The prevalence of overweight and obesity is increasing and affects around 60 % of the European adult population(1). This represents an important public health concern since overweight and obesity are linked with the development of major chronic diseases and premature mortality(Reference Dai, Alsalhe and Chalghaf2,3) . As part of their Cancer Prevention Recommendations, the World Cancer Research Fund and American Institute for Cancer Research recommend to stay within healthy weight ranges and avoid weight gain in adult life(3). Main drivers of overweight and obesity are unhealthy lifestyle behaviours, including physical inactivity and consumption of dietary patterns composed of higher processed foods(Reference Hall, Ayuketah and Brychta4–Reference Valicente, Peng and Pacheco7).
It has been suggested that dicarbonyl compounds could play a role in obesity development(Reference Nigro, Leone and Fiory8) since obese individuals have higher plasma levels of dicarbonyl compounds(Reference Masania, Malczewska-Malec and Razny9). Dicarbonyl compounds are highly reactive metabolites and precursors of advanced glycation end products (AGE)(Reference Degen, Hellwig and Henle10–Reference Hellwig, Gensberger-Reigl and Henle13). They are endogenously formed from sugar fragmentation and act as intermediates in the Maillard reaction or are generated during lipid peroxidation(Reference Hellwig, Gensberger-Reigl and Henle13). Increased endogenous formation and accumulation of dicarbonyl compounds appear to contribute to dicarbonyl stress (i.e. a pro-inflammatory and oxidative glycative environment which is damaging cellular proteins and DNA)(Reference Rabbani and Thornalley14), potentially leading to impaired cell and tissue function(Reference Rabbani, Xue and Thornalley15) and the development of adverse health outcomes(Reference Rabbani, Xue and Thornalley15–Reference Li, Lee and Lee21) such as diabetes and diabetes-related vascular complications(Reference Tikellis, Pickering and Tsorotes17,Reference Schalkwijk and Stehouwer18) and the development of cancers(Reference Schalkwijk and Stehouwer18), chronic kidney diseases(Reference Wang, Ma and Liu19) and neurological disorders(Reference Kuhla, Lüth and Haferburg20,Reference Li, Lee and Lee21) . Endogenous dicarbonyl compounds are detoxified by the glyoxalase pathway, which converts glyoxal (GO) and methylglyoxal (MGO) into less reactive molecules, that is, d-lactate and glycolate(Reference Hellwig, Gensberger-Reigl and Henle13). 3-deoxyglucosone (3-DG) is metabolised by aldo-keto reductase and aldehyde dehydrogenase enzymes, which convert the dicarbonyl compound into 3-deoxyfructose and 2-keto-3-deoxygluconic acid that are then excreted via the urine(Reference Hellwig, Gensberger-Reigl and Henle13).
Dicarbonyl compounds are also present naturally in some foods or formed during food processing, such as heat treatment, storage or fermentation(Reference Degen, Hellwig and Henle10–Reference Hellwig, Gensberger-Reigl and Henle13,Reference Thornalley, Langborg and Minhas22) . Dicarbonyl compounds provide aroma and flavours to foods and are used as key sensory ingredients in food preparation and production(Reference Hellwig, Gensberger-Reigl and Henle13). MGO, GO and 3-DG are three major dicarbonyl compounds present in a wide range of commonly consumed foods and drinks, with coffee and bread being the most important single food contributors to dicarbonyl compound intakes(Reference Maasen, Scheijen and Opperhuizen12). Other important dietary sources include sugary products, such as dried fruits, bakery products, honey or candy bars(Reference Maasen, Scheijen and Opperhuizen12).
However, the long-term health effects of higher consumption of dietary dicarbonyl compounds remain largely unknown(Reference Maasen, Scheijen and Opperhuizen12), notably with regard to body weight change over time. It has been observed that higher exposure to dietary dicarbonyl compounds can lead to higher circulating concentrations(Reference Hellwig, Gensberger-Reigl and Henle13,Reference Maasen, Eussen and Scheijen23) . However, very little is known about the uptake, digestion and metabolism of dietary dicarbonyls. Although it may be assumed that dietary dicarbonyls promote dicarbonyl stress like their endogenous counterparts, recent observations show that higher intake of dietary MGO is associated with reduced inflammation(Reference Maasen, Eussen and Dagnelie24) and less insulin resistance in humans(Reference Maasen, Eussen and Dagnelie25). While dietary AGE, which are mainly derived from dicarbonyl compounds, have been linked to body weight gain and overweight(Reference Cordova, Knaze and Viallon26), little is known about associations between dietary dicarbonyl compounds and body weight change and obesity from human studies.
Therefore, the objective of the present study was to prospectively investigate associations between the three major dietary dicarbonyl compounds, MGO, GO and 3-DG, and body weight change in a sub-cohort of the European Prospective Investigation into Cancer and Nutrition (EPIC) study with repeated body weight measures throughout follow-up: the EPIC–Physical Activity, Nutrition, Alcohol, Cessation of Smoking, Eating Out of Home in Relation to Anthropometry (PANACEA) study(Reference May, Romaguera and Travier27).
Methods
Study population
The EPIC study is an ongoing prospective cohort study across twenty-three centres in ten European countries: Denmark, France, Germany, Greece, Italy, the Netherlands, Norway, Spain, Sweden and the UK(Reference Riboli, Hunt and Slimani28). From 1992 to 2000, a total of 521 323 men and women aged 25–70 years were recruited. In France, Norway, Utrecht (The Netherlands) and Naples (Italy), only women were recruited. Individuals were selected from the general population with few exceptions. In France, state-school employees participating in the national health insurance scheme were recruited. The Utrecht and Florence (Italy) centres included women invited to a local population-based breast cancer screening programme. Some centres in Italy and Spain included members of local blood donor associations. In Oxford (UK), one-half of the cohort was recruited from lacto-ovo vegetarians and vegans. The rationale and design of the EPIC have been described in detail elsewhere(Reference Riboli, Hunt and Slimani28,Reference Riboli and Kaaks29) . All participants gave written informed consent, and the EPIC study was approved by the Ethical Review Boards of the International Agency for Research on Cancer and the Institutional Review Board of each participating EPIC centre.
