Introduction
The quest for sustainable alternatives to traditional materials has led to the exploration of living organisms for the production of biodegradable material substitutes with low environmental impact (Crawford Reference Crawford, Mason and Sharr2022). This has resulted in materials that harness biological processes and research into living microorganisms for design purposes. Such organisms include biomineralizing algae, cellulose-producing bacteria, and fungi as binding agents and decomposers of waste products (Crawford Reference Crawford2023a). Mycelium in particular, the filamentous part of the fungus (Elsacker et al., Reference Elsacker, Vandelook and Peeters2023; Vandelook et al., Reference Vandelook, Elsacker, Van Wylick, De Laet and Peeters2021), has been explored for the purposes of creating bio-composites for building insulation (Kalika Reference Kalika2018), packaging (Holt et al. Reference Holt, Mcintyre, Flagg, Bayer, Wanjura and Pelletier2012), interior solutions (Crawford Reference Crawford, Crawford, Diniz, Beckett, Vanucchi and Swackhamer2023b; Sydor et al. Reference Sydor, Bonenberg, Doczekalska and Cofta2021), and structural construction materials (Alemu et al. Reference Alemu, Tafesse and Mondal2022). In more recent studies, the flexible nature of the pure mycelial mats has come to the forefront as a potential substitute for non-woven materials such as animal-derived leather and petroleum-based faux leather alternatives (Crawford Reference Crawford, Chakrabarti and Singh2023c; Crawford et al. Reference Crawford, Branco, Sheldon and Ebert2023). This has prompted a rise in research that investigates the development of these bio textiles and their associated properties, which this paper builds upon (Amobonye et al. Reference Amobonye, Lalung, Awasthi and Pillai2023; Raman et al. Reference Raman, Kim, Kim, Oh and Shin2022; Rathinamoorthy et al. Reference Rathinamoorthy, Sharmila Bharathi, Snehaa and Swetha2023b; Williams et al. Reference Williams, Cenian, Golsteijn, Morris and Scullin2022).
Environmental impacts of animal leather and petroleum-based leather
The environmental impact of animal-derived leather has been a subject of concern both in terms of the impact of animal farming and the subsequent leather treatment stages that transform the material into a resilient product. Despite the high environmental costs, leather continues to be a sought-after material with the leather industry predicted to grow to $738.61 billion by 2030 (Research Reference Research2023). Extensive treatment is necessary to transform hide into leather including manual processing of the hide and subsequent chemical treatment stages involved in tanning. The tanning process involves a preparatory stage where the hide is cleaned and treated in a base solution to rehydrate it and prepare it for tanning. The next stage involves tanning the pickled hide to alter the mechanical properties of the leather. This may involve the use of vegetable tanners or chrome tanners, which make the leather more durable. Following tanning, the leather hide has to undergo basification typically with the use of sodium bicarbonate, which allows for further treatments and liquoring, which prevents the fibers from fusing at later stages. Tanning methods may have varied associated costs, processing times and environmental impacts (Kefale et al. Reference Kefale, Kebede and Birlie2023). The pre-tanning and tanning processes are responsible for 80%–90% of pollution associated with animal leather production (Arvanitoyannis and Kassaveti Reference Arvanitoyannis and Kassaveti2008). Chromium tanning, in particular, is responsible for highly oxidizing pollution with heavy metals, which enters the environment through industrial waste streams (Tripathi et al. Reference Tripathi, Singh, Singh, Bala, Pathak, Singh, Chauhan and Singh2023).
As a response to the challenges of animal leather production and associated ethical concerns, petrochemical alternatives have gained popularity. In most instances, these faux leather products are made up of polyurethane (PU) and polyvinyl chloride (PVC). These materials are typically made of a textile lined with a plastic layer that creates an aesthetic appearance similar to that of animal leather. However, plastic-based faux leathers have environmental impacts associated with the extraction of fossil fuels (Kefale et al. Reference Kefale, Kebede and Birlie2023), long degradation spans (Mohanan et al. Reference Mohanan, Montazer, Sharma and Levin2020), and potential off-gassing risks (Čech et al. Reference Čech, Paschová, Gaff, Li and Kačík2023).
