Introduction
To understand the range of male infertility conditions, knowledge of normal male anatomy and its embryology is essential. This chapter will review the major developmental events from fertilization to organization of the germ layers into organ systems. It will review in depth the formation of the male genitourinary system and external genitalia. By laying a strong foundation in embryology and anatomy, providers will have a more thorough understanding of disease states.
Fertilization to Germ Layers
After fertilization of the ovum by sperm, a genetically unique zygote is formed on day two. The zygote undergoes multiple mitotic divisions to create the morula (from the Latin word for mulberry) on day three. The internal cells of the morula are surrounded by trophoblastic cells, which will later become the placenta. By day four, a fluid-filled cavity called the yolk sac, or umbilical vesicle, appears and separates the trophoblast from the developing embryo. The subsequent blastocyst then attaches to the uterine wall on day six, stimulating the trophoblast to rapidly proliferate and differentiate into its two layers: the inner cytotrophoblast and the outer syncytiotrophoblast. The syncytiotrophoblast invades the endometrium, embedding the blastocyst and establishing early uteroplacental circulation, as shown in Fig. 1.1. With implantation, the blastocyst divides into a bilaminar embryonic disc of pluripotent epiblast and hypoblast. Other structures that form during the second week include the amniotic cavity, amnion, umbilical vesicle, and chorionic sac. The syncytiotrophoblasts produce human chorionic gonadotropin (hCG), which maintains the corpus luteum to secrete estrogen and progesterone throughout pregnancy [Reference Moore, Persaud and Torchia1].
Gastrulation takes place during the third week with proliferation and movement of epiblast cells on the embryonic disc, creating the primitive streak and notochord. The bilaminar embryonic disc matures into the three germ layers: the embryonic endoderm, mesoderm, and ectoderm. The primitive streak forms the mesoderm until the fourth week, after which it degenerates and disappears. This mesoderm further differentiates into paired structures straddling the notochord; from medial to lateral, these are the paraxial, intermediate, and lateral mesoderm (Fig. 1.2). The urinary and genital systems largely develop from the intermediate mesoderm.
Around day 16, a caudal outpouching from the umbilical vesicle extends into the connecting stalk of the developing placenta. This diverticulum is known as the allantois. The proximal part of this diverticulum will eventually fibrose and become the adult urachus, or median umbilical ligament. The mesoderm of the allantois expands into blood vessels, which feed the placenta as the umbilical arteries. Late in the fourth week of development, as the embryo folds in the horizontal plane, a longitudinal elevation of the mesoderm appears on either side of the dorsal aorta, called the urogenital ridge. This urogenital ridge gives rise to the nephrogenic cord and the gonadal ridge.
Key Points
Following fertilization, the zygote undergoes multiple mitotic divisions to form the morula on day two, then implants into the endometrium as a blastocyst around day six.
By the third week of development, the embryo has divided into its three main germ layers: the endoderm, mesoderm, and ectoderm.
The majority of the urogenital system is derived from the intermediate mesoderm.
Renal Development
The fetal kidney passes through three phases in establishing its permanent form: the pronephros, mesonephros, and metanephros. The bilateral pronephroi appear early in the fourth week near the developing neck. The pronephric ducts run caudally and open into the cloaca (from the Latin word for sewer), which drains the allantois and hindgut. The majority of the pronephros degenerates as the mesonephros develops at the end of the fourth week. Fig. 1.3 shows the progression of the nephrogenic cord as it passes from the pronephros to the mesonephros and metanephros, with its insertion into the cloaca.
The mesonephros are made up of primitive glomeruli and mesonephric tubules, which drain into the mesonephric ducts. These structures serve as an interim kidney for 4 weeks, until the appearance of the metanephros. Although the majority of the mesonephros also involutes by week 12, the mesonephric duct persists in the male as the appendix of the epididymis, ductus deferens, ejaculatory duct, and seminal vesicles (Table 1.1).
Table 1.1. Derivatives and vestigial remnants of embryonic urogenital structuresa
Embryonic structure | Female | Male |
---|---|---|
Indifferent gonad | Ovary | Testis |
Cortex | Ovarian follicles | Seminiferous tubules |
Medulla | Rete ovarii | Rete testis |
Gubernaculum | Ovarian ligament Round ligament of uterus | Gubernaculum testis |
Mesonephric tubules | Epoophoron | Efferent ductules of testis |
Paroophoron | Paradidymis | |
Mesonephric duct | Appendix vesiculosa | Appendix of epididymis |
Duct of epoophoron | Duct of epididymis | |
Longitudinal duct (Gartner duct) | Ductus deferens | |
Ejaculatory duct and seminal gland | ||
Stalk of ureteric bud | Ureter, pelvis, calices, and collecting tubules | Ureter, pelvis, calices, and collecting tubules |
Paramesonephric duct | Hydatid (of Morgagni) | Appendix of testis |
Uterine tube | ||
Uterus, cervix | ||
Urogenital sinus | Urinary bladder | Urinary bladder |
Urethra | Urethra (except navicular fossa) | |
Vagina | Prostatic utricle | |
Urethral and paraurethral glands | Prostate | |
Greater vestibular glands | Bulbourethral glands | |
Sinus tubercle | Hymen | Seminal colliculus |
Primordial phallus | Clitoris | Penis |
Glans clitoris | Glans penis | |
Corpora cavernosa of clitoris | Corpora cavernosa of penis | |
Bulb of vestibule | Corpus spongiosum of penis | |
Urogenital folds | Labia minora | Ventral aspect of penis |
Labioscrotal swellings | Labia majora | Scrotum |
The metanephros develops in the sacral region in the fifth week, and is functional by weeks 9–10. It consists of the ureteric bud (metanephric diverticulum) and the metanephrogenic blastema. Through reciprocal induction, the ureteric bud and metanephrogenic blastema become the collecting system and nephron, respectively. The ureteric bud begins as a diverticulum of the mesonephric duct, near its insertion into the cloaca. Influenced by the metanephrogenic blastema, the ureteric bud branches repeatedly, growing from the ureter to the renal pelvis, major and minor calyces, and collecting tubules, as shown in Fig. 1.4.
Each tubule induces clusters of mesenchymal cells in the metanephrogenic blastema to differentiate into parts of the nephron: the glomerulus, proximal and distal convoluted tubules, and loop of Henle. Failure of the ureteric bud to associate with the metanephrogenic blastema will result in renal agenesis and often also results in maldevelopment of the other Wolffian duct structures, including the ipsilateral epididymis, vas, seminal vesicle, and ejaculatory duct [Reference Ochsner, Brannan and Goodier2–Reference Holt and Peterson4]. Thus, when unilateral vasal agenesis is encountered, the patient should be referred for renal ultrasound to evaluate the upper tracts. Urine production begins around week 10 of development. The number of glomeruli increases throughout gestation until week 36, so that nephron formation is complete at birth, with each kidney containing 200 000–2 million nephrons [Reference Wein, Kavoussi, Novick, Partin and Peters5].
The paired metanephroi develop within the pelvis, and with abdominal growth, they gradually rise and rotate, until the hilum faces anteromedially and the superior portion of the kidney is in contact with the adrenal glands. The renal blood supply changes with ascent, initially coming from the common iliac arteries, then the abdominal aorta, as it reaches its final position in the retroperitoneum. For this reason, it is not uncommon for kidneys to have accessory renal arteries.
Bladder Development
The cloaca is the early chamber where the hindgut and allantois empty, and it is covered by the cloacal membrane. Two theories exist regarding separation of the cloaca into the dorsal rectum and ventral urogenital sinus. Traditionally, cloacal division was thought to occur between weeks 4 and 7 through a combination of coronal folding and caudal descent of the urorectal septum. Fusion of the urorectal septum with the cloacal membrane was thought to form the perineal body. However, microscopic studies, aided with computer-assisted three-dimensional reconstruction, have suggested that although the distance between the urorectal septum and the cloacal membrane decreases, the two structures do not fuse. Rather, there is flattening, and then apoptosis, of the cloacal membrane, ending in rupture around week 7. This leads to exposure of two surfaces: the anterior urogenital groove and the posterior anal orifice. The area in between, which is the tip of the urorectal septum, becomes the future perineum [Reference Nievelstein, van der Werff, Verbeek, Valk and Vermeij-Keers6, Reference Nebot-Cegarra, Fàbregas and Sánchez-Pérez7]. Early rupture of the cloacal membrane and failure of mesenchymal cell migration between the abdominal ectoderm and the cloacal endoderm result in the bladder exstrophy–epispadias complex.
