-domain techniques such as the calculation of standard deviations and coefficient of variations. Reference Soehle, Czosnyka, Chatfield, Hoeft and Peña5,Reference Rickards and Tzen8,Reference Van den Brule, Vinke, Van Loon, van der Hoeven and Hoedemaekers12,Reference Seely and Macklem18 Non-linear methods of analysis included Fractality measures [Hurst coefficient, Reference Soehle, Czosnyka, Chatfield, Hoeft and Peña5,Reference Panerai7 Fractal dimension, Reference Beckers, Verheyden, Couckuyt and Aubert13,Reference Voss, Schulz, Schroeder, Baumert and Caminal14 and Higuchi exponent Reference Higuchi19 ] and descriptors of deterministic chaos [correlation dimension (D2) Reference Keunen, Pijlman, Visée, Vliegen, Tavy and Stam9,Reference Liau, Yeh, Chiu and Tsai20–Reference Baselli, Cerutti, Porta and Signorini22 and maximum Lyapunov exponent (λ)]. Reference Ozturk and Arslan10,Reference Ozturk, Arslan and Hardalac11,Reference Keunen, Vliegen, Stam and Tavy15 In the frequency domain, we sought to calculate the power spectrum for the very-low-frequency (0.003–0.040 Hz), low-frequency (0.040–0.150 Hz), and high-frequency (0.15–0.4 Hz) ranges derived from the mean flow velocity, pulsatility index and mean systemic blood pressure. Reference Panerai7,Reference Rickards and Tzen8 Four durations of recordings (25, 30, 40, and 45 minutes) were tested by two of the authors (RAR, CLH) to determine which would provide sufficient data to calculate the metrics of interest. It was concluded that a 40-minute recording was the minimal recording that would provide the necessary data.
Population
aSAH group
We enrolled consecutive adult patients (>18 years) with a clinical and radiographic Reference Fisher, Kistler and Davis23 diagnosis of aSAH (Fisher scale ≥2) within the first 5 days of their ictus and who were admitted to the Intensive Care Unit (ICU) of the Civic Campus, The Ottawa Hospital, between January and November 2018. Patients with traumatic aSAH or with other unrelated central neurologic disorders, active infection or sepsis, additional bleeding disorders, or hemodynamic instability for other reasons were excluded. Patients were treated according to a standard clinical protocol for aSAH with the purpose of maintaining cerebral perfusion pressure and to prevent secondary ischemia related to vasospasm. None of the patients with aSAH were known to have vasospasm at the time of recording. All participants received routine monitoring of vital signs, intensive nursing care, and routine assessment of their neurologic status including the Glasgow Coma Scale (GCS) at admission and during their stay in the ICU. Reference Teasdale and Jennett24 Aneurysms were clipped surgically or coiled endovascularly according to the preferences of the treating team.
Healthy Controls
To determine whether these metrics of variability might have the ability to distinguish deviations from normality in aSAH patients, a healthy population was identified as a control group by assessing their wellness status using a self-reporting health questionnaire. Thus, staff volunteers from our institution were invited to participate as control subjects. Two control groups were planned a priori. The first group included students and young staff under 40 years of age (young healthy controls), and the second group comprised of older healthy subjects who were matched according to their age with the aSAH group (age-matched healthy controls).
Monitoring Procedure
Two 2 MHz pulse-wave Doppler probes were secured on the temporal area to simultaneously monitor cerebral blood flow velocity from the right and left middle cerebral arteries (MCAs) using the ST3 digital Transcranial Doppler (TCD) system (Model PMD150; Spencer Technologies, Redmond, WA). The envelopes of the Doppler spectra waveform corresponding to the peak, mean, and diastolic flow velocities were exported from the TCD system and analyzed with a custom-designed software program (CIMVA executable, Version 4.6, OHRI Dynamic Analysis Laboratory). Systemic blood pressure was also continuously recorded from the radial artery in all aSAH participants. In all age-matched controls and in two of the young controls, non-invasive continuous recording of blood pressure from the finger was obtained by photoplethysmography Reference Imholz, Wieling, van Montfrans and Wesseling25 using a Finometer (Finapres Medical Systems, Enschede, The Netherlands). The Finometer was calibrated using upper arm return-to-flow systolic pressure detection and physical criteria following brachial artery pressure reconstruction. Reference Bos, van Goudoever, van Montfrans, van den Meiracker and Wesseling26 Heart rate from an electrocardiogram was monitored in all participants. Continuous TCD recordings lasted approximately 40 minutes. Measurements for aSAH patients were performed at the bedside while for controls, recordings were performed in an isolated room, in the supine position, with the head elevated to 30°, and with the subjects awake or in physiological light sleep. All TCD recordings in the young control group were performed in the morning (10:00–11:00 am). In the age-matched controls, however, we accommodated morning (10:00 am to noon) and afternoon sessions (13:00–15:00 hours). No complications were identified during any of the recording sessions.