Mycelium as vegan leather
In comparison, mycelium materials offer a low-cost and environmentally sustainable alternative to some petroleum-based materials and a more sustainable and ethical alternative to animal-derived leather. Myco materials can be grown on a wide variety of agricultural and industrial organic waste or side streams. With greater uptake and scaling of production, these products have the potential to become more economically viable than established traditional materials and can be optimized to meet consumer demands. In this context, it is important to look for ways to optimize such materials both in terms of their cultivation as well as their material properties so as to be able to phase out damaging practices and processes. Various commercial companies have developed proprietary methods for the cultivation and treatment of such materials, including Mogu, MycoWorks, MycoTech, and Ecovative (Vandelook et al. Reference Vandelook, Elsacker, Van Wylick, De Laet and Peeters2021). However, in the context of the global ecological crisis, there is a rising need for open-source methods and material testing data to allow for greater uptake and development. The products of commercial and open-source methods are now acquiring aesthetic qualities that are comparable to those of animal and PU leather and are hybridizing existing dyeing methods, including natural and synthetic options, to achieve similar results. These aesthetic treatments are demonstrated in this paper in conjunction with the myco-leather mats studied.
In most instances, mycelium-based leather is produced by growing the fungus as a biological tissue or mat on top of a liquid (Gandia et al. Reference Gandia, van den Brandhof, Appels and Jones2021) or solid substrate (Narayanamurthy et al. Reference Narayanamurthy, Ramachandra, Rai, Ganapathy, Kavitha and Manohara2008) or as fungal biomass in submerged liquid fermentation (Elsacker et al. Reference Elsacker, Vandelook and Peeters2023). Solid-state fermentation offers superior growth conditions, optimizing the rate of growth and offering a surface to which the hyphae can adhere whilst minimizing water consumption. However, liquid-state surface fermentation allows mycelium mats to be harvested more easily, yet growth rates are slower due to lower oxygen levels within the media. Lastly, liquid-state fermentation gives improved yields. However, the product must be further manipulated to produce a mycelium mat.
As a response to these challenges, in this study, we look at an alternative method for cultivation based on a paste consistency substrate that offers the benefits of high nutrient content as well as small nutrient particle size aiding the uptake of nutrients. This study further looks at species compatibility for the purposes of leather mat development by employing two fungal species. Namely, Ganoderma lucidum (reishi), a medicinal mushroom widely utilized within bio-design, and Pleurotus djamor (pink oyster), a gourmet mushroom that has the tendency to quickly colonize the substrate and enter the fruiting stage. Both species were chosen for their fast rate of colonization and wide availability, removing economic constraints and prohibitive cultivation timeframes. Furthermore, the paper looks at various post-treatment options as a way to improve the mechanical and aesthetic properties of the material and speculates as to alternative approaches for fabrication so as to improve the material’s tensile strength and open up a greater range of applications.
Method
This study was conducted on two distinct species of fungi: Ganoderma lucidum (reishi) and Pleurotus djamor (pink oyster). Farmer-grade grain spawn for each species was sourced from Liquid Fungi (liquidfungi.com). For each species, a total of ten mycelial mats were grown within aluminum foil trays with clear plastic lids, the trays measuring 20 cm × 20 cm. Each tray was covered with a 0.5 cm thick layer of sterilized paste growth media using a spatula in aseptic conditions. Each tray was then cultured with 3 g of grain spawn. The grain was evenly distributed to ensure equal coverage.
Growth paste media
For this experiment, a paste consistency growth media was prepared based on previous research Crawford et al. Reference Crawford, Branco, Sheldon and Ebert2023). The paste was prepared according to the following recipe: 450 ml deionized (D. I.) water, 165.5 g whole wheat flour, 2.5 g malt extract, 12.5 g xanthan gum, 1.25 g cream of tartar, and 0.2 g citric acid. The mixture was homogeneously mixed and placed within a mushroom growth bag with an air filter before autoclaving at 125 °C and 15 psi for a duration of an hour.