The bladder forms from the urogenital sinus above the mesonephric (Wolffian) ducts. It begins as a cylindrical tube lined by a single layer of cuboidal cells and is surrounded by loose mesenchymal tissue. From weeks 7 to 12, the surrounding connective tissue condenses and smooth muscle appears. Mature urothelial epithelium is present by week 17. Bladder development is guided by several signaling factors, including Shh, Bmp4, and Fgfr2 [Reference Liaw, Cunha and Shen8]. Around week 10, when urine production begins, the ratio of elastic-to-collagen fibers increases and that of thick-to-thin collagen fibers decreases, improving bladder compliance. The layers of the adult bladder are the urothelium, lamina propria, muscularis mucosa, smooth detrusor muscle, and perivesical fat.
The trigone of the bladder is formed by a combination of smooth muscle from the bladder and incorporation of the ureters from the mesonephric (Wolffian) duct. The mesonephric duct caudal to the take-off of the ureteric bud is known as the common excretory (nephric) ducts, as shown in Fig. 1.5 [Reference Liaw, Cunha and Shen8].
As the common excretory duct contacts the developing bladder, it undergoes apoptosis, separating from the developing ureter, as seen in Fig. 1.5C and D. Through remodeling, expansion, and differentiation, the ureteral orifices move superolaterally and ultimately enter obliquely through the base of the bladder within the trigone (Fig. 1.5D, E) [Reference Viana, Batourina and Huang9]. Adequate detrusor backing is required to prevent the reflux of urine from the bladder to the ureter/renal pelvis as the bladder fills. Vesicoureteral reflux can result in urinary tract infections, renal parenchymal damage, and hypertension. In males, the Wolffian ducts migrate caudally, entering the prostatic urethra at the verumontanum as the ejaculatory ducts (Fig. 1.5E).
External striated muscle fibers appear around week 15 at the caudal end of the urogenital sinus, while internal smooth muscle layers thicken at the bladder neck, laying the groundwork for the future continence mechanism.
Key Points
The fetal kidney passes through three stages before arriving at its adult form: the pronephros, mesonephros, and metanephros. The pronephros and mesonephros largely degrade, but the mesonephric duct persists as Wolffian structures in the male: the ejaculatory duct, vas deferens, epididymal tail, and seminal vesicles. In both male and female fetuses, the mesonephric duct gives off the ureteric bud.
The metanephros forms via reciprocal induction between the ureteric bud and the metanephrogenic blastema, resulting in the collecting system and renal parenchyma, respectively.
The urorectal septum divides the cloaca into the anterior urogenital sinus and posterior rectum (hindgut). Urine production begins around week 10 of gestation, coinciding with bladder development from the urogenital sinus.
Male Gonadal and Genital Duct Development
To review, there are a series of ducts that give rise to the genitourinary system. The pronephric duct is primarily for the rudimentary urinary system and gives rise to the mesonephric duct, which becomes coopted by the genital system in males. The paramesonephric duct is also primarily for the genital system; however, the fates of the mesonephric and paramesonephric ducts are determined by the chromosomal makeup of the fetus. Due to the lack of anti-Müllerian hormone (AMH) in 46XX fetuses, 46XX fetuses retain their paramesonephric ducts while the paramesonephric duct will largely involute in 46XY fetuses.
For both sexes, gonadal development begins in the fifth week with development of the gonadal ridge, a thickened area of mesothelium medial to the mesonephros. Gonadal cords grow into the underlying mesenchyme and are joined by primordial germ cells, which are the earliest undifferentiated sex cells. These cells begin in the yolk sac and migrate along the dorsal mesentery of the hindgut to the gonadal ridge (Fig. 1.6), forming the primitive sex cords.

Fig. 1.6 Primordial germ cells, shown in blue, migrating from the wall of the yolk sac along the dorsal mesentery of the hindgut to the developing mesenchyme of the bilateral genital ridges.
In the seventh week of gestation, this indifferent gonad is identical between 46XX and 47XY embryos, with an epithelial cortex and a mesenchymal medulla. In the 46XX embryo, the cortex of the indifferent gonad will differentiate into an ovary, with subsequent degeneration of the medulla. Conversely, the cortex will regress and the medulla will develop into a testis in the 47XY embryo.
The short arm of the Y chromosome contains the sex-determining region (SRY gene), which produces the SRY protein, also known as testis-determining factor (TDF). Without the SRY gene, the ovary is the default future for the indifferent gonad. The SRY protein induces the cells in the medullary region of the indifferent gonad to form testicular epithelial cords, which will eventually become seminiferous tubules. The primordial germ cells develop into spermatogonia, while the surrounding mesenchyme becomes Sertoli cells. The SRY gene influences seminiferous tubule differentiation through multiple regulating genes: Sox9, Wnt4, Foxl2, Fst, and Rspo1 [Reference Moore, Persaud and Torchia1]. Fig. 1.7 demonstrates the effect of TDF on the indifferent gonad (left), in comparison to the genital course taken without the presence of TDF (right).

Fig. 1.7 (A) Undifferentiated gonads of the 5-week embryo. (B) Testis development is shown on the left. The testis-determining factor on the Y chromosome forces regression of the cortex and development of the medulla into the testicle. The gonadal cords become the rete testis and seminiferous tubules. The bottom left of this image shows the two main cells of the testis: spermatogonia (from the primordial germ cells) and Sertoli cells (from the mesenchyme). Note the lack of the paramesonephros on the left, as this degenerates in the male due to anti-Müllerian hormone. (C) Ovarian development is shown on the right. The primordial germ cells enter the cortical cords, which have developed from the surface epithelium. In contrast to the testis, the medulla of the undifferentiated gonad regresses and the cortex becomes the ovary. Note that the paramesonephros persists and develops into the uterine tube.
In the sixth and seventh weeks, Sertoli cells begin secreting AMH, which suppresses paramesonephric duct development, thereby preventing formation of the uterus, Fallopian tubes, and upper vagina. The mesenchyme separating the seminiferous cords matures into interstitial Leydig cells, which begin producing testosterone in the eighth to ninth weeks under the influence of placental hCG. Later, as the pituitary gland develops, fetal gonadotropins take over androgen production. Testosterone and its more potent derivative, dihydrotestosterone, masculinize the mesonephric duct (Wolffian duct), genital tubercle, and urogenital sinus (as noted in Table 1.1) [Reference Wein, Kavoussi, Novick, Partin and Peters5]. Type 2 5-alpha reductase, secreted by the urogenital sinus and genital tubercle, converts testosterone to dihydrotestosterone, which is essential for normal penile and prostatic development.
Testosterone from fetal Leydig cells stimulates the mesonephric duct to become the Wolffian structures. Although the lumen of the seminiferous tubules will not develop until puberty, the walls of the seminiferous cords are made of spermatogonial stem cells and Sertoli cells. The seminiferous cords condense and extend into the rete testis, which grows into 15–20 mesonephric tubules. These tubules will become the efferent ductules. Distally, the mesonephric duct develops into the duct of the epididymis and, as it becomes enveloped in smooth muscle, thickens into the vas deferens. The caudal end of the mesonephric duct enters medially at the prostatic urethra as the ejaculatory duct. Laterally, the mesonephric duct gives rives to the seminal vesicles.
There is very limited research on seminal vesicle development. Brewster [Reference Brewster10] documented the first evidence of distal bulbous dilations from the mesonephric duct in the 12th week of gestation. Over the following 13 weeks, these dilations grow, forming branching diverticula and folding into concentric circular layers of undifferentiated mesenchyme surrounding an epithelial-lined lumen. The mesenchyme gradually differentiates into the lamina propria, surrounded by the tunica muscularis and adventitia postnatally. Fig. 1.8 shows the progression in seminal vesicle development from 14 weeks’ gestation to a 1-day-old infant to an 18-year-old male. Similar to the prostate, branching morphogenesis of the seminal vesicle is directed by local androgens and fibroblast growth factor, Hedgehog, and transforming growth factor-beta factors [Reference Thomson and Marker11]. The seminal vesicle contributes fructose, ascorbic acid, prostaglandins, and coagulation factors to the male ejaculate [Reference Owen and Katz12].

(A) A 14-week embryo, showing the beginnings of a tubular seminal vesicle, growing from the ampulla. AA, ampulla; ED, ejaculatory duct; P, prostate; PD, paramesonephric duct; SV, seminal vesicle.

(B) Section through the seminal vesicle of a 1-day-old infant. A diverticulum is branching from the main lumen. The lumen contains cellular debris and mucoid substance. BC, basal cells; D, diverticulum; L, lumen. M, smooth muscle; PC, principal cells.

(C) Section through the seminal vesicle of an 18-year-old patient, showing two layers of tunica muscularis and the circumferential nature of the epithelial folding. BC, basal cells; L, lumen; LP, lamina propria; PC, principal cells; TM, tunica muscularis.
The prostate is unique amongst the other male accessory sex glands in that it is derived from the endodermal urogenital sinus. Around weeks 10–12, endodermal outgrowths at the base of the fetal bladder grow into the surrounding mesenchyme. Hox genes control glandular morphogenesis, which results in mesenchymal differentiation into the dense stroma and smooth muscle of the prostate [Reference Huang, Pu, Alam, Birch and Prins13]. Fibroblast growth factor 7, activin A, insulin-like growth factor 1, and bone morphogenetic proteins 4 and 7 have been implicated in the repeated branching of the prostatic ducts from their urethral insertion to the distal ductal tips [Reference Wein, Kavoussi, Novick, Partin and Peters5]. As previously noted, prostate differentiation is guided by fetal androgens, namely dihydrotestosterone, which peak during the second trimester. Prostate development is highly sensitive to varying steroid hormones. In utero exposure to elevated maternal or exogenous estrogens, such as bisphenol A, during prostate development results in decreased prostatic growth and increased risk of prostate cancer in adulthood [Reference Prins, Tang, Belmonte and Ho14]. This is likely secondary to permanent alterations in DNA methylation patterns. With regard to male fertility, the prostate is the main source of acid phosphatase, citric acid, inositol, calcium, zinc, magnesium, and liquefying factors found in the ejaculate [Reference Owen and Katz12].
The verumontanum elongates in a craniocaudal fashion on the dorsal wall of the male urethra, and is the entrance for the terminal mesonephric (Wolffian) ducts and any remnant Müllerian duct, which takes the form of a prostatic utricle. As seen in Fig. 1.9, the verumontanum is an interface between the mesodermal Wolffian duct and the endodermal Müllerian duct [Reference Wein, Kavoussi, Novick, Partin and Peters5]. Similarly, smaller glands derived from the spongy urethra and the adjacent mesenchyme develop into the paired bulbourethral glands. The bulbourethral glands contribute about 5 percent of seminal volume, mostly as a lubricating mucus within the male urethra [Reference Owen and Katz12].

Fig. 1.9 Section of a prebud 9-week human fetal urogenital sinus (UGS) in the region of the verumontanum, a dorsal hillock projecting into the UGS (dotted line). The Wolffian ducts (WDs) flank the Müllerian-derived prostatic utricle (marked), and both open into the UGS at/near the apex of the verumontanum. The section is stained by FOXA1, an endodermal marker.
Testicular development begins in the upper lumbar region near the developing kidney. The germ cells, Sertoli cells, and Leydig cells become surrounded by a thick, fibrous capsule of connective tissue known as the tunica albuginea. As shown in Fig. 1.10A, the testis is supported by a cranial and caudal suspensory ligament, the latter of which becomes the gubernaculum. As the mesonephros and paramesonephros degrade and the fetal intra-abdominal pressure increases, the testes descend caudally along the posterior abdominal wall, typically between weeks 10 and 15. As the testis descends, the cranial suspensory ligament degrades and the gubernaculum thickens (Fig. 1.10B), eventually bulging obliquely beyond the future external inguinal ring and into the scrotum. This process is facilitated by hollowing of the peritoneum, allowing for a ventral evagination of the peritoneal cavity known as the processus vaginalis. As the gubernaculum shortens, pulling the testicle through the inguinal canal into the scrotum, it drags the surrounding processus vaginalis, and thus the abdominal wall layers with it, to surround the testis and spermatic cord: the transversalis fascia, internal oblique fascia, and external oblique fascia. The opening of the transversalis fascia becomes the internal inguinal ring, and the opening of the external oblique the external inguinal ring. The processus vaginalis typically involutes, leaving the testis almost completely surrounded by the tunica vaginalis, except at the entrance of the vas deferens and testicular blood vessels (Fig. 1.10C). A persistent patent processus vaginalis may result in an inguinal hernia and/or a hydrocele [Reference Rafailidis, Varelas, Apostolopoulou and Rafailidis15]. Testicular descent is controlled by fetal androgens and is typically complete by 32 weeks. Cryptorchidism, or undescended testes, is present in up to 9 percent of all newborns, with approximately 40 percent of these testes descending spontaneously by three months of age [Reference Kolon, Herndon and Baker16].
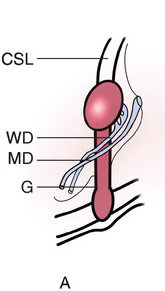
(A) Before descent, the developing testis is held in the urogenital ridge by the cranial suspensory ligament (CSL) and the gubernaculum (G). The adjacent Wolffian duct (WD) forms the epididymis and vas deferens in the male, and the Müllerian duct (MD) forms the uterus and Fallopian tubes in the female.

(B) Around week 15, the transabdominal journey has completed and the testis is held near the future inguinal ring by dilation of the gubernaculum.

(C) The inguinoscrotal phase requires the gubernaculum to elongate into the scrotum, under control of androgens and calcitonin gene-related peptide (CGRP). After migration is complete, the peritoneum of the processus vaginalis (PV) closes and then completely involutes and disappears.
Fig. 1.10 The stages of testicular descent.
Female Gonadal Development
Bladder development above the entry of the mesonephric ducts is the same between male and female fetuses. In the 46XX fetus, the caudal portion of the urogenital sinus develops into the urethra in a similar fashion to the 46XY fetus, although without phallic lengthening. Due to the lack of AMH and testosterone in the female, there is persistence of the paramesonephric ducts and involution of the Wolffian ducts. Maternal estrogen facilitates development of the female sex organs from the paramesonephric ducts. Midline fusion of the paramesonephric ducts, regulated by HoxA10, results in formation of the uterus, as well as of the upper two-thirds of the vagina, broad ligament, rectouterine pouch, and vesicouterine pouch [Reference Moore, Persaud and Torchia1]. The remainder of the paramesonephric duct that does not fuse gives rise to the Fallopian tubes. The distal third of the vagina, as well as the hymen, appears to originate from the urogenital epithelium [Reference Wein, Kavoussi, Novick, Partin and Peters5].
Key Points
The short arm of the Y chromosome contains the SRY gene, which produces TDF. TDF promotes Sertoli cell development and differentiation of the primitive germ cells into spermatogonia. Sertoli cells produce AMH, which prevents formation of the uterus, Fallopian tubes, and upper two-thirds of the vagina from the paramesonephric ducts. Leydig cell production of testosterone influences the mesonephric duct to form the Wolffian structures.
The prostate originates from endodermal outgrowths at the base of the bladder around week 10. This is guided by dihydrotestosterone, which is produced from testosterone via 5-alpha reductase.The testicles form in the abdomen, then descend from weeks 10 to 32 to the scrotum, guided by the gubernaculum (rudder) and processus vaginalis.