Follow-Up
Determination of vasospasm was extracted from the patient chart at the time of discharge, and the assessor for this outcome was blinded to the study results. We relied upon the indices used by the clinical teams: namely, evidence of cerebral vasospasm determined by CT/MRI angiography (narrowing of the vascular segment of at least one-third of its diameter) Reference Anderson, Ashforth, Steinke and Findlay27 or by TCD examination (mean flow velocity >120 cm/s). Reference Aaslid28 The angiographic definition of vessel narrowing sufficient to be considered cerebral vasospasm was established without consideration of the clinical manifestations of delayed cerebral ischemia. Reference Vergouwen, Vermeulen and van Gijn29 Data collected for the purposes of this feasibility study were not made available to treating physicians and, therefore, did not impact any diagnostic or therapeutic decision-making.
Outcome Measures
Primary feasibility outcomes were the proportion of completed TCD recordings in both aSAH patients and healthy controls as well as the proportion of TCD recordings that provided acceptable time-series estimates to calculate linear and non-linear variability metrics. Secondarily, we determined differences in the proposed set of linear and non-linear indices of variability for the mean blood flow velocity, mean systemic blood pressure, and TCD pulsatility index between the aSAH group (including those with and without vasospasm) and the control groups. In calculating these between-group differences, we hoped to identify the best indices of variability that would discriminate between these two populations.
Data Processing and Analysis
All TCD and mean arterial pressure waveforms were visually inspected for artifacts. Only artifact-free data were included in the analyses. Data analysis was performed by an independent observer who was not aware of the clinical characteristics of aSAH participants. Separate time-averaged values for all indices of variability were obtained from a minimum of three artifact-free overlapping windows with 1800 beats each. From this analysis, we calculated separate variability indices for the cerebral blood flow velocity, pulsatility index, and mean systemic blood pressure (see Supplementary Table S-1). The pulsatility index was calculated as the difference between the systolic and diastolic flow velocity divided by the mean flow velocity. Reference Gosling and King30
Statistical Analysis
Data are reported as means, standard deviations, or their 95% confidence intervals (95% CI). Discrete variables are expressed in absolute values and percentages. To describe each population, variability data were averaged across patients. Due to the feasibility nature of our primary outcome, expected small sample size, and exploratory character of the between-group differences, we did not attempt to perform any statistical analyses, and all comparative observations were reported descriptively.
Results
Demographics and Clinical Data
We included eight patients with aSAH (age: 58 ± 10 years; two females; range: 44–74 years), eight young healthy controls (age: 26 ± 5 years; four females; range: 21–31 years), and eight age-matched healthy controls (age: 57 ± 10; one female; range: 40–72 years). In the aSAH group, the mean GCS at admission was 10 ± 4, and the average time from admission to the TCD examination was 4 ± 1 days. Of the patients with aSAH, three developed cerebral vasospasm according to our criteria. At the time of recording, none of the aSAH patients had a definitive clinical diagnosis of vasospasm, and the interval between the TCD examination and a confirmed diagnosis varied from 48 to 72 hours. One patient died of heart failure; most of the survivors except one were discharged with at least one neurologic sequela (Table 1).
Table 1: Demographics, clinical features, and evolution of participants in the aSAH group
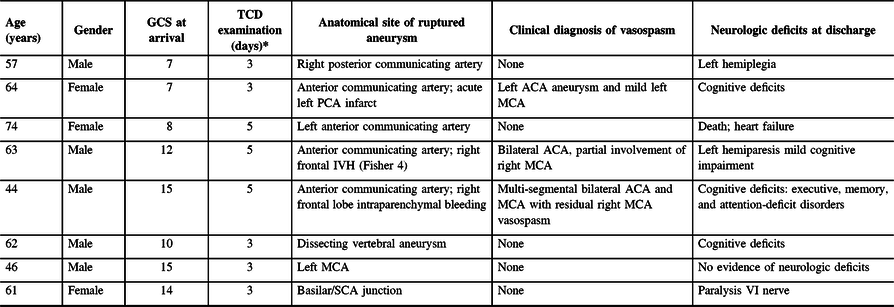
*Date of examination: in days relative to the time of admission; aSAH = aneurysmal Subarachnoid Hemorrhage; GCS = Glasgow Coma Scale score; PCA = posterior cerebral artery; ACA = anterior cerebral artery; IVH = Intraventricular hemorrhage; MCA = middle cerebral artery; SCA = superior cerebellar artery; TCD = Transcranial Doppler.