Incubation and monitoring
During the growth phase, the growth trays were incubated in complete darkness for 21 days at a temperature of 22 °C and relative humidity of 40%. During this period, samples were photographed every 48 hours.
Harvesting, sample preparation and data collection
Samples were peeled from the substrate using a spatula and gently rinsed from excess paste residue using tap water (Figure 1). Three strips measuring 3 cm × 9 cm and three squares measuring 2 cm2 were cut using a scalpel from the center of each mycelial mat immediately following harvesting. Weights were recorded for each sample and samples were split into sets containing three strips to be used for tensile testing and three squares for microscopy imaging. Samples were further weighed once dried following each subsequent treatment and drying phase. Sample thickness was also measured at the end of the full set of treatments for each set using a digital caliper.

Figure 1. (Left) Ganoderma lucidum (rishi) grown in aluminum trays with clear plastic lids, (Middle) Large mat of Ganoderma lucidum (rishi) being harvested via peeling, (Right) Mycelium mat after harvesting and rinsing of growth media residue.
Sample preparation and treatment
Eighteen sample sets were treated with glycerol at different concentrations (5%, 10%, and 20% w/w) for a duration of 24 hours and 48 hours to determine if soaking time significantly affected strip tensile strength. Some of these glycerol-treated samples were further subjected to a tanning process for either 6 or 24 hours using a commercial leather tanning solution by NuTan (sourced from advancedtanningsolutions.com). Further, eighteen sets were initially treated with citric acid at concentrations of 5 g/L, 10 g/L, and 15 g/L for a duration of 24 hours. Nine sets were further treated with varying concentrations of glycerol (10%, 20%, 30% w/w) for a duration of 48 hours. In addition, three sets were treated with the same concentrations of citric acid, 30% w/w glycerol, and magnesium sulfate of 10 g/L for 1 hour. A control was also prepared, which did not receive any treatment following harvesting. Each set contained triplicates. See Table 1 for a full list of post-treatment combinations.
Table 1. Post-treatments applied to sets of samples containing triplicates, (Yellow) initial glycerol treatment, (Green) initial citric acid treatment

To showcase the aesthetic potential of the material, independent samples that had undergone the same treatment as Set 17 were painted with acrylic leather paint or sprayed with blue algae solution prepared according to the recipe; 5 g blue spirulina microalgae powder per 250 ml water.
After each treatment, the samples were rinsed to remove any residual treatment solution. They were then dried for 72 hours prior to initiating the following stage of treatment. After the final drying period, all samples were heat-pressed for 220 seconds at 270°F, using a craft-grade heat press machine by WALA Press (sourced from www.heattransferwarehouse.com).
Tensile strength testing
Material testing was conducted using a Mxmoonfree Digital Force Gauge 500N with a manual wheel-operated test stand for tensile material testing (Figure 2). Maximum tensile strength of the samples was calculated according to the following equation:


Figure 2. Tensile testing of mycelium samples using Mxmoonfree Digital Force Gauge 500N.
TS = Ultimate Tensile Strength (N/cm2)
A = Area (cm2)
F = Ultimate Force (N)
The part of each sample tested measured 2.5 cm × 6 cm, for a total area of 15 cm2.
Microscopy
Scanning Electron Microscopy (SEM) was also performed to examine the microstructure of the samples. The SEM images were captured using a high-resolution scanning electron microscope. Images were further captured using AmScope SM-4TZ-144A Microscope and Barlow Lenses of magnification of 0.5X and 2.5X along with 18 MP camera.
Statistical analysis
The statistical analysis of the data collected in this study was performed using a variety of methods to ensure a comprehensive understanding of the results. Firstly, descriptive statistics were calculated for all quantitative data, including the various stages and tensile strength testing. An Analysis of Variance (ANOVA) was conducted to compare the means of the different treatment groups, ensuring that data sets met the assumptions of the ANOVA (normal distribution, equal variance and independence). One-way ANOVAs were performed to investigate the effect of one variable on the tensile strength, and two-way ANOVAs were performed to investigate the effects of two variables. If the ANOVA indicated a significant difference, a post-hoc test (Tukey’s HSD) was performed to identify which specific groups differed from each other.