Male External Genitalia
The external genitalia develop from weeks 4 to 12 of gestation. They begin as a genital tubercle at the cranial end of the cloacal membrane, flanked on either side by the labioscrotal swellings and urogenital folds. The future of the labioscrotal swellings and urogenital folds in the 46XX and 46XY fetuses are shown in Table 1.1. There are many gene loci, in addition to the SRY gene, needed for successful male differentiation. The urogenital ridge is driven by Wt1, Sf1, and Dax1 [Reference Nachtigal, Hirokawa, Enyeart-VanHouten, Flanagan, Hammer and Ingraham17]. Sertoli cell production of AMH depends on Sox9, FGF9, SRY, Fog2, and Igf1 [Reference Sekido, Bar, Narváez, Penny and Lovell-Badge18–Reference Nef, Verma-Kurvari and Merenmies21]. Finally, Leydig cell production of testosterone is influenced by enzymes produced by the following genes: StAR, Cyp11a1, Cyp17, and 3beta-HSD [Reference Brennan, Tilmann and Capel22]. The urogenital sinus and genital tubercle produce 5-alpha reductase, so that under the influence of testosterone and dihydrotestosterone, the distance between the phallus and the anus increases and the genital tubercle elongates into the primordial phallus and then the penis.
There is some debate regarding male urethral development. The classic fusion theory, proposed by Glenister in 1954, maintains that the urethral plate canalizes on the ventral aspect of the primordial phallus using the urogenital sinus endoderm. The urethral groove folds and fuses to form the spongy urethra, which then meets an ingrowth of the surface ectoderm distally at the glans penis [Reference Glenister23]. However, this theory does not explain the wide variety of anomalies seen in men with hypospadias. Hadidi et al. [Reference Hadidi, Roessler and Coerdt24] conducted a histological study of 15 male fetuses, ranging from 6 to 14 weeks of development, and found the glandular urethra to develop in parallel to the spongy urethra. They observed three stages of development within the spongy urethra: a solid epithelial plate, a deep urethral groove, and a fused urethra. The glandular urethra appeared to have four stages of development: a solid epithelial plate, a blind central canal, a deep glandular groove, and distal migration of the glans/prepuce, which fuses with the distal-most edges of the glandular groove to form the floor of the glandular urethra. The authors did not find evidence of ectodermal ingrowth, as previously suggested by Glenister. Using optical projection tomography, Li et al. [Reference Li, Sinclair and Cao25] proposed a “double zipper theory,” where the first (opening) zipper begins at the scrotal urethral meatus and is characterized by high levels of cellular proliferation within the dorsal aspect of the urethral plate, allowing for urethral plate canalization. The second (closing) zipper is represented by midline fusion of the epithelial edges lining the urethral groove, which also moves distally from the scrotum to the terminal urethral meatus at the mid-glans. The authors noted a lack of caspase 3 within their histological analysis, which would have suggested a role for apoptosis or ectodermal ingrowth, as previously suggested.
The corpus cavernosum and corpus spongiosum develop from the mesenchyme within the primordial phallus. In the male, the paired labioscrotal swellings meet in the midline and fuse to form the scrotum. The future prepuce forms simultaneous with the glandular urethra and is dependent on normal urethral development. This is evidenced by the lack of a normal ventral prepuce in hypospadias and the lack of a normal dorsal prepuce in epispadias.
Key Points
The male urethra likely develops via a “double zipper” process, where the opening zipper is represented by urethral canalization, followed by midline fusion (the closing zipper), moving the meatus from the proximal phallus to the distal glans.
Dihydrotestosterone directs the formation of the external male genitalia from the ambiguous genital tubercle. The urogenital folds and labioscrotal swellings fuse to form the ventral penis and scrotum, respectively. In the female, these structures become the labia minor and labia majora, respectively.
Conclusion
Embryology of the genital system is intimately associated with the urinary tract. Greatly impacted by chromosomal makeup, cellular proliferation and apoptosis, correct timing of events, and reciprocal differentiation, there are many opportunities for missteps and congenital anomalies. Although the majority of these malformations are not fatal, they do have lasting impacts on the infant into adulthood. An understanding of how normal anatomy is formed is essential to a solid foundation in male infertility diagnosis, evaluation, and management.