Vitamin D deficiency is now recognised as a worldwide problem, which even affects athletic populations(Reference Larson-Meyer and Willis1). Previous findings show associations between vitamin D deficiency and some chronic conditions such as CVD, cancer, type 2 diabetes and neurological disorders(Reference Holick2,Reference Baggerly, Cuomo and French3) . Vitamin D plays an important role in immunomodulation, which can be beneficial not only in treatment and in management of inflammatory diseases but also for exercise-related inflammation(Reference Larson-Meyer and Willis1,Reference Guillot, Semerano and Saidenberg-Kermanac’h4) . Besides the immune system, literature also suggests that vitamin D has effects on muscular function through its role in muscle cell maturation, growth, repair and mitochondrial function, which potentially links this secosteroid to physical capability(Reference Willis, Peterson and Larson-Meyer5). Indeed, prior results indicate that low levels of vitamin D can directly impair both muscle strength and sports performance(Reference Miraj, Thunga and Kunhikatta6). Therefore, improving vitamin D deficiency seems essential to achieve optimal physiological effects during and following exercise(Reference Garcia, Ferrini and Norris7,Reference Sinha, Hollingsworth and Ball8) .
It is well established that acute resistance exercise (RE) enhances glucose uptake and increases insulin action(Reference Henriksen9). Additionally, acute RE can induce muscle damage and prompt a temporary inflammatory response, which is dependent on the intensity and duration of the session(Reference Ghafourian, Ashtary-Larky and Chinipardaz10). As a response, creatine kinase (CK) levels are increased, triggering the release of pro-inflammatory cytokines such as TNF-α and IL-1(Reference Bruunsgaard, Galbo and Halkjaer-Kristensen11). It is also known that the plasma level of the anti-inflammatory myokine IL-6 increases during intense or prolonged exercise(Reference Petersen and Pedersen12), producing beneficial metabolic effects and contributing to the maintenance of glucose homeostasis and exercise-induced lipolysis(Reference Petersen and Pedersen12). Recent findings indicate that vitamin D3 supplementation attenuates exercise-induced muscle damage in rats(Reference Choi, Park and Cho13). Furthermore, vitamin D supplementation resulted in an improved resistance and sensitivity to insulin in different populations(Reference Houmard, Tanner and Slentz14). However, the effects of a vitamin D3 injection on the inflammatory and metabolic response to an acute bout of RE in vitamin D-deficient athletic cohorts have not been previously evaluated. Furthermore, very little is identified about vitamin D improvement and its physiological effects among athletes and fitness enthusiasts. These represent a gap in knowledge that must be addressed. Therefore, the objective of the present study was to evaluate the effects of a single vitamin D3 injection on the metabolic, inflammatory and muscular damage responses to an acute bout of RE in vitamin D-deficient resistance-trained males. We hypothesised that a single vitamin D3 injection would improve the metabolic, inflammatory and muscular damage markers following acute RE.
Experimental methods
Participants
Fourteen resistance-trained healthy males between the ages of 20 and 30 years old volunteered for the present study. Participants were recruited from a group of individuals referred to a sport nutrition clinic (Ahvaz, Iran). All participants regularly performed RE, at least 3 d a week, for at least 2 years prior to the study. Participants were vitamin D deficient (25-hydroxycholecalciferol (25(OH)D) level < 20 ng/ml), but had no history of diabetes, cardiovascular, inflammatory or thyroid diseases or were taking any medications or nutritional supplements that could affect the inflammatory and/or metabolic response. Additionally, participants did not smoke, consume alcohol or had dietary changes in the year before the study. All subjects gave written informed consent, and the ethical committee of Ahvaz Jundishapur University of Medical Sciences approved the protocol (Ethic code: IR.AJUMS.REC.1394.212).
