The liver is a primary organ of energy metabolism and a key site for detoxification of exogenous toxic substances like lipopolysaccharide (LPS)(Reference Nakao, Taki and Yasui1). LPS is a part of the outer surface membrane of gram-negative bacteria(Reference Zhao, Wu and Yi2). Gram-negative bacteria invasion is one of the main causes of weaning stress in piglets. In weaning stress condition, the gram-negative bacteria can release LPS when adhering to the small intestinal epithelium. Then LPS enters into the liver through intestinal epithelium in infected piglets(Reference Shen, Han and Zheng3). LPS in the liver stimulates Kupffer cells to active the STAT3 pathway, which produces proinflammatory cytokines such as interleukin-6 (IL-6) and transforming growth factor-beta 1 (TGF-β1)(Reference Ding, Zhang and Su4). Excessive TGF-β1 activates hepatic stellate cells to release α-smooth muscle actin (α-SMA) through mediating Smads pathway, which further promotes the progression of hepatic fibrosis(Reference Dai, Hu and Niu5). Ultimately, hepatic fibrosis perturbs disordered liver metabolism and retards growth(Reference Kyritsi, Kennedy and Meadows6). Notably, LPS challenge induced by weaning stress is an important cause of inflammatory liver injury and the death of the pig that creates great economic losses in livestock production worldwide(Reference Mani, Weber and Baumgard7). Nutritional interventions have been shown effective in alleviating liver inflammation and preventing liver fibrosis progression induced by LPS(Reference Zhang, Xu and Zhu8).
Pyrroloquinoline quinone (PQQ) is a bacterial redox cofactor that can enhance immunological functions in animals(Reference Killgore, Smidt and Duich9). Diets supplemented with PQQ and fed to obese mice during pregnancy provided a protection against hepatic lipotoxicity and inflammation in dams(Reference Jonscher, Stewart and Alfonso-Garcia10) and attenuate liver fibrosis(Reference Friedman, Dobrinskikh and Alfonso-Garcia11) in their offspring. PQQ supplementation suppresses murine liver fibrosis induced by thioacetamide(Reference Jia, Duan and Peng12) and attenuates liver inflammation via PPAR/STAT3 signalling during liver ischaemia and reperfusion injury in mice(Reference Zhou, Sun and Zhong13). However, few researches focused on the influence of PQQ supplementation on hepatic health in weaned piglets.
We have reported recently that diet supplemented with 3 mg/kg of diet PQQ decreases expression of inflammatory factors(Reference Yin, Ming and Bai14) and regulates glycolipid metabolism via AMPK phosphorylation(Reference Huang, Shi and Li15) in intestine of piglets during weaning. What is more, diet supplemented with 3 mg/kg PQQ attenuated inflammatory injury by inhibiting NF-κB signalling in jejunum of piglets challenged with enterotoxigenic Escherichia coli (Reference Huang, Ming and Wang16). To extend these findings, we hypothesise that PQQ supplementation can ameliorate liver inflammation, suppress progression of hepatic fibrosis and ultimately regulate energy metabolism in liver. In the present study, we investigate the effect of PQQ-supplemented diets on liver morphology and inflammatory injury together with hepatic energy metabolism in piglets challenged with LPS.
Materials and methods
Experimental
Crossbred barrows (n= 72, Duroc × Landrace × Yorkshire) were weaned at 23 (sem 2) d of age and with initial body weight of 7·2 (sem 0·65) kg. Piglets in each replicate were housed individually in a pen (1·80 × 1·10 m) with free access to feed and water in a well-ventilated, environmentally controlled (29 (sem 2)°C) nursery room. Piglets were fed the same dietary treatments, either basal diet or basal diet supplemented with 3 mg/kg PQQ disodium (purity > 98 %). The basal diet without antibiotics was formulated according to the National Research Council (2012) requirements (Table 1), and dosage of PQQ used was determined by our previous study(Reference Huang, Ming and Wang16).