Feasibility Outcomes
Bilateral extended TCD recordings were successfully completed in all aSAH patients and control subjects. Consent was provided by a substitute decision-maker in all patients, and by the participant for healthy controls. Two eligible patients declined enrollment. No enrolled subject or patient sought to opt out of the study. The average duration for the recording session in the aSAH, age-matched, and young control groups was 40 ± 6, 44 ± 3, and 41 ± 2 minutes, respectively. All individual TCD recordings provided at least three artifact-free overlapping windows of 1800 beats, which were sufficient to calculate reliable linear and non-linear metrics of variability for every participant.
Exploratory Outcomes
Among the metrics assessed, the correlation dimension (Table 2), an indicator of the complexity of physiologic signals, was higher in aSAH patients than in both control groups. aSAH patients also showed higher pulsatility indices, greater high-frequency spectral power, and decreased very-low-frequency spectral power in the mean blood flow velocity relative to healthy controls (see Table 2). Variability metrics for the pulsatility index did not show noticeable differences among the three groups (Supplementary Table S-2). aSAH patients had higher mean systemic blood pressures, low spectral power in the very-low frequencies, and reduced fractal dimension in the systemic blood pressure compared to age-matched controls (Table 3). aSAH patients who developed vasospasm showed higher mean MCA blood flow velocities, smaller pulsatility indices, and lower coefficient of variations in the MCA mean blood flow velocity compared to those who did not develop vasospasm (Table 4). Finally, there were no observable differences in variability between the control groups that would suggest any age-related effects (see Table 2).
Table 2: Linear and non-linear indices of variability for the mean CBFV in the aneurysmal SAH group and in the two healthy control groups. Data represent combined values from the right and left MCAs for each patient. Values are reported as the means and their 95% confidence intervals

CBFV = cerebral blood flow velocity; MCA = middle cerebral artery; SAH = subarachnoid hemorrhage; **Very-low-frequency band: 0.003–0.040 Hz; ***Low-frequency band: 0.040–0.15 Hz; ****High-frequency band: 0.15–0.40 Hz.
Table 3: Linear and non-linear indices of variability for the mean arterial blood pressure in the aSAH group and the age-matched healthy control group. Values are reported as the means and their 95% confidence interval

† Mean arterial blood pressure recorded from the arterial line; *Blood pressure recordings were performed from the middle finger using the photoplethysmography Finapres technology; **Very-low-frequency band: 0.003–0.040 Hz; ***Low-frequency band: 0.040–0.15 Hz; ****High-frequency band: 0.15–0.40 Hz. aSAH = aneurysmal subarachnoid hemorrhage.
Table 4: Linear and non-linear variability indices for the mean cerebral blood flow velocity and mean arterial blood pressure between patients with aSAH who developed vasospasm compared to those without vasospasm. Data for the blood flow velocity in patients without vasospasm represent combined values from the right and left MCAs for each patient. In those with vasospasm, data values are separated according to the ipsilateral or contralateral side of vasospasm. Values are reported as mean ± SD

aSAH: aneurysmal subarachnoid hemorrhage; MCA: middle cerebral artery; **Very-low-frequency band: 0.003–0.040 Hz; ***Low-frequency band: 0.040–0.15 Hz; ****High-frequency band: 0.15–0.40 Hz; n/a = not applicable; &relative to the side of vasospasm.
Discussion
Our findings indicate that it is feasible to perform a 40-minute TCD recording to obtain linear and non-linear metrics of variability from cerebral blood flow velocity in patients with aSAH and healthy controls. Our exploratory analysis suggested that several parameters including the correlation dimension, pulsatility index, the spectral power of the very-low and high-frequency bands, and the coefficient of variation may be helpful to differentiate patients with aSAH and healthy controls and between those with and without vasospasm. A potential explanation for these findings, if they are confirmed in larger studies, is that the complexity of the system that regulates cerebral blood flow may be greater in patients with aSAH than in healthy individuals, and that the progression to cerebral vasospasm diminishes this complexity by decreasing the variability of cerebral blood flow. Reference Soehle, Czosnyka, Chatfield, Hoeft and Peña5,Reference Van den Brule, Vinke, Van Loon, van der Hoeven and Hoedemaekers12,Reference Liau, Yeh, Chiu and Tsai20 Moreover, aSAH patients showed reduced spectral power in the very-low-frequency area for both mean systemic blood pressure and mean cerebral blood flow velocity compared to healthy controls, though more so in systemic blood pressure than in cerebral blood flow velocity. This finding suggests that variability in cerebral blood flow in the very-low-frequency range may be partly dependent on variability in systemic blood pressure. Reference Zhang, Zuckerman and Levine17,Reference Zhang, Zuckerman and Levine31,Reference Tzeng and MacRae32 Since the very-low frequencies represent the frequency range where cerebral autoregulation is expected to be most active, Reference Tzeng and MacRae32 the dependence of cerebral blood flow on changes in systemic pressure could explain the vulnerability of aSAH patients to large fluctuations in systemic blood pressure.