Study design
We used a non-randomised within-subject crossover design (Fig. 1), in which each participant served as their own control(Reference Lloyd, Evans and Zerfass15). Besides a familiarisation session, participants were evaluated in two different occasions, in which they participated in acute RE bouts 4 weeks after normal saline (lower vitamin D (LVD) trial) and vitamin D3 (higher vitamin D (HVD) trial) injections. The experiments were conducted in the afternoon, in temperature-controlled room (23°C) and at the same time of the day (±2 h). Participants abstained from caffeinated drinks for 12 h and avoided intense exercise 72 h before each testing session. Anthropometric measurements were collected upon entering the laboratory, before each RE session. Thereafter, blood samples, heart rate (HR) and blood pressure (BP) measurements were collected at rest, immediately and 1 h after acute RE. Four weeks before the first RE session, 1 ml of normal saline was injected. Approximately 24 h after the first RE session, an intramuscular injection of 7500 μg vitamin D3 was performed on each participant. A second RE session was conducted 4 weeks after vitamin D3 injection; this period allowed for the improvement in the vitamin D values of the participants (Fig. 1)(Reference Wylon, Drozdenko and Krannich16).
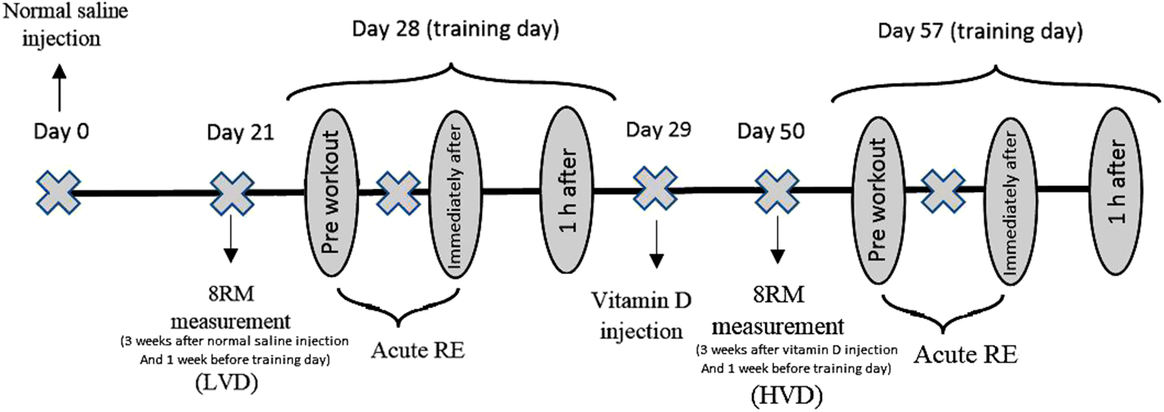
Fig. 1. Study design. HVD, high vitamin D; LVD, low vitamin D; RE, resistance exercise; RM, repetitions maximum.
Since diet plays an important role in metabolic and systemic inflammation, food records were taken 3 d before each RE trial. To equalise macronutrient intake in exercise day, pre-exercise meals and its contents calculated based on each subject’s body weight (Table 1).
Table 1. Amount of macronutrient intake on exercise days

8 Repetition maximum testing
One week before each acute RE trial, participants underwent 8 repetition maximum (RM) testing to prescribe RE intensity during the trials. The 8RM was assessed for the nine exercises used in acute RE, as described in previous research(Reference Miranda, Fleck and Simão17).
Acute resistance exercise protocol
In both trials, acute RE consisted of three sets of eight repetitions (using 8RM load) and 1-min rest between sets and exercises. The exercises included barbell bench press, dumbbell bench press, incline barbell press, incline dumbbell fly, cable crossover, barbell curl, incline dumbbell curl, lying triceps extension and close-grip bench press. If needed, the participants were spotted to complete the eight repetitions in each set(Reference De Souza, Tibana and Cavaglieri18). The acute RE protocol was adapted from the previous literature(Reference De Souza, Tibana and Cavaglieri18,Reference George, Pearson and Witham19) .
Anthropometric measurements
Body composition was measured using a precision body composition analyser (inbody 270, Biospace) as previously described(Reference Ghanavati, Behrooz and Rashidkhani20).
Blood pressure measurements
BP, both systolic and diastolic BP, was measured in the supine position by an automatic BP monitor (Jawon Medical). Rate pressure product was estimated by systolic BP × HR. The HR was obtained utilising HR monitor (Beurer, PM70). Mean arterial pressure was calculated according to the formula: (2 diastolic BP + systolic BP)/3.
Biochemical and inflammatory analysis
A volume of 5 ml of blood samples was collected from the cubital vein of subjects. Venous blood was also drawn immediately after the exercise and 1 h after the exercise. Blood sugar (BS), CK and lactate dehydrogenase (LDH) were measured by the spectrophotometric method (Pars Azmoon Inc.) using an auto-analyser (Hitachi). Inter- and intra-assay CV were <2·2 % for glucose, 1·6 % for CK and 1·2 % for LDH. Fasting serum insulin is assayed by an ELISA kit (Diaplus Inc.) with intra- and inter-assay CV of 4·9 and 8 %, respectively. Insulin resistance and pancreatic β-cell function were estimated using homeostasis model assessment (HOMA)(Reference Ghaffari, Payami and Payami21,Reference Matthews, Hosker and Rudenski22) . The 25(OH)D level of each sample was measured by an ELISA kit (Bioactiva Diagnostica). The secreted cytokine levels including IL-6, IL-10 and TNF-α in the serum samples were determined using commercially available Sandwich ELISA kits (Eastbiopharm). All inter- and intra-assay CV for the inflammatory markers were <12 and <10 %, respectively.
Statistical analysis
An estimation of an appropriate sample size was conducted using the G*Power analysis software(Reference Faul, Erdfelder and Lang23). Our rationale for sample size was based on a previous study, which evaluated changes in glucose, insulin and HOMA-IR scores after acute RE in twelve young men(Reference Kazemi24). The results of the present study showed significant declines in glucose, insulin and HOMA-IR following RE. Using the equation for effect size = (mean before − mean after acute RE)/the pooled standard deviation, the present study revealed an effect size of 0·19 = ((91·48 − 82·98)/45·8), 0·36 = ((4·87 − 4·69)/0·5) and 0·35 = ((1·10 − 0·96)/0·4) for glucose, insulin and HOMA-IR, respectively. Based on α = 0·05, a power (1 − β) of 0·80 and an effect size = 0·4 (highest approximate effect size for our variables), a sample size of at least eleven participants was needed to have sufficient power to detect significant changes in BS, insulin and HOMA-IR after acute RE among our two study trials. The Kolmogorov–Smirnov test was used to check the normality of the observations. Differences in mean values for each variable between groups were compared by a 2 × 3 ANOVA with repeated measures, followed by LSD test for pairwise comparisons. Pearson correlation coefficients were utilised to examine the relationships between intensity of exercise and all baseline anthropometric indices, metabolic, inflammatory and BP changes during exercise. Statistical significance was considered as P < 0·05. All data are presented as mean values and standard deviation. The SPSS version 19.0 was used for analysing the data.
Results
Effects of vitamin D injection on anthropometrics and exercise load
Participants’ baseline characteristics and exercise load for each trial are presented in Table 2. Other than vitamin D level, no significant differences were observed in baseline characteristics between trials (P > 0·05). Additionally, there were no significant differences in anthropometric values and exercise load between trials.
Table 2. Participant characteristics and exercise load for each trial (Mean values and standard deviations)

LVD, low vitamin D; HVD, high vitamin D; LBM, lean body mass; FM, fat mass; TBW, total body water; FFM, fat-free mass; PBF, percentage body fat; WC, waist circumference; HC, hip circumference; 25(OH)D, 25-hydroxycholecalciferol.
Effects of vitamin D injection on the metabolic responses to resistance exercise
Metabolic responses to RE are shown in Fig. 2. There were significant trial-by-time interactions for insulin and HOMA-IR which significantly (P < 0·05) declined 1 h after RE in the HVD trial when compared with LVD trial. Only a time effect was detected for HOMA-IR immediately and 1 h after RE in the LVD trial. HOMA-B levels were significantly (P < 0·05) reduced 1 h after RE in the HVD trial when compared with LVD trial; however, no significant intra-trial differences were achieved (P = 0·07). There was also a time effect for BS which significantly (P < 0·05) decreased 1 h after RE in both the LVD and HVD trials. There were no significant changes in the level of C-peptide following RE in both LVD and HVD trials.