Table 1. Ingredient composition and nutrient levels of the experimental diets (%, as-fed basis)
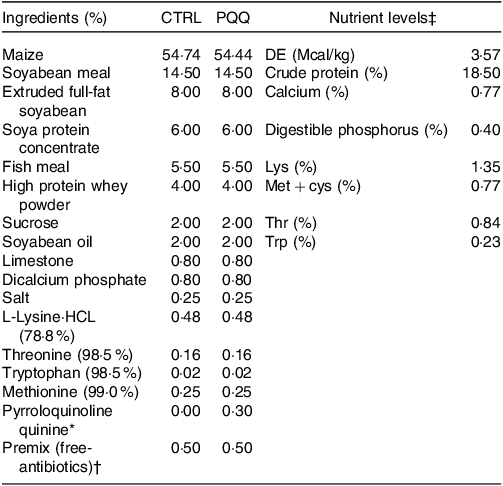
* The PQQ·Na2 (purity, ≥ 98 %) was synthesised by chemical reactions and donated by the Changmao Biochemical Engineering Company (Changzhou, China), and was diluted with maize starch to a concentration of 1 g/kg mixture, and then blended proportionally into a premix before being added to the diet.
† Premix provided the following per kg: vitamin A, 10 000 μg; vitamin D3, 2300 μg; vitamin E, 25 μg; vitamin K3, 2·40 mg; thiamine, 2·0 mg; riboflavin, 4·0 mg; pyridoxine, 3·0 mg; vitamin B12, 12 μg; niacin, 30 mg; pantothenic acid, 13 mg; folic acid, 1 mg; biotin, 50 μg; Fe, 9 mg; Cu, 20 mg; Zn, 90 mg; Mn, 20 mg; I, 0·35 mg; Se, 0·3 mg.
‡ The crude protein (CP) and Ca content are analytical value; however, the digestible energy (DE), digestible phosphorus, Lys, Met + cys, Thr and Trp value are calculated value.
These piglets were in a 2 × 2 factorial design and randomly divided into four groups with six replicates per group and three piglets per replicate as follows: (1) piglets that received basal diet and were not injected with LPS (CTRL group); (2) piglets that received the basal diet supplemented with 3 mg/kg of diet PQQ and were not injected with LPS (PQQ group); (3) piglets that received basal diet and were injected with LPS (CTRL + LPS group) and (4) piglets that received the basal diet supplemented with 3 mg/kg PQQ and were injected with LPS (PQQ + LPS group).
The challenge trial was conducted at 08.00 h on days 7, 11 and 14 of the experiment, piglets from CTRL + LPS group and PQQ + LPS group were received intraperitoneal injection of Escherichia coli Lipopolysaccharide (LPS, E. coli serotype 055:B5; Sigma Chemical) at a dose of 80 μg/kg body weight and others were offered equivalent amount vehicle (0·9 % NaCl). Three injections were chosen according to the previous study with minor modifications(Reference Duan, Song and Zheng17,Reference Wang, Hou and Yi18) . And the dose of LPS was set according to the previous research(Reference Duan, Zheng and Zhong19).
Blood was sampled from the anterior vena cava into 10-ml vacuum tubes without anticoagulant (Becton Dickinson Vacutainer System) after piglets being overnight starvation for 12 h. Then the blood was centrifuged at 3000 × g at 4°C for 15 min to separate serum. And the piglets were slaughtered for harvesting liver tissue at 4 h after LPS injection at day 14. A block of tissue (about 1 cm3) was fixed in 4 % neutral-buffered paraformaldehyde for analysis of liver morphology. Another block (2 g) was removed from the right hepatic lobe, snap-frozen in liquid N2 and stored at –80°C for further analysis.
Hepatic morphology
Haematoxylin and eosin (HE) staining of liver was assayed in accordance with our previous study(Reference Huang, Wang and He20). In brief, after 24 h of fixation with 4 % paraformaldehyde solution, