Book contents
- Escaping From PredatorsAn Integrative View of Escape Decisions
- Escaping From Predators
- Copyright page
- Dedication
- Contents
- Contributors
- Foreword
- Book part
- Part I Overview and behaviors preceding and following initiation of escape
- Part II Escape and refuge use: theory and findings for major taxonomic groups
- Part III Related behaviors and other factors influencing escape
- 10 Vigilance, alarm calling, pursuit deterrence, and predation inspection
- 11 Determinants of lizard escape performance: decision, motivation, ability, and opportunity
- 12 Sensory systems and escape behavior
- 13 The physiology of escape
- 14 Maternal and genetic effects on escape: a prospective review
- 15 The personality of escape
- Part IV The application and study of escape
- Index
- References
13 - The physiology of escape
from Part III - Related behaviors and other factors influencing escape
Published online by Cambridge University Press: 05 June 2015
- Escaping From PredatorsAn Integrative View of Escape Decisions
- Escaping From Predators
- Copyright page
- Dedication
- Contents
- Contributors
- Foreword
- Book part
- Part I Overview and behaviors preceding and following initiation of escape
- Part II Escape and refuge use: theory and findings for major taxonomic groups
- Part III Related behaviors and other factors influencing escape
- 10 Vigilance, alarm calling, pursuit deterrence, and predation inspection
- 11 Determinants of lizard escape performance: decision, motivation, ability, and opportunity
- 12 Sensory systems and escape behavior
- 13 The physiology of escape
- 14 Maternal and genetic effects on escape: a prospective review
- 15 The personality of escape
- Part IV The application and study of escape
- Index
- References
Summary
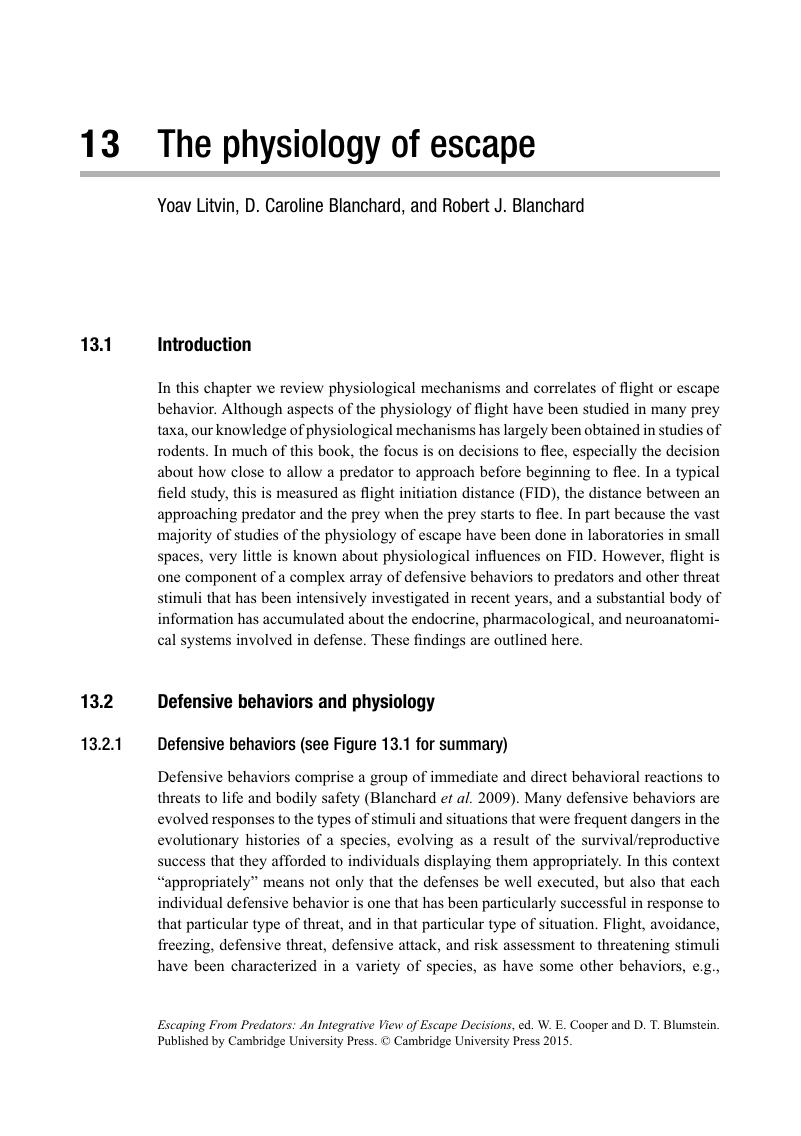
- Type
- Chapter
- Information
- Escaping From PredatorsAn Integrative View of Escape Decisions, pp. 343 - 359Publisher: Cambridge University PressPrint publication year: 2015
References
- 1
- Cited by