For the PANACEA study, some of the original twenty-three centres were combined within countries depending on their follow-up times and/or body weight measurement methods, resulting in sixteen centres. The study population was based on the EPIC cohort (n 521 323), excluding participants with extreme energy intake/energy requirement (n 5362), with no available value for body weight at baseline (n 89 954) or follow-up (n 117 258), recruited from Greek centres due to data access restrictions (n 28 561), and those with outlying anthropometry (n 17 193). More details on follow-up exclusions are given in online Supplementary Fig. 1 (Online Resource). After these exclusions, a sample of 263 095 participants (92 385 men and 170 710 women) with complete and plausible longitudinal body weight data was available for analyses.
Anthropometric measures and body weight change
Two body weight measures were available for each participant: at baseline and after a median follow-up time of 5·4 years (min.: 1·5 years for Murcia (Spain), max.: 18·9 years for Ragusa (Italy)). All centres used standardised procedures to measure body weight and height at baseline, except in France, Norway and Oxford centres, where participants self-reported their body weight. Follow-up body weight was self-reported, except for Cambridge (UK) and Doetinchem (The Netherlands) where it was measured(Reference Vergnaud, Norat and Romaguera30,Reference Vergnaud, Norat and Romaguera31) . The accuracy of self-reported anthropometric measures at baseline and at follow-up was improved with prediction equations derived from participants with both measured and self-reported body weight at baseline(Reference Spencer, Appleby and Davey32). The main outcome of this study was body weight change in kg per 5 years, calculated as body weight at follow-up minus body weight at baseline divided by the follow-up time in years and multiplied by 5 years.
Dietary assessment and estimation of dicarbonyl compound intakes
In the EPIC study, usual food intake was assessed once at baseline using validated country-specific dietary questionnaires, either self-reported or interviewer-administered(Reference Riboli, Hunt and Slimani28). In brief, three types of dietary questionnaires were used to assess habitual food consumption over the past 12 months(Reference Huybrechts, Rauber and Nicolas33) (https://www.frontiersin.org/articles/10·3389/fnut.2022·1035580/full#supplementary-material): (1) quantitative dietary questionnaires were used in North-Central Italy, Ragusa in Italy, The Netherlands, Germany, Greece, Spain and France, (2) semi-quantitative FFQ were used in Denmark, Norway, Naples (Italy) and Umeå (Sweden) and (3) a combination of semi-quantitative FFQ and 7–14 d food records was used in the UK and Malmö (Sweden). Harmonisation of food grouping and portion sizes for quantification was carried out centrally at IARC in close collaboration with EPIC centres.
Estimation of individual intakes of the three major dicarbonyl compounds was based on a reference database containing content values of MGO, GO and 3-DG (in mg/g of food) in 223 commonly consumed foods and drinks(Reference Maasen, Scheijen and Opperhuizen12). The content values were obtained from European foods measured by the ultraperformance liquid chromatography tandem mass spectrometry. Using the same method, dicarbonyl compound content was quantified in fifty-nine additional food items missing from the database but commonly consumed in EPIC countries. Food items from EPIC dietary questionnaires were then matched to the dicarbonyl compound database by name and by their associated descriptors, particularly those pertaining to preparation and/or processing in a similar manner as previously performed for the estimation of dietary AGE intake(Reference Cordova, Knaze and Viallon26). Food-specific multiplication factors were applied to dicarbonyl compound concentrations for foods that were heat-treated and available in the reference database. Generic or multi-ingredient foods that could not be matched directly were decomposed into more specific foods or ingredients based on country-specific recipes provided by previous EPIC projects(Reference Cordova, Knaze and Viallon26,Reference Slimani, Deharveng and Unwin34) . An end-user EPIC dicarbonyl compound database was then generated containing MGO, GO and 3-DG content (in mg/100 g) for all dietary questionnaire foods and recipes. In turn, this database was used to calculate (in mg/d) individual intakes of these three dicarbonyl compounds for the full EPIC cohort by multiplying with the daily intake of each food, which was based upon the reported frequency and quantity of foods consumed by each EPIC participant.
Assessment of other covariates
Validated self-administered questionnaires were used at recruitment to collect data on socio-demographic, lifestyle and other characteristics(Reference Riboli, Hunt and Slimani28). Information was obtained for the highest attained educational level (none, primary, technical, professional, secondary, higher), physical activity, which was categorised according to the Cambridge definition (inactive, moderately inactive, moderately active, active), smoking status (current, former or never smoker), alcohol intake at recruitment (in g/d) and during lifetime (never, former, current or lifetime drinkers), adherence to a Mediterranean diet pattern according to the relative Mediterranean Diet Score (categorised in scores indicative of low, medium and high levels of adherence)(Reference Buckland, González and Agudo35), medical history and for women menopausal status (pre-, post-, peri-menopausal or surgical menopause).
Statistical analyses
Baseline characteristics of the study population were described comparing highest and lowest quintiles of each dicarbonyl compound. Contribution of each dicarbonyl compound to overall intake and main food sources was also examined by comparing percentage contribution of food groups for dietary MGO, GO and 3-DG.