The relationship between the different variables was also examined using correlation analysis. This helped to identify if there was a linear relationship between variables such as glycerol concentration and tensile strength. Finally, regression analysis was performed to compare tensile strength and one or more independent variables (e.g., glycerol concentration, tanning duration). All statistical analyses were performed using a significance level of 0.05. The data was analyzed using the statistical software RStudio (R version 4.2.1) using packages rstatix (v 0.7.2) and tidyverse (v 2.0.0).
Results
Species growth comparison
The initial growth study served to determine the viability of the fungal species for the purposes of growing a mycelial mat on a paste consistency media. Based on the development of the cultured trays, it became apparent that Pleurotus djamor (pink oyster) could not colonize the entire surface of the tray before contaminants took hold and it further failed to produce a mat with suitable thickness (Figure 3).

Figure 3. Pleurotus djamor (pink oyster) mat growing on paste media, Day 21, showing various levels of colonization and pining of the fruiting body.
In contrast, G. lucidum covered the majority of the surface area within the first week of growth (Figure 3), producing mats measuring 0.4–0.8 mm in thickness that could easily be detached from the paste substrate. Following treatments and heat pressing mat thickness ranged between 1.4 and 2.58 mm. Strips measuring 3 cm × 9 cm had an average initial wet weight of 5.99 g.
It is worth noting that the growth method employed resulted in grain from the mycelium spawn being embedded within the mycelial mat following harvesting, contributing to variations in tensile strength and level of homogeneity of the mycelial mat (Figure 4).

Figure 4. Ganoderma lucidum (reishi) leather mats growing on paste media until full surface colonization, (1) Day 0, (2) Day 4, (3) Day 7, (4) Day 14.
Visual analysis
Samples treated with higher glycerol percentage solutions presented greater flexibility in comparison with lower concentrations. Crosslinking agents impacted the color and flexibility of the samples with higher citric acid concentrations, resulting in warping and higher levels of rigidity, making them prone to breakage. Microscopy images collected with a stereo microscope show textural changes to the surface of the samples with tanned samples exhibiting a waxy surface, with greater color change compared to the control (Figure 5). This can be attributed to the tint present within the tanning product. Samples only treated with glycerol retained a surface aesthetic closer to the control. The subsequent color treatments were not visually impacted by the color alteration caused by the post-treatment (see Figures 8 and 9).

Figure 5. Mycelium samples include; A) and E) Set 10 untreated control, B) and F) Set 3 Glycerol 30% w/w (24 hours), C) and G) Glycerol 30% w/w (24 hours) + Tanner 6 hours, D) and H) Glycerol 30% w/w (24 hours) + Tanner 24 hours. Magnification levels shown in images A-D are 0.5X and 2.5X in images E–H.

Figure 6. SEM images captured at magnification on 5.00 k; A) Sat 4 Glycerol 10% w/w (24 hours) and NuTan Tanner (6 hours), B) Set 10 Control, C) Set 11 Glycerol 10% w/w (48 hours), D) Set 24 Glycerol 10% w/w (48 hours) Citric Acid 10 g/L (24 hours).