Methodological Considerations and Limitations
We used high temporal resolution TCD ultrasound to measure beat-to-beat changes in blood flow velocity in the MCA, but this technique is based on measurements of blood flow velocity and not volumetric flow. Reference Panerai7,Reference Liu, Zhu and Hill33,Reference Zweifel, Castellani and Czosnyka34 Although we assumed that the diameter of the MCA remained relatively constant in normal subjects, we cannot ensure the same for aSAH patients due to the use of therapeutic agents aimed to maintain elevated systemic blood pressures. In age-matched controls, we measured changes in systemic blood pressure using finger plethysmography (i.e. Finometer), Reference Imholz, Wieling, van Montfrans and Wesseling25 but this technique may not be the same as that obtained from intra-arterial pressure recordings in our aSAH patients admitted to the ICU. In addition, we recognize that our study was not powered to identify differences in variability indices. As a consequence, all of these results are reported descriptively. Although we recognize that performing 40-minute assessments repeatedly in critically ill patients may pose a logistical challenge under routine clinical conditions, an important consideration for future studies is the reproducibility of these variability metrics on repeated measurements and under controlled conditions. Moreover, several physiological variables that are well known to affect cerebral blood flow velocity, such as respiratory rate, hematocrit, PCO2, metabolic factors, and cardiac output, Reference Panerai7,Reference Rickards and Tzen8,Reference Zhang, Zuckerman and Levine31 were not controlled in our study. Our decision to choose an age-matched healthy population as control group was based on our primary objective of feasibility to determine whether we would be able to provide adequate linear and non-linear metrics of variability. Given the known association of smoking and hypertension with aSAH, Reference Okamoto, Horisawa and Ohno35 an additional control group with these risk factors would have been clinically relevant as well. Despite our study identified differences in variability between young healthy males and females (see Supplementary Table S-3), sex-related effects were not accounted for in aSAH patients. We recognize that the choice of angiography as “gold standard” and our definition for the determination of cerebral artery vasospasm may be a limitation. The use of a different definition criterion or “gold standard” test could have modified the relationship between variability indices and the diagnosis of cerebral vasospasm in our patient population. Finally, the lack of a formal clinical assessment and/or brain imaging studies at the time of discharge precludes associating these indices of variability with clinical outcomes.
Conclusions
In summary, we determined that it was feasible to conduct extended TCD recordings for linear and non-linear metrics of cerebral blood flow velocity in healthy controls and patients with aSAH. We were able to measure non-linear metrics, and identified a few specific measures (correlation dimension, coefficient of variation, pulsatility index, very-low and high-frequency spectral power) that are of interest for future studies as potential predictors of vasospasm in patients with aSAH. Further studies are necessary to confirm whether these metrics are reproducible and if any true relationship exists between variations in the complexity of the cerebral blood flow velocity of aSAH patients and their progression into vasospasm and/or poor clinical outcomes. If effective, sequential measurements of the beat-to-beat temporal variability in cerebral blood flow velocity and systemic blood pressure during relatively long periods (i.e. 40 minutes) in patients with aneurysmal SAH may impact risk stratification in patients with aSAH and guide interventions aimed to prevent delayed cerebral ischemia.
Acknowledgments
The study was funded by the Clinical Research Award Program from the Department of Medicine, The Ottawa Hospital, and the University of Ottawa. We acknowledge Dr. Matthew Hogan from the Division of Neurology, The Ottawa Hospital, for his advice and feedback to this project and Robert Meade, from the Human and Environmental Physiology Research Unit of the University of Ottawa for his assistance during finger plethysmography and data extraction. The support from Brigette Gomes, Irene Watpool, Stephanie Belanger, staff nurses, physicians, and coordinators from the ICU and Clinical Investigative Unit, at The Ottawa Hospital, is gratefully appreciated. We thank the voluntary participation of patients, families, and staff.
Statement of Authorship
RAR and MS conceived and designed the study; RAR performed all TCD recordings and drafted the manuscript; CLH contributed to the technical processing and analysis of biological signals; MS and RR obtained funding for this project; SWE and AJES facilitated recruitment and provided clinical input to the project; GPK provided access and guidance on the Finapress technology; RAR and TR developed the analytical plan. All authors participated in the study interpretation, manuscript revisions, and approval of the final version of the manuscript.
Conflict of Interest
AJES and CLH are shareholders of Therapeutic Monitoring Systems Inc. focused on commercialization of variability-derived clinical decision support tools. AJES and CLH are patent holders of variability monitoring and physiological waveform analysis. All other authors (RAR, SWE, TR, GPK, MS) declare that they have no conflict of interest.
Supplementary material
To view supplementary material for this article, please visit https://doi.org/10.1017/cjn.2020.157.