As primary analyses, the associations between intakes of MGO, GO, and 3-DG, and body weight change (in kg/5 years) were estimated separately using multilevel mixed linear regression models with centre as random effect and dicarbonyl compounds intake and confounders as fixed effects. MGO, GO and 3-DG intakes were natural log-transformed to improve normality and their standardised residuals were computed by regressing ln-transformed values on participant energy intake and centre. Associations with body weight change are thus expressed per 1-sd increment in the ln-transformed dicarbonyl compound intake in continuous models. Associations by sex-specific/country-specific quintiles based on ln-transformed dietary dicarbonyls were also computed, with the first quintile (lowest intake) considered the reference category. Models with three different sets of adjustments were fitted. The first model (M1) was adjusted for age, sex and BMI at baseline. Model 2 (M2) was further adjusted for follow-up time, total energy intake, educational level, physical activity, smoking status at baseline and plausibility of dietary energy reporting(Reference Goldberg, Black and Jebb36,Reference Mifflin, St Jeor and Hill37) . Model 3 (M3), considered as the main model, was additionally adjusted for Mediterranean diet, representing healthy dietary habits, using the modified relative Mediterranean Diet Score (mrMDS)(Reference Buckland, González and Agudo35). Fruits, vegetables, legumes, cereals, fish, olive oil and moderate alcohol intakes contributed positively to the mrMDS, while meat, dairy products and alcohol intake outside of the moderate range contributed negatively to the mrMDS(Reference Buckland, González and Agudo35). Because inter-correlations between the three forms of ln-transformed dietary dicarbonyls were not strong (Pearson r 0·4 between MGO and GO; 0·3 between GO and 3-DG; and 0·3 between MGO and 3-DG), M3 models were also mutually adjusted for other dietary dicarbonyl compounds. Participants with missing or unspecified values for physical activity (n 3722, 1·4 %), highest education level (n 10 251, 3·9 %) and smoking status (n 2512, 1·0 %) at baseline were classified in a separate category and included in the models. Model assumptions and fit were checked visually by plotting the residuals against each of the categorical covariates.
To assess whether the observed associations with 5-year body weight change were driven by any of the main food sources of dicarbonyl compounds, estimates for each dietary dicarbonyl by food source were computed based on M3.
Effect modification by sex, age (categorised in quartiles), BMI categories at baseline (<25, 25–30, >30 kg/m2) and categories of dietary AGE intakes (low, medium, high, defined as tertiles of the sum of Nε-Carboxymethyl-lysine (CML), Nε-1-Carboxyethyl-lysine, and Nδ-(5-Hydro-5-methyl-4-imidazolon-2-yl)-ornithines) (MG-H1) was tested a priori. This was done by including interaction terms between each potential effect modifier and each individual dietary dicarbonyl compound (continuous per 1 sd/d) in the models. P values for the interaction term were calculated using F tests and analyses were computed in each category. The associations between each dietary dicarbonyl compound and body weight change were also tested in each country separately and by European region: North (Sweden, Denmark), Central (Netherlands, UK, Germany, North of France) and South (Italy, Spain, South of France).
As secondary analyses, the risk of becoming overweight (for normal weight participants) or obese (for normal weight and overweight participants) associated with dicarbonyl compound intakes was estimated in continuous models (per 1-sd increment in the ln-transformed dicarbonyl compounds). These associations were computed using multivariable-adjusted logistic regression with the same set of covariates as M3.
Sensitivity analyses, based on M3, were performed for primary and secondary analyses to assess the robustness of the findings and address potential biases: (a) adjusting for smoking status at follow-up instead of baseline (online Supplementary model 1 (SM1)), further adjusting for the number of cigarettes smoked (in pack-years) (SM2), further adjusting for self-reported prevalent chronic conditions (myocardial infarction, angina, stroke or diabetes) at recruitment (SM3), further adjusting for dietary AGE intake (ln-transformed energy adjusted total AGE intake) (SM4) or further adjusting for coffee intake (SM5); (b) excluding participants with missing values in any of the covariates (n 14 549) (SM6), excluding participants with less than 5 years of follow-up (SM7) or excluding participants who started or quit smoking during follow-up (SM8).
All tests were two-sided, and P < 0·05 was considered statistically significant. The statistical analysis software SAS, version 9.4, was used for analyses.
Results
Characteristics of the study population
The main baseline characteristics of the study population are presented in Table 1, in the lowest (Q1) and highest (Q5) quintiles of each energy-adjusted dicarbonyl compound intake. Characteristics varied depending on dicarbonyl compounds, for instance, participants in the highest quintile of MGO intake were more often in the overweight and obese categories (39·8 % and 15·4 %, respectively), compared with the lowest quintile (35·6 % and 14·3 %, respectively). Participants with higher intake of 3-DG were more often in the normal weight category (51·5 % of participants, v. 44·5 % in the lowest quintile). There were more current smokers in participants with higher MGO intakes (30·5 % v. 17·0 % in Q1) and lower GO consumers (29·7 % v. 16·8 % in Q5). Differences in level of dicarbonyl intakes by food groups were largely aligned with the dicarbonyl compound content of each food group. For example, consumption of vegetables; fruits, nuts and seed; and cakes and biscuits was higher in each higher quintile of dicarbonyl compound, whereas intakes of dairy products and fats were lower in each Q5 compared with Q1 (Table 1). Overall, the Mediterranean diet score was higher in highest quintiles compared with lowest, for all three dicarbonyl compounds.