Figure 7. Ultimate tensile strength of post-treatments: Set 1 Glycerol 10% w/w (24 hours) (mean StDev = 0.278), Set 2 Glycerol 20% w/w (24 hours) (mean StDev = 0.152), Set 3 Glycerol 30% w/w (24 hours) (mean StDev = 261), Set 4 Glycerol 10% w/w (24 hours) + Tanner 6 hours (mean StDev = 0.183), Set 5 Glycerol 20% w/w (24 hours) + Tanner 6 hours, Set 6 Glycerol 30% w/w (24 hours) + Tanner 6 hours (mean StDev = 0.406), Set 7 Glycerol 10% w/w (24 hours) + Tanner 24 hours (mean StDev = 0.322), Set 8 Glycerol 20% w/w (24 hours) + Tanner 24 hours (mean StDev = 0.184), Set 9 Glycerol 30% w/w (24 hours) + Tanner 24 hours (mean StDev = 0.116), Set 10 None (Control), Set 11 Glycerol 10% w/w (48 hours) (mean StDev = 0.349), Set 12 Glycerol 20% w/w (48 hours) (mean StDev = 0.474), Set 13 Glycerol 30% w/w (48 hours) (mean StDev = 0.161), Set 14 Glycerol 10% w/w (48 hours) + Tanner (6 hours) (mean StDev = 0.384), Set 15 Glycerol 20% w/w (48 hours) + Tanner (6 hours) (mean StDev = 0.337), Set 16 Glycerol 30% w/w (48 hours) + Tanner (6 hours) (mean StDev = 0.143), Set 17 Glycerol 10% w/w (48 hours) + Tanner (24 hours) (mean StDev = 0.110), Set 18 Glycerol 20% w/w (48 hours) + Tanner (24 hours) (mean StDev = 0.0.098), Set 19 Glycerol 30% w/w (48 hours) + Tanner (24 hours) (mean StDev = 0.250), Set 20 Citric Acid 5 g/L w/w (24 hours) + 30% Glycerol + 10g Epsom/L (1 hr) (mean StDev = 0.297), Set 21 Citric Acid 10 g/L w/w (24 hours) + 30% Glycerol + 10 g Epsom/L (1 hr) (mean StDev = 0.307), Set 22 Citric Acid 15 g/L w/w (24 hours) + 30% Glycerol + 10 g Epsom/L (1 hr) (mean StDev = 0.068), Set 23 Citric Acid 5 g/L w/w (24 hours) + 10% Glycerol (mean StDev = 0.354), Set 24 Citric Acid 10 g/L w/w (24 hours) + 10% Glycerol (mean StDev = 0.403), Set 25 Citric Acid 15g/L w/w (24 hours) + 10% Glycerol (mean StDev = 0.090), Set 26 Citric Acid 5 g/L w/w (24 hours) + 20% Glycerol (mean StDev = 0.555), Set 27 Citric Acid 10 g/L w/w (24 hours) + 20% Glycerol (mean StDev = 0.071), Set 28 Citric Acid 15 g/L w/w (24 hours) + 20% Glycerol (mean StDev = 0.061), Set 29 Citric Acid 5 g/L w/w (24 hours) + 30% Glycerol (mean StDev = 0.366), Set 30 Citric Acid 10 g/L w/w (24 hours) + 30% Glycerol (mean StDev = 0.284), Set 31 Citric Acid 15 g/L w/w (24 hours) + 30% Glycerol (mean StDev = 0.191).

Figure 8. Tapestry of mycelium leather pieces measuring 12 cm × 12 cm treated with acrylic paint.

Figure 9. Mycelium leather samples measuring 30 cm × 48 cm grown using Ecovative living material, treated with blue algae solution using a spray-on application.
Based on the captured SEM data there is a visible difference in the structure of the mycelial, with untreated control samples and samples treated for a duration of 24 hours with glycerol exhibiting a continuous surface texture without visible strands. The cracking of the samples captured in images A) and B) is likely due to the collision of the electrons with the surface of the sample. Samples treated for 48 hours with glycerol exhibit individual hyphae structures, likely due to the loosening of the fibers, which is also in line with the greater flexibility of samples treated for longer periods of time with glycerol. Image C) and D) also differ in the thickness of individual strands with Set 24 exhibiting thicker hyphal structures reflective of the higher tensile strength in comparison to Set 11 (see Figure 6).
Ultimate tensile strength in relation to post-treatments
In the first instance, Sets 1–3 tested the effects of glycerol concentration along with the control group (Set 10), which the study established was not a significant factor in relation to tensile strength (Two-way ANOVA, n = 9, d.f. = 8, F = 1.049, P = 0.422). However, the samples treated with higher concentrations of glycerol exhibited higher levels of flexibility and maintained a sticky feel to the touch. The same was true for soaking time (Two-way ANOVA, n = 9, d.f. = 12, F = 0.071, P = 0.794), and interaction between soaking time and glycerol concentration in Sets 1–3 and Sets 11–13 (Two-way ANOVA, n = 18, d.f. = 12, F = 0.300, P = 0.746). However, a longer soaking time of 48 hours did increase the flexibility of the samples (Figure 7).