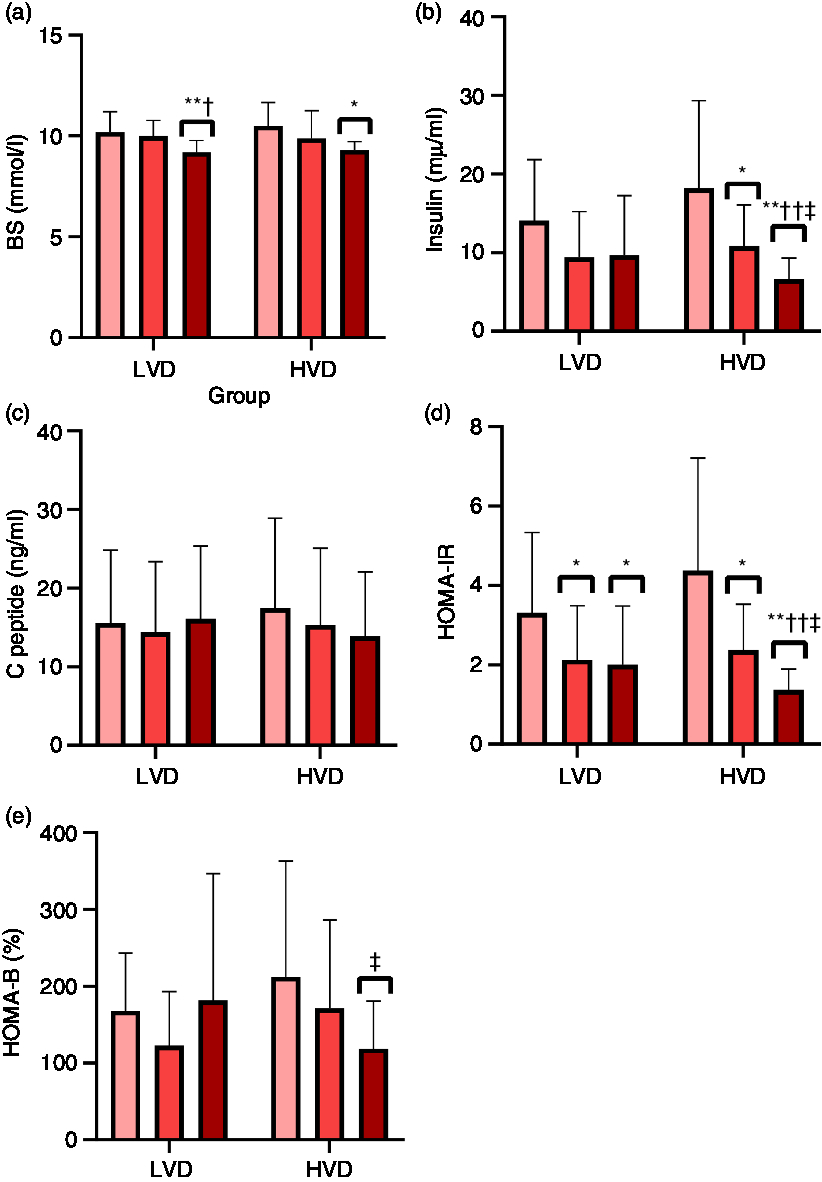
Fig. 2. Metabolic responses to acute resistance exercise in the LVD (low vitamin D) and HVD (high vitamin D) interventions. (a) BS, blood sugar; (b) insulin; (c) C peptide; (d) HOMA-IR, homeostasis model of assessment-insulin resistance; (e) HOMA-B, homeostasis model of assessment-β-cell function assessment of insulin secretory capacity. * P < 0·05 and ** P < 0·01 compared with the values of pre workout. † P < 0·05 and †† P < 0·01 compared with the values of immediately after workout, respectively. ‡ P < 0·05 compared with LVD at the same time period. , Preworkout;
, immediately after;
, 1 h after.
Effects of vitamin D injection on the muscle damage, inflammatory and cardiovascular responses to resistance exercise
Muscle damage, inflammatory and cardiovascular responses are shown in Table 3. Muscle damage markers, CK and LDH increased significantly (P < 0·05) immediately and 1 h after RE in both trials. However, differences between trials were insignificant (time-effect only).