Table 1. Main characteristics of the study population according to the lowest (Q1) and highest (Q5) sex-specific quintile of dietary reactive dicarbonyl compounds intake – EPIC–PANACEA study (n 263 095) (Standard deviations and percentages)
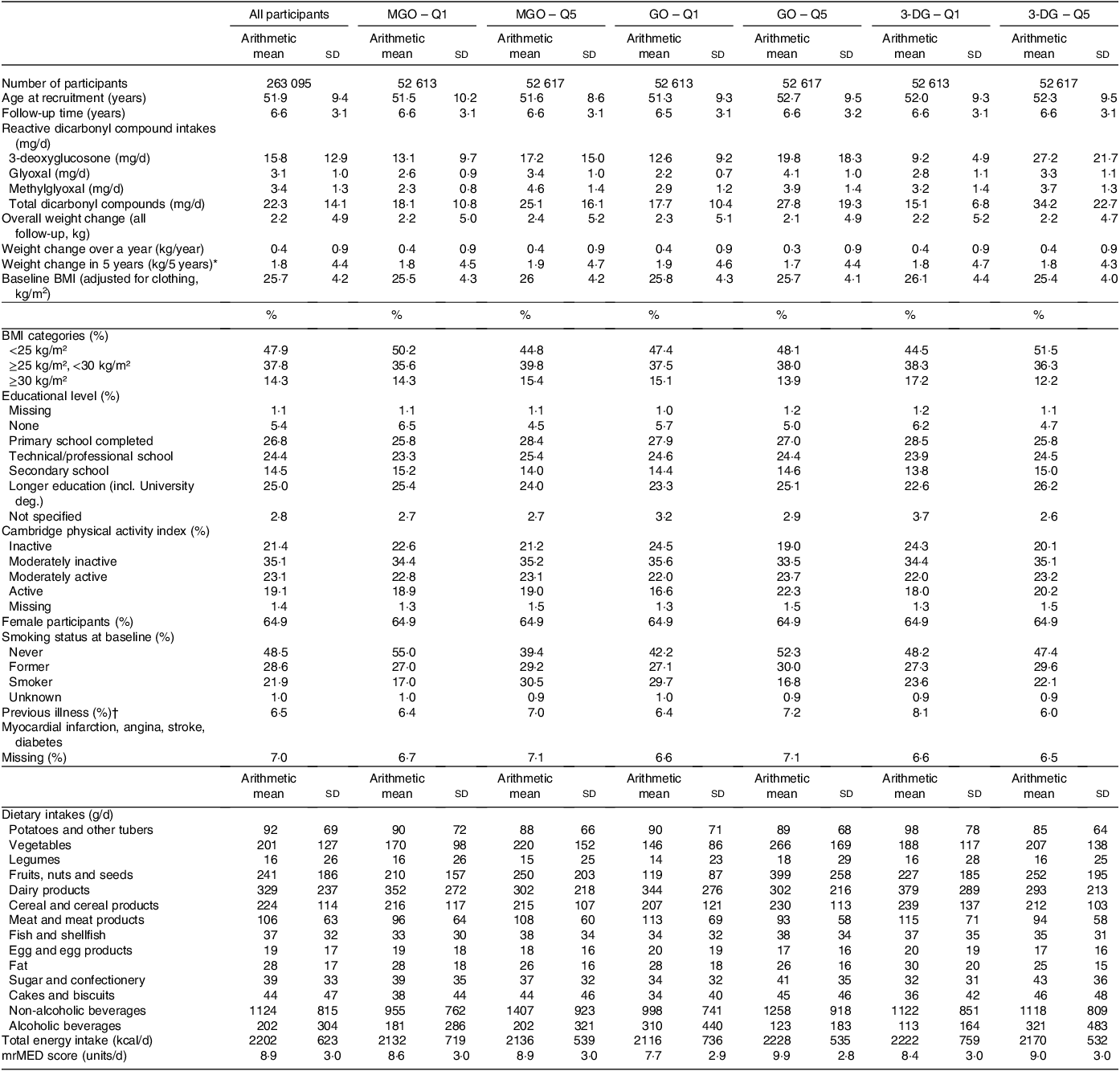
EPIC, European Prospective Investigation into Cancer and Nutrition; PANACEA, Physical Activity, Nutrition, Alcohol, Cessation of Smoking, Eating Out of Home in Relation to Anthropometry; MGO, methylglyoxal; GO, glyoxal; 3-DG, 3-deoxyglucosone.
P values for continuous variables (ANOVA) and Chi square tests for categorical variables were all < 0·001 (except for non-alcoholic beverages across quintiles of 3-DG).
Data are expressed as arithmetic mean (sd) unless stated otherwise.
Quintiles were calculated using total energy, centre-standardised residuals and log-transformed dietary dicarbonyls. First quintile corresponds to the lowest and fifth quintile to the highest intake.
BMI (calculated as weight in kg divided by height in square metres).
mrMED, modified relative Mediterranean diet score (range 0–18; higher score characterising higher adherence to the Mediterranean diet).
* Calculated as weight at follow-up minus weight at baseline divided by the follow-up time in years and multiplied by 5 years.
† Myocardial infarction (heart attack), angina, stroke, hypertension (high blood pressure) or diabetes.
Main food sources of dicarbonyls
The main dicarbonyl contributor to total dicarbonyl intake combined was 3-DG (71 %), followed by MGO (15 %) and GO (14 %) (Fig. 1). As for contribution by food groups, coffee was the main contributor to dietary MGO (31 %), followed by cereals (21 %), vegetables (10 %) and meat (8 %). For dietary GO, main contributors were cereals (23 %); fruits, nuts and seeds (21 %) and vegetables (16 %). Lastly, cereals (21 %); fruits, nuts and seeds (18 %); sugar and confectionery (16 %) and beer (10 %) were the main contributors to 3-DG intakes. Online Supplementary Figures 2–4 (Online Resource) present the percentage of contribution of main food groups of each dicarbonyl compounds by study centre.

Fig. 1. Relative contribution of food group to total intake of each of the three main forms of dicarbonyl compounds, methylglyoxal (MGO), glyoxal (GO) and 3-deoxyglucosone (3-DG) (%) – EPIC–PANACEA study (n 263 095). EPIC, European Prospective Investigation into Cancer and Nutrition; PANACEA, Physical Activity, Nutrition, Alcohol, Cessation of Smoking, Eating Out of Home in Relation to Anthropometry.
Dicarbonyl intake and 5-year changes in body weight
Between baseline and the second body weight assessment, on average 6·6 years later, the mean body weight increase in the study population was 2·2 kg with variation between participants (sd 4·9 kg). The body weight changes (in kg) over an average of 5 years according to baseline dicarbonyl compounds intake are shown in Table 2. Dietary MGO was positively associated with body weight gain (M3:0·089 kg per 1 sd higher intake/5 years, 95 % CI 0·072, 0·107). Dietary 3-DG was negatively associated with body weight gain (M3: −0·076 kg per 1 sd higher intake/5 years, 95 % CI −0·094, −0·058). Dietary GO was associated positively with body weight gain (M3: 0·018 kg per 1 sd higher intake/5 years, 95 % CI −0·002, 0·037).
Table 2. Difference in body weight gain (kg) over 5 years according to baseline dietary dicarbonyl compounds intake – EPIC–PANACEA study (n 263 095) (95 % confidence intervals)

EPIC, European Prospective Investigation into Cancer and Nutrition; PANACEA, Physical Activity, Nutrition, Alcohol, Cessation of Smoking, Eating Out of Home in Relation to Anthropometry; MGO, methylglyoxal; GO, glyoxal; 3-DG, 3-deoxyglucosone.
Multilevel linear mixed models with random effect on the intercept and slope according to centre.
Overall mean 5-year weight gain corresponded to 1·8 kg (sd 4·4) and positive beta values indicated more weight gain (kg) over the same period.
Model 1 was adjusted for age, sex and BMI at baseline.
Model 2 was further adjusted for follow-up time in years, total energy intake (kcal/d), educational level, levels of physical activity, smoking status at baseline and plausibility of dietary energy reporting.
Model 3 was further adjusted for modified relative Mediterranean diet score and for dietary dicarbonyls intake other than the one studied.
Quintiles were calculated using total energy, centre-standardised residuals and log-transformed dietary dicarbonyls.
Mean dietary MGO intake (mg/d) (sd) within the quintiles of log-transformed energy-adjusted MGO: Q1 = 2·3 (0·8), Q2 = 3·0 (0·9), Q3 = 3·4 (1·0), Q4 = 3·8 (1·1), Q5 = 4·6 (1·4).
Mean dietary GO intake (mg/d) (sd) within the quintiles of log-transformed energy-adjusted GO: Q1 = 2·2 (0·7), Q2 = 2·7 (0·7), Q3 = 3·1 (0·8), Q4 = 3·4 (0·8), Q5 = 4·1 (1·0).
Mean dietary 3-DG intake (mg/d) (sd) within the quintiles of log-transformed energy-adjusted 3-DG: Q1 = 9·2 (4·9), Q2 = 11·8 (5·6), Q3 = 13·9 (6·4), Q4 = 17·1 (8·6), Q5 = 27·2 (21·7).
Findings by quintiles of each dicarbonyl compound were in line with results of the continuous models. Participants in the highest quintiles of MGO had increased body weight gain compared with participants in the lowest quintile (P trend < 0·001; M3) (Table 2). Those in the highest quintile of 3-DG had less body weight gain compared with participants in the lowest quintile (P trend < 0·001; M3). No conclusive associations were observed with regard to GO intake by quintiles (P trend = 0·253; M3).
Estimates by main food sources of dietary dicarbonyl are presented in online Supplementary Table 1. Higher weight gain was observed for dietary MGO from coffee, vegetables and meats while MGO from cereals and cakes/biscuits was associated with less weight gain. Dietary GO from vegetables and coffee was positively associated with weight change, whereas negative associations were shown for GO from cakes/biscuits and juices. Less weight gain was observed for dietary 3-DG from cereals, fruits/nuts/seeds and cakes/biscuits, and higher weight gain was observed for 3-DG from vegetables.
Results for interaction tests and stratified analyses are presented in online Supplementary Table 2. An interaction was observed between sex and dietary GO (P interaction = 0·001). Additionally, analyses stratified by sex show higher body weight gain among women (0·045 kg per 1 sd higher intake of GO /5 years, 95 % CI 0·021, 0·070) but not among men (–0·017 kg per 1 sd higher intake of GO /5 years, 95 % CI −0·050, 0·016). No significant sex-specific interactions were detected with dietary MGO and 3-DG.
Significant P interaction values were observed between age at recruitment and dietary GO and 3-DG. Associations with body weight gain for dietary GO were more pronounced in participants in the third quartile (0·053 kg per 1 sd higher intake of MGO /5 years, 95 % CI 0·015, 0·091). Association between dietary 3-DG and lower body weight gain was, in turn, more important in younger participants (–0·099 kg per 1 sd higher intake/5 years, 95 % CI −0·137, −0·062) (online Supplementary Table 2).
Significant interactions were suggested between baseline BMI and dietary GO (P interaction = 0·001) and 3-DG (P interaction = 0·002), but not MGO (P interaction = 0·112). For GO, stratified analyses suggested higher body weight among overweight and obese BMI categories compared with normal weight participants, but the associations were not significant. For 3-DG, lower body weight gain was observed in < 25 kg/m2 and there were no significant associations among participants with a BMI > 30 kg/m2. Interaction tests between each dicarbonyl compound and tertiles of dietary AGE suggested significant interaction for GO only (P interaction 0·003), with higher associations with body weight gain in participants in the lowest tertile of AGE intake.
Results of the analyses conducted in each specific country were similar to the main associations. In all countries, except Denmark, dietary MGO was associated with higher body weight change. In all countries, except Italy, dietary 3-DG was associated with lower body weight change. Dietary GO was not significantly associated with body weight change except in Spain and Denmark (positive associations) and Germany (negative association). Results by European region were similar except for a significant association observed between dietary GO and body weight change in South Europe. In North Europe, the association with dietary 3-DG was not significant.