The difference of glycerol concentration in relation to NuTan treatment for 6 hours also did not have a significant effect (ANOVA, n = 9, d.f. = 6, F = 3.519, P = 0.097). However, glycerol concentration in relation to NuTan treatment with a 24-hour soaking time was a significant factor (ANOVA, n = 9, d.f. = 6, F = 16.787, P = 0.003). Tanning time was also not a significant factor (Two-way ANOVA, n = 18, d.f. = 12, F = 0.853, P = 0.450).
Citric acid concentration was not a significant factor in relation to tensile strength (ANOVA, n = 27, d.f. = 18, F = 0.354, P = 0.707). However, glycerol concentration was a significant factor when applied following a citric acid bath (Two-way ANOVA, n = 27, d.f. = 18, F = 4.995, P = 0.019). There was no significant interaction between concentration of citric acid and concentration of glycerol (Two-way ANOVA, n = 27, d.f. = 18, F = 1.377, P = 0.281).
Treatment with magnesium sulfate did not significantly impact the tensile strength of the samples. However, the level of flexibility and textural changes to the material following a magnesium sulfate bath produced a superior product, as flexibility and texture were akin to widely recognizable textile behaviors. The treatment dried out any excess glycerol residue and improved the degree of flexibility, resulting in a material less prone to breakage or permanent deformation.
Discussion
Using a paste consistency growth media provides benefits in relation to growing thicker mycelial mats over a shorter period of time in comparison to growth on nutrient-enriched agar or liquid culture as shown in previous studies (Crawford Reference Crawford, Chakrabarti and Singh2023c; Crawford et al. Reference Crawford, Branco, Sheldon and Ebert2023). It provides a further benefit during the harvesting stage as mats are peeled without the need to cut the sheet, as is the case with some solid-state growth methods (Williams et al. Reference Williams, Cenian, Golsteijn, Morris and Scullin2022). However, the flour-based paste mixture is prone to contamination from dormant spores that survive the sterilization process. Therefore, it is only suitable for fast-colonizing species that can colonize the surface before contaminants can take hold. This makes the paste method of cultivation particular to species that grow horizontally and form an even mycelial mat, as shown in the case of Ganoderma lucidum, but becomes problematic in the instance of Pleurotus djamor as the growth starts off thin and patchy and localizes in areas where the mat begins to enter a mushroom pinning phase. This also points to the necessity for using spawn rather than liquid culture due to its ability to colonize the surface quicker, resulting in a thicker mat than liquid culture or agar-grown culture (Crawford Reference Crawford2023a; Rathinamoorthy et al. Reference Rathinamoorthy, Bharathi, Snehaa and Swetha2023a). The average wet thicknesses being 9, 1.8, and 1.1 mm, respectively after a 21-day period. Further development of the paste substrate is also key to scaling and commercialization as a growth media reliant on low-grade or waste materials will make the cultivation method more sustainable and economically viable (Attias et al. Reference Attias, Danai, Tarazi, Pereman and Grobman2019; Elsacker et al. Reference Elsacker, Vandelook, Van Wylick, Ruytinx, De Laet and Peeters2020; Sydor et al. Reference Sydor, Bonenberg, Doczekalska and Cofta2021; Udayanga and Miriyagalla Reference Udayanga and Miriyagalla2021).
The presented method adds to existing methods and studies to assess economical means of cultivating such products. Alternatives include 5% ethylene glycol, and 20% polyethylene glycol as plasticizers and 20% corn zein and 5% tannic acid as crosslinking agents as discussed by Raman et al. (Reference Raman, Kim, Kim, Oh and Shin2022). The study also builds upon an existing body of work utilizing as glycerol as a plasticizer and assesses concentration and soaking time in relation to tensile strength. Examples include 20% v/v glycerol and a duration of 1 hour as highlighted by Wijayarathna et al. (Reference Wijayarathna, Mohammadkhani, Soufiani, Adolfsson, Ferreira, Hakkarainen, Berglund, Heinmaa, Root and Zamani2022).
The difficulties encountered in creating higher tensile strength using various post-treatments can be mitigated using mechanical approaches to textile product development as evidenced by other products on the market (Čech et al. Reference Čech, Paschová, Gaff, Li and Kačík2023; Williams et al. Reference Williams, Cenian, Golsteijn, Morris and Scullin2022). This may range from introducing a textile as a backing material that gives the mycelium leather the tensile strength necessary for commercial implementation for purposes associated with textile use, ranging from apparel to architectural tensile structures and soft furnishes as is the case with mess durable materials (Sheikhi et al. Reference Sheikhi, Xie, Li and Gürgen2024). This can be achieved during the growth phase by introducing layers of compatible textiles during various stages of cultivation. This may be a single layer or multiple layers introduced at intervals, allowing the hyphae to grow in between the woven fibers, fusing the layers within the structure of the material. Alternatively, a textile layer can be applied after the material has been treated using an adhesive (Wang et al. Reference Wang, Li and Cao2022).
There is a potential for the tensile strength of the material to be improved via the lamination of multiple layers and further surface treatments, such as the introduction of PLA coating, as is in the case of Mycoworks’ leather products and various dyes and veneers (Scullin et al. Reference Scullin, Wenner, Chase, Miller and Ross2020). Alternative applications where tensile strength is not a consideration are still possible in the pure material form. These may include surface covers that do not involve high contact, such as wall and ceiling finishes and applications over scaffolding structures (Crawford Reference Crawford, Crawford, Diniz, Beckett, Vanucchi and Swackhamer2023b; Manan et al. Reference Manan, Atta, Shahzad, Ul-Islam, Ullah and Yang2022; Sydor et al. Reference Sydor, Bonenberg, Doczekalska and Cofta2021).
The material appearance has the potential to be altered beyond the natural color and textural changes produced by the above-tested post-treatments for aesthetic purposes. As shown in Figure 8 the material can be treated with acrylic dyes producing an effect similar to widely available products on the market or through bio-dyes as shown in Figure 9 where the translucency of the dye preserves a remanence of the natural texture and color variations. Therefore, research into sustainable pigments becomes key in making myco-textiles viable commercial products (Hasanah and Islam Reference Hasanah and Islam2024; Rather et al. Reference Rather, Shabbir, Ganie, Zhou, Singh and Li2023; Roy Reference Roy and Roy2023; Zannat et al. Reference Zannat, Uddin, Mahmud, Mia and Ahmed2024).
Aesthetic qualities can also be affected by pressure and heat treatments such as embossing or heat pressing or by the aggregate size used to culture the growth media. For example, sheets grown with Ecovative living material, which features long timber shavings, produce a rough textured material in comparison to paste inoculated with liquid culture or mycelium propagated on agar, which produces a perfectly smooth sheet (Figure 10).
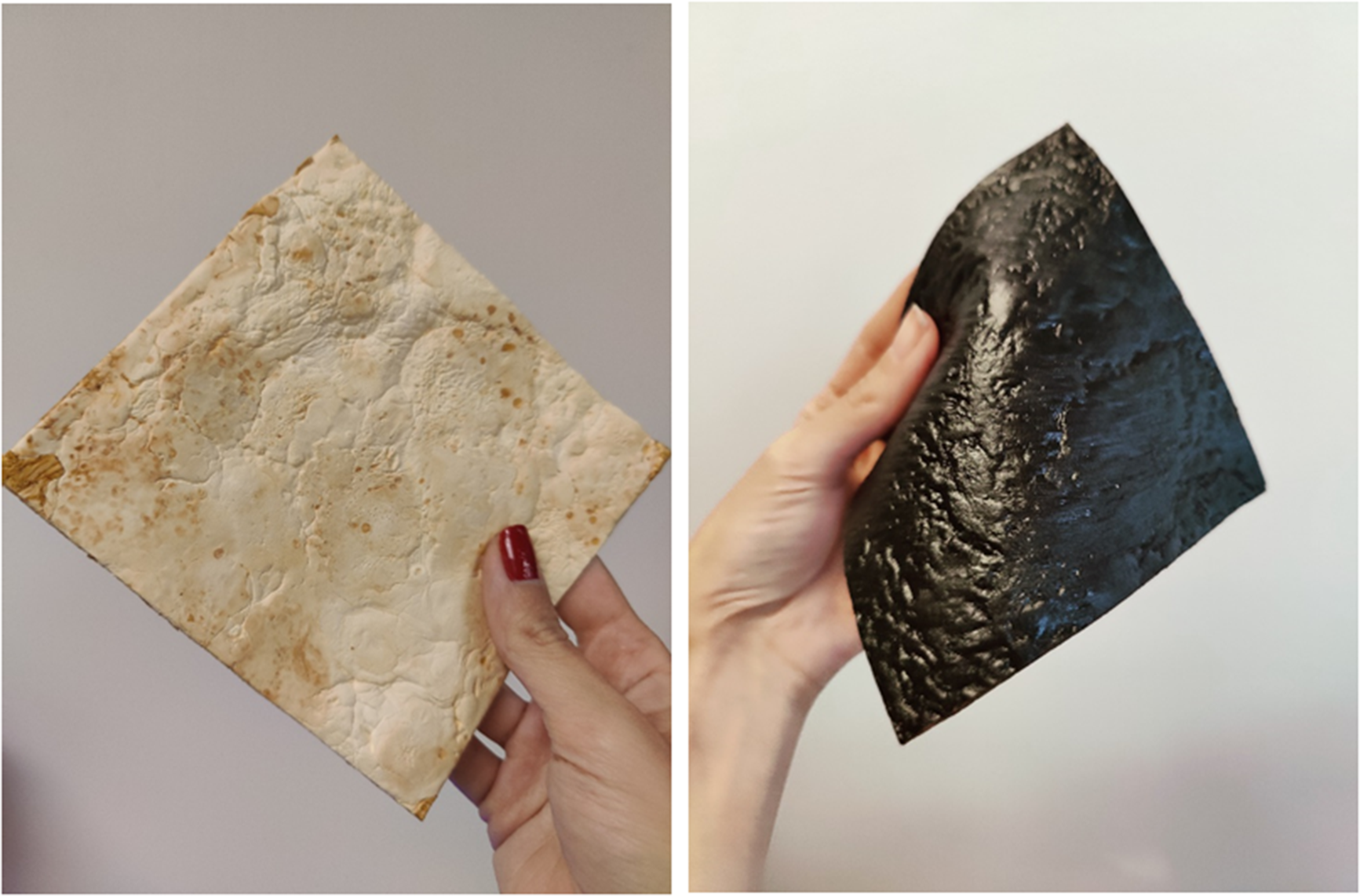
Figure 10. (Left) Mycelium leather grown using agar culture on paste, treated with 10% glycerol for 72 hours and heat-pressed, (Right) Same treatment with black leather dye.
Further testing is necessary to quantify the level of flexibility and to create a pallet of fungal species suited to paste media cultivation. Scaling of the material also poses challenges as the growth media is susceptible to contamination and necessitates sterile growth conditions for culturing and incubation. Currently, the largest sheets cultivated measuring 70 cm × 40 cm. This points to the need for a large-scale growth environment or the need for myco-welding development techniques that have the ability to fuse smaller sheets into larger areas.
Conclusion
The paper outlines a method for growing mycelium leather substitutes on a paste media using two fungal species with varying degrees of success. The potential for this method of cultivation opens up a possibility for faster cultivation and ease of harvesting. The study looks at a variety of post-treatment methods for Ganoderma lucidum mycelial mats that offer an improved level of tensile strength, although not comparable with faux leather or animal leather products. The study demonstrate the superior tensile strength exhibited by samples treated at a lower concentration for a 48 hour period. The paper proposes alternative approaches to tackle low tensile strength and suggests a range of applications within the built environment where mycelium leather with the above properties can be reinforced using a variety of strategies to achieve greater tensile strength and more desirable material properties.
Data availability statement
Data available on request from the authors.
Financial support
This study was funded by the Office of Research Services, University of Colorado Denver.
Competing interests
The authors declare that there are no conflicts of interest.
Ethics statement
Ethical approval and consent are not relevant to this article type.
Comments
No accompanying comment.