Table 3. Muscle damage, inflammatory and cardiovascular responses to acute resistance exercise (Mean values and standard deviations)

CK, creatine kinase; LDH, lactate dehydrogenase; SBP, systolic blood pressure; DBP, diastolic blood pressure; PP, pulse pressure; HR, heart rate; RPP, rate-pressure product; MAP, mean arterial pressure; LVD, low vitamin D; HVD, high vitamin D.
*P < 0.05 and ** P < 0.01 compared with the values of pre workout time.
†P < 0.05 and †† P < 0.01 compared with the values of immediately after workout, respectively.
‡ Values are significantly different (P < 0.05).
Amongst the inflammatory biomarkers analysed, significant increases (P < 0·05) were observed in the level of IL-6 immediately and 1 h after RE in both trials. A significant rise was also seen in the ratio of IL-6:IL-10 in the LVD trial only, but no significant differences between trials were observed. There were no significant changes in the level of TNF-α, IL-10 and the ratio of TNF-α:IL-10 in both the LVD and HVD trials.
Systolic BP, HR, rate pressure product and pulse pressure significantly (P < 0·05) increased immediately and returned to baseline 1 h after RE in both trials. There were no significant differences in response to these variables between trials (time-effect only). Diastolic BP stayed unchanged in both trials.
Discussion
The present study aimed at examining the effect of a single intramuscular high dose of vitamin D3 injection of 7500 μg on inflammatory, muscular damage, metabolic and cardiovascular responses to an acute bout of RE in previously vitamin D-deficient resistance-trained males. Our finding showed that the injection declined insulin levels, improved insulin resistance and β-cell function following RE. Conversely, a single injection of vitamin D3 did not change muscle damage, cardiovascular and the inflammatory responses to acute RE in previously vitamin D-deficient resistance-trained males.
Skeletal muscle tissue is responsible for most insulin-dependent glucose uptake in humans. It was previously reported that the capacity of glucose uptake mediated by insulin is directly associated with the muscle mass and adversely related to fat mass(Reference Richter and Hargreaves25). In our study, a strong inverse relationship was observed between reduced BS levels after exercise with muscle mass but not fat mass. In comparison with the endurance exercise, there is little information in relation to the potential effects of RE on insulin sensitivity or glucose resistance in vitamin D-deficient athletic populations. Based on our findings, in both trials, the amount of insulin and HOMA-IR declined significantly immediately after RE, but only remained reduced in the HVD trial after 1 h of the exercise. Although not significant, β-cell function (HOMA-β) (approximately 94, P = 0·07 intra-group) showed a trend to decline in the HVD trial, reaching a significant between-group difference 1 h post-RE. Our results agree with the notion that the function of β-cell does not significantly change during an intense session and immediately after an exercise session(Reference Lloyd, Evans and Zerfass15,Reference Bordenave, Brandou and Manetta26) . Koopman et al. examined the effect of an intense session of RE on insulin resistance. According to the study, BS and insulin levels did not change significantly during 24 h after an exercise, but insulin sensitivity improved following RT(Reference Koopman, Manders and Zorenc27). In another study, Magkos et al. reported that a session of RT improved insulin resistance through the reduction in HOMA-IR index, and this reduction was observed in insulin and blood glucose levels(Reference Magkos, Tsekouras and Kavouras28). The researchers noted that the reduction of insulin resistance is associated with energy expenditure during exercise(Reference Lloyd, Evans and Zerfass15). GLUT-4 protein expression plays an important role in skeletal muscle capacity to stimulate insulin-dependent glucose transport(Reference Henriksen, Bourey and Rodnick29). It seems that overexpression of this protein in a prolonged exercise session can cause the declining effects of insulin resistance; and the impacts of improvement in insulin resistance during a single bout of RE are related to increase in GLUT4 translocation(Reference Stockton, Mengersen and Paratz30). Following this translocation, membranous appearance of the proteins will be significantly increased. It has been previously shown that vitamin D supplementation and vitamin D levels could play a role in improving insulin sensitivity(Reference Teegarden and Donkin31). While based on our literature search, no studies have investigated the effect of supplementation with vitamin D on the metabolic response and insulin resistance during a RE session, it seems that moderating effects of the vitamin D are associated with nuclear receptor of this vitamin(Reference Stockton, Mengersen and Paratz30).