Dicarbonyl intake and risk of becoming overweight or obese
Results for the associations between dietary dicarbonyls and the risk of becoming overweight or obese are shown in Table 3. Among normal weight participants at baseline (n 126 015), 29 880 became overweight and 553 became obese at follow-up. Among overweight participants at baseline (n 99 438), 15 127 became obese at follow-up. For normal weight participants at baseline, MGO intake was associated with an increased risk of becoming overweight (OR per 1 sd higher intake 1·02, 95 % CI 1·01, 1·03; P-value = 0·006) or obese at follow-up (OR = 1·14 (1·05, 1·25) P-value = 0·002). Similarly, among overweight participants, MGO was associated with an increased risk of becoming obese (OR = 1·02, (1·01, 1·04) P-value = 0·008). Results suggested that dietary 3-DG was associated with lower risks of becoming overweight (OR = 0·98 (0·97, 0·99) P-value = 0·005) or obese (OR = 0·93 (0·85, 1·02) P-value =0·135) among normal weight participants or obese among overweight participants (OR = 0·98 (0·97, 1·00) P-value = 0·085). No associations were observed for dietary GO and the risk of becoming overweight (OR = 0·99 (0·98, 1·01) P-value = 0·386) or obese at follow-up (OR = 1·06 (0·96, 1·17) P-value = 0·268, among normal weight participants at baseline, and OR = 1·00 (0·98, 1·02) P-value 0·951, among overweight participants at baseline).
Table 3. Risk of becoming overweight or obese according to baseline BMI – OR and 95 % CI obtained using multivariable-adjusted logistic regression models – EPIC–PANACEA study (n 263 095)

EPIC, European Prospective Investigation into Cancer and Nutrition; PANACEA, Physical Activity, Nutrition, Alcohol, Cessation of Smoking, Eating Out of Home in Relation to Anthropometry; MGO, methylglyoxal; GO, glyoxal; 3-DG, 3-deoxyglucosone.
Multivariable-adjusted logistic regression models.
Main model (M3): adjusted for age, sex, BMI at baseline, follow-up time in years, total energy intake (kcal/d), educational level, levels of physical activity, smoking status at baseline, plausibility of dietary energy reporting, modified relative Mediterranean diet score and dietary dicarbonyls intake other than the one studied.
Using total energy, centre-standardised residuals and log-transformed dietary dicarbonyls.
Additional analyses
Results of sensitivity analyses for the difference in 5-year body weight change are presented in online Supplementary Table 3. Findings for dietary MGO and 3-DG are similar across sensitivity analyses, but results were attenuated when M3 was additionally adjusted for coffee intake. However, dietary GO was associated with 5-year body weight gain when M3 was additionally adjusted for the number of cigarettes smoked in pack-years (SM2: 0·024 kg per 1 sd higher intake/5 years, 95 % CI 0·004, 0·043) or when the analyses were restricted to participants with at least 5 years of follow-up (SM7: 0·031 kg per 1 sd higher intake/5 years, 95 % CI 0·010, 0·052). Results for the risk of becoming overweight or obese are displayed in online Supplementary Table 4 and are consistent in all online Supplementary models, except for SM5 additionally adjusted for coffee intake for which no significant associations were observed.
Discussion
Principal findings
In this prospective analysis, higher intake of dicarbonyl compounds was associated with body weight change over a 6·6-year average follow-up in adults from eight European countries. Body weight changes were in divergent directions depending on the dicarbonyl compound. Dietary MGO intake was associated with greater body weight gain and higher risks of becoming overweight or obese, whereas dietary 3-DG was inversely associated with body weight gain and a lower risk of becoming overweight or obese. Dietary GO was not significantly associated with weight change overall. However, in sub-group analyses, dietary GO showed a positive association in women and older participants, but not in men or younger participants. Significant interactions by age quartiles were observed for dietary GO (higher body weight gain among older participants) and 3-DG (inverse association with body weight gain among younger participants). 3-DG also demonstrated an interaction with BMI (inverse association with body weight gain among normal weight participants, and no significant association among participants with obesity).
Comparison with literature and hypotheses of underlying mechanisms
This is the first prospective study investigating associations between dietary dicarbonyl compounds and body weight changes over time. Previous analyses within the same cohort showed that higher intakes of AGE (i.e., CML, CEL and MG-H1) were associated with body weight gain(Reference Cordova, Knaze and Viallon26). Since dicarbonyl compounds are precursors of AGE and exert similar metabolic effects(Reference Maasen, Scheijen and Opperhuizen12), their higher dietary consumption was expected to be positively associated with body weight change. This was the case for dietary MGO, one of the main precursors of AGE(Reference Maessen, Stehouwer and Schalkwijk16), but not for the other two main dietary dicarbonyls (i.e., GO, 3-DG) that were also assessed. There is currently scant understanding of the efficiency of absorption and conversion of dietary dicarbonyl compounds to AGE upon consumption, and whether their potential impacts on weight gain may in fact be more due to their conversion to AGE than directly. MGO leads to production of MG-H1 and CEL(Reference Hellwig, Gensberger-Reigl and Henle13), which have previously been observed to be associated with weight gain in the same cohort(Reference Cordova, Knaze and Viallon26). Similarly, CML can be produced from both GO (via reaction with lysine) and 3-DG (via reaction with protein amino groups). But, in the present study 3-DG was not associated with weight gain, while dietary CML has previously been(Reference Cordova, Knaze and Viallon26). In our attempt to disentangle these relationships, we adjusted our models for the level of dietary AGE intake, but this did not modify our observations. However, this would not address the potential conversion of dietary dicarbonyls into AGE within the digestion tract or post-absorption. Moreover, 3-DG can also be converted to other AGE such as pyrraline, pentosidine, imidazolone and 3DG-H1(Reference Hellwig, Gensberger-Reigl and Henle13), but there is currently scant information on the potential weight gain impact of these AGE in humans. It can also be postulated that dietary dicarbonyls may not be as reactive as their endogenously produced counterparts, for example, if they are trapped within the matrix of a food and unabsorbed from the digestive tract or metabolised by the gut microbiome. Moreover, very little is known about the uptake and stability of these compounds in the digestive tract, how they may be absorbed, the efficiency of their absorption across different ranges of intake and how they are metabolised in the liver upon absorption from the digestive tract.
Underlying mechanisms for the association observed between dietary dicarbonyls and body weight change are not clear. However, dietary patterns high in ultra-processed foods and sugars (e.g. processed baked goods, biscuits, sugary breakfast cereals, candy bars, etc.) are rich in dicarbonyl compounds(Reference Maasen, Scheijen and Opperhuizen12) and are associated with increased weight gain(Reference Hall, Ayuketah and Brychta4–Reference Cordova, Kliemann and Huybrechts6,Reference Te Morenga, Mallard and Mann38,39) . In fact, these types of dietary patterns are less satiating, highly palatable(Reference Hall, Ayuketah and Brychta4) and linked to excessive energy intakes(Reference Hall, Ayuketah and Brychta4,Reference Te Morenga, Mallard and Mann38) . In Europe, ultra-processed foods represent on average 27 % of energy intake. This proportion reaches higher percentages in some countries, such as the UK (41 %) or Sweden (44 %). Additionally, in Finland, Spain and the UK the consumption of ultra-processed food seems to keep increasing over time (by 3–9 %)(Reference Mertens, Colizzi and Peñalvo40). Thus, positive weight changes observed in the current study could be partly explained by higher intakes of sugar-rich processed and ultra-processed foods. Nonetheless, this was largely accounted for in our models by adjusting for energy, suggesting that other mechanisms could potentially be involved. Findings of Maasen et al. suggested that higher intakes of dietary MGO and GO could lead to higher plasma MGO and GO concentrations in human(Reference Maasen, Eussen and Scheijen23). This could increase dicarbonyl stress, which, in turn, could be obesity promoting(Reference Nigro, Leone and Fiory8). In contrast, higher dietary MGO has been associated with less low-grade inflammation in participants of the Maastricht Study(Reference Maasen, Eussen and Dagnelie24). Overall, these results suggest that further studies are needed to better understand the role and underlying metabolic effects of those important and common dietary compounds.
Coffee is the main contributor to dietary MGO (over 30 % of total intakes). As suggested in previous studies, higher coffee consumption is more likely to protect against body weight gain(Reference Lopez-Garcia, van Dam and Rajpathak41–Reference Lee, Lim and Kim43), but it is unclear whether this effect may be due to its MGO content or another coffee component. Additional adjustment for coffee intake in our analyses seemed to attenuate our observed association between dietary MGO and increased body weight gain. A previous study in EPIC–PANACEA suggested a link with increased body weight gain for hydroxycinnamic acids, a subclass of polyphenols contained mostly in coffee, whereas other polyphenols were associated with lower body weight gain(Reference Gil-Lespinard, Castañeda and Almanza-Aguilera44). This requires further research around coffee consumption and its constituents.
Of the three dietary dicarbonyl compounds, GO is the one most commonly derived from fruits and vegetables (37 %, v. 16 and 27 % for MGO and 3-DG, respectively). Higher fruits and vegetables consumption is in turn negatively associated with the risk of overweight(45). Thus, inverse associations between dietary GO and body weight gain in crude models are likely due to confounding, as evidenced in loss of significance in the more adjusted models. Indeed, quintile 5 estimates differ substantially from M2 to M3. The latter model was adjusted for Mediterranean diet, which accounts for healthier diet patterns observed among participants with higher GO intakes. Dietary GO seemed to be associated with body weight gain in subgroups of the population, that is, women and older participants. Potential underlying mechanisms involved in the association between dietary GO intakes and greater body weight gain could be similar to the ones suggested for MGO. GO are precursors of CML, a dietary AGE previously associated with weight gain(Reference Cordova, Knaze and Viallon26). Dietary GO could participate in increased dicarbonyl stress and lead to deleterious metabolic health effects, including oxidative stress, insulin resistance or inflammation(Reference Schalkwijk and Stehouwer18,Reference Kolb, Stumvoll and Kramer46,Reference Gao, Bielohuby and Fleming47) .
A previous experimental study suggested that dietary 3-DG could inhibit glucagon-like peptide 1(Reference Wang, Zhou and Song48), which has been associated with weight loss in clinical trials(Reference Ard, Fitch and Fruh49). Unexpectedly, in the present study, dietary 3-DG was associated with reduced body weight change. This observation requires additional validation, and the potential underlying mechanisms need to be elucidated. A recent study suggests that potential harmful effects of dicarbonyl compounds could, to some extent, be bypassed by other components of the diet with anti-inflammatory properties(Reference de Graaf, Scheijen and Spooren50). Also, as previously suggested for dietary MGO(Reference Watson, Soro-Paavonen and Sheehy51,Reference Zemva, Fink and Fleming52) , dietary 3-DG may also be subject to a “hormetic effect” wherein certain levels of exposure could be considered as advantageous by stimulating detoxification, activation of signalling pathways and increased antioxidant activity(Reference Nigro, Leone and Fiory8). The current incomplete understanding of the metabolism of dietary dicarbonyl compounds and their contributions to endogenous dicarbonyl compound and AGE pools requires further research.
Strengths and limitations
The main strengths of this study are its prospective design, large sample size, inclusion of participants from eight European countries, detailed dietary and lifestyle data collections and repeated body weight change data, coupled to dietary dicarbonyl compound intake estimates derived from the most extensive food composition database for dicarbonyl compounds currently available with information from 282 commonly consumed foods and drinks in Europe(Reference Maasen, Scheijen and Opperhuizen12).
Nonetheless, several limitations are also present. First, although the food composition dataset we applied for this study is large and detailed, it may not cover all foods and account adequately for different food preparation/processing methods. In addition, potential low-level exposure to dicarbonyl compounds from drinking water could not accounted for(Reference Hellwig, Gensberger-Reigl and Henle13,Reference Fujioka and Shibamoto53,Reference Bao, Pantani and Griffini54) . Any exposure for dicarbonyl compounds from smoking is highly likely to have been accounted for by adjustments for smoking in our main model and hence unlikely to influence our findings. Second, only three dicarbonyl compounds out of many were considered in our study. It remains unclear if the presence or formation of other dicarbonyl compounds in foods could play a role in body weight changes. Third, dietary dicarbonyl compounds are potentially rapidly converted to AGE and there is still very little information on the absorption dynamics of dietary dicarbonyls, how and to what extent they are absorbed and whether they are converted to AGE within the digestive tract or post-absorption and their accumulation and effects in different tissues and body compartments. Circulating plasma concentrations need to be measured to assess exposure levels relevant to body weight change. Lastly, possible residual confounding cannot be discounted, and dietary intake estimates were only available at baseline, and it was not possible to assess dietary dicarbonyl compounds accumulation over time or alterations in intake levels that may have influenced eventual weight changes.