LDH and CK isoenzymes are muscle injury biomarkers(Reference Noakes32). Various studies have shown that after intense exercise, changes in serum levels of enzymes and muscle isoenzymes in athletes and normal individuals can be observed(Reference Priest, Oei and Moorehead33,Reference Brancaccio, Maffulli and Limongelli34) . An increase in the activity of CK may be caused by metabolic and mechanical reasons. In fact, metabolically exhausted muscle fibres show a reduction in resistance of membrane after an increase in free intracellular Ca ions that stimulate the activation of K channels. Other mechanisms could be the localised tissue damage with the sarcomeric destruction of the Z line(Reference Brancaccio, Maffulli and Limongelli34). In our study, there was also a significant increase in CK activity in both conditions immediately after and 1 h after exercise compared with baseline. Unlike CK that increased within 1 h following exercise, LDH activity was declined after exercise. While the LDH activity was reduced after 1 h compared with immediately after RE in both trials, only on the LVD, the LDH activity recovered back to baseline. Based on our results, the increased CK activity was positively associated with RE intensity (8RM load). This relationship between RE intensity and CK changes was not observed in LDH. Rodrigues et al. reported that after a session of the RT, CK activity increased for 48 h and went down 72 h after the exercise. In the present study, the level of LDH activity did not change significantly(Reference Rodrigues, Dantas and de Salles35). According to the study by Güzel et al., the CK activity increased immediately after RT and will be increased continuously until 24 h then will be reduced(Reference Güzel, Hazar and Erbas36). Although the role of vitamin D supplementation on muscle tissue function is well known(Reference Bischoff, Borchers and Gudat37), effects of this supplementation on muscle damage during exercise have been less studied. In the only study conducted to date, Choi et al. reported vitamin D supplementation in rats with muscle damage induced by high-intensity aerobic exercise (30 min/d and 5 d/week for 8 weeks) that could decrease the CK activity compared with the control group and its rate statistically reached a level similar to a non-exercise group as well(Reference Choi, Park and Cho13). Discrepancies between this observation and our results may be related to a higher dose of vitamin D3 (25 μg/kg per d vitamin D3) and a longer duration of the exercise protocol in the study by Choi et al.(Reference Choi, Park and Cho13). Furthermore, animal studies are often poor predictors of human outcomes(Reference Bracken38). Nevertheless, future studies evaluating muscle damage in vitamin D-deficient individuals after oral or intramuscular vitamin D3 should focus on using higher doses and longer duration than our present investigation.
Based on our results, a single injection of high-dose vitamin D3 did not improve inflammatory response to a RT session. Although the immunomodulatory effect of vitamin D is well known(Reference Guillot, Semerano and Saidenberg-Kermanac’h4), this is the first study to evaluate the effect of a single dose of vitamin D3 injection on inflammatory response following an acute RE session. In agreement with our findings, an 8-week intervention of aerobic exercise combined with vitamin D supplementation in rats produced no changes in serum levels of IL-6 and TNF-α. Although other anti-inflammatory effects, such as a reduction in the phosphorylation of AMP-activated protein kinase (MAPK) pathway, proteins (AMPK, p38, Extracellular signal-regulated protein kinases 1 and 2, IκB and IκB kinase) and expression of IL-6 and TNF-α were shown(Reference Choi, Park and Cho13).
Our findings also show that regardless of vitamin D level, a RT session could increase the level of IL-6 and the ratio of the IL-6:IL-10, but other inflammatory markers remain unchanged. Consistent with our results, Phillips et al. reported that the level of IL-6 immediately after RT increased and returned to the baseline following 6 h(Reference Phillips, Mitchell and Currie-Elolf39). In another study, there was no any change in the serum level of IL-1β, 2, 5, 6, 8, 10 and TNF-α following a RT session. However, the mRNA of IL-1β, IL-6, IL-8 and TNF-α was up-regulated(Reference Buford, Cooke and Willoughby40). It seems that increased levels of IL-6 are caused by contraction of muscle fibres, which induce different metabolic effects(Reference Pedersen, Steensberg and Schjerling41). Increased levels of IL-6 due to exercise cause the stimulation of lipolysis and fat oxidation and involvement in glucose homeostasis, particularly through the induction of glycogenosis(Reference Pedersen, Steensberg and Schjerling41). Based on our results, other inflammatory cytokines and index did not change significantly in different times in both conditions. This unchanging could be due to the biological role of IL-6, which based on it, increased levels of IL-6 induced by muscle contraction during intense exercise have strong anti-inflammatory effects and could cause a decrease in TNF-α, inhibition of the TNF-induced insulin resistance and an increase in the anti-inflammatory IL-1ra IL-10(Reference Petersen and Pedersen12,Reference Pedersen, Steensberg and Schjerling41) .
In the present study, similar changes showed in BP, HR and the rate pressure product at rest and in response to exercise were seen in both trials. Therefore, intervention by vitamin D had no effect on the cardiovascular profile. Our findings agree with previous systematic reviews and meta-analyses that do not support the use of vitamin D for the improvement of resting cardiovascular health and function, due to the inconsistent findings of interventional studies and the need for larger-scale studies(Reference El-Fakhri, McDevitt and Shaikh42–Reference Agbalalah, Hughes and Freeborn44). Therefore, our present outcomes reinforce the notion that vitamin D supplementation does not change resting cardiovascular markers. Moreover, our results add further information on this area as to the best of our knowledge, this is the first investigation to assess cardiovascular changes post-exercise after vitamin D level improvement.
Our subjects were resistance-trained healthy Iranian males. Previous studies in Iran and other Middle Eastern countries reported a high prevalence of vitamin D deficiency and insufficiency, despite a high level of sun exposure in that region of the world(Reference Hovsepian, Amini and Aminorroaya45–Reference Rahnavard, Eybpoosh and Homami47). For instance, the prevalence of vitamin D deficiency/insufficiency in adults was reported as 61·4 and 87·8 % in Kuwait and Saudi Arabia, respectively(Reference Gaafar and Badr48,Reference Ardawi, Sibiany and Bakhsh49) . The high prevalence of vitamin D deficiency in Iranians may be due to various factors such as the presence of different skin pigments within the population, low dietary vitamin D intake, clothing preference and genetic factors such as special vitamin D receptor polymorphisms. Vitamin D deficiency may also result from low Ca intakes, involved in the vitamin D catabolism(Reference Salek, Hashemipour and Aminorroaya50,Reference Ziaee, Javadi and Javadi51) . Importantly, our findings may have potential clinical implications not only in countries with low sun exposure but also in countries with elevated sunshine levels and a high prevalence of vitamin D deficiency, such as those in the Middle East.
Our study has some limitations such as a small sample size, although our power calculation was suitable to detect significant differences between groups in metabolic markers. Additional limitations include the short duration and the use of a single dose of vitamin D3, which warrants further studies with longer interventions and different doses of vitamin D3 with a focus on understanding the relationship between changes in metabolic and anti-inflammatory parameters after RE.
In conclusion, a single injection of vitamin D3 declined insulin and BS levels, improved insulin-resistance and β-cell function following RE in previously vitamin D-deficient resistance-trained males. On the other hand, single injections of 7500 μg vitamin D3 did not improve muscle damage and inflammatory response to a RT session. Furthermore, no significant changes in baseline (resting) metabolic, inflammatory and cardiovascular changes were detected after vitamin D level improvement in this population.
Acknowledgements
The authors thank all the subjects and the Diabetes Research Center for their kind cooperation on the biochemical tests.
This work was financially supported by grant CMRC-9412 from the Vice Chancellor for Research Affairs, Cellular and Molecular Research Center, of Ahvaz Jundishapur University of Medical Sciences.
D. A. L. conducted the experiments and wrote the paper; R. B. revised some minor comments throughout the paper; A. W. edited, interpreted data and wrote some content of the paper; A. K. and M. A. G. designed and concepted the study; I. M. performed statistical analysis; and S. A. M. and S. J. H. performed analysis and interpretation of data.
The authors declare no conflicts of interest.