Conclusion
In conclusion, in this large-scale prospective cohort study we observe modest associations between dietary dicarbonyl compound MGO, a major precursor of AGE, and increased body weight over a 5-year period. Conversely, dietary 3-DG was inversely associated with body weight gain. The possible underlying mechanisms of these observed associations remain unclear, further epidemiological and experimental studies are needed to clarify how these and other dietary dicarbonyl compounds and AGE behave and interact in weight change, as well as in other chronic diseases.
Supplementary material
For supplementary material/s referred to in this article, please visit https://doi.org/10.1017/S0007114524000503
Acknowledgements
The authors would like to thank the EPIC study participants and staff for their valuable contribution to this research. We thank Bertrand Hemon for the preparation of the databases. We also thank Petra H. Peeters and Anne M. May from the Julius Centre for Health Sciences and Primary Care, University Medical Centre Utrecht, The Netherlands, for coordinating the EPIC–Panacea study.
We are grateful to the Hyblean Association for Epidemiological Research AIRE – ONLUS, which is a data contributor of EPIC-Ragusa.
The EPIC-Norfolk study (DOI 10.22025/2019.10.105.00004) has received funding from the Medical Research Council (MR/N003284/1 and MC-UU_12015/1) and Cancer Research UK (C864/A14136). We are grateful to all the participants who have been part of the project and to the many members of the study teams at the University of Cambridge who have enabled this research.
The authors are grateful for the support and contributions of the EPIC centres of Aarhus, Denmark; Villejuif, France; Asturias, Spain; Bilthoven, The Netherlands; Utrecht, The Netherlands; and Oxford, UK, for their contribution and support to the present study.
The coordination of EPIC is financially supported by International Agency for Research on Cancer (IARC) and by the Department of Epidemiology and Biostatistics, School of Public Health, Imperial College London which has additional infrastructure support provided by the NIHR Imperial Biomedical Research Centre (BRC). The national cohorts are supported by: Danish Cancer Society (Denmark); Ligue Contre le Cancer, Institut Gustave Roussy, Mutuelle Générale de l’Education Nationale, Institut National de la Santé et de la Recherche Médicale (INSERM) (France); German Cancer Aid, German Cancer Research Center (DKFZ), German Institute of Human Nutrition Potsdam Rehbruecke (DIfE), Federal Ministry of Education and Research (BMBF) (Germany); Associazione Italiana per la Ricerca sul Cancro-AIRC-Italy, Compagnia di San Paolo and National Research Council (Italy); Dutch Ministry of Public Health, Welfare and Sports (VWS), Netherlands Cancer Registry (NKR), LK Research Funds, Dutch Prevention Funds, Dutch ZON (Zorg Onderzoek Nederland), World Cancer Research Fund (WCRF), Statistics Netherlands (The Netherlands); Health Research Fund (FIS), Instituto de Salud Carlos III (ISCIII), Regional Governments of Andalucía, Asturias, Basque Country, Murcia and Navarra and the Catalan Institute of Oncology, ICO (Spain); Swedish Cancer Society, Swedish Research Council, Region Skåne and Region Västerbotten (Sweden); Cancer Research UK (14136 to EPIC-Norfolk; C8221/A29017 to EPIC-Oxford), Medical Research Council (1000143 to EPIC-Norfolk; MR/M012190/1 to EPIC-Oxford) (UK). The authors are grateful for the centres of Cambridge and Utrecht, which also provided their data for this study. The funders had no role in study design, data collection and analysis, decision to publish or preparation of the manuscript.
This project has received co-funding from the PPP Allowance made available by Health Holland, Top Sector Life Sciences & Health, to stimulate public–private partnerships under the umbrella of the European Joint Programming Initiative “A Healthy Diet for a Healthy Life” (JPI HDHL) and of the ERA-NET Cofund HDHL INTIMIC (GA N 727565) of the EU Horizon 2020 Research and Innovation Programme.
C. G. S. (PI) and M. J. (work-package leader) applied for and obtained project funding from the JP’s Impact of Diet, Food Components and Food Processing on Body Weight Regulation and Overweight Related Metabolic Diseases (METADIS 2019) joint funding action. H. F. and M. J. developed the overall research plan; V. Kn. and R. C. performed the data matching; C. G. S. provided the dicarbonyl compounds database; C. D. conducted the statistical analyses. C. D. and M. J. wrote the manuscript; M. J. supervised the data analysis, reviewed/edited the manuscript and had primary responsibility for final content and all authors (C. D., R. C., A. L. M., K. M., V. Kn., S. J. P. M. E., C. G. S., I. H., A. T., J. H., V. Ka., R. B., M. B. S., G. M., V. P., F. P., A. M., D. P., J. C., C. S., P. A., C. M. I., Y. B., E. S., I. J., A. E., E. K. A., M. J. and H. F.): contributed substantially to data collection, interpretation of data and drafting or critical revision of the manuscript for important intellectual content. All authors have read and approved the final manuscript.
For information on how to submit an application for gaining access to EPIC data and/or biospecimens, please follow the instructions at http://epic.iarc.fr/access/index.php.
Where authors are identified as personnel of the International Agency for Research on Cancer/WHO, the authors alone are responsible for the views expressed in this article and they do not necessarily represent the decisions, policy or views of the International Agency for Research on Cancer/WHO.
None of the authors declared a conflict of interest.
The present study was approved by the ethics committees of the IARC and the individual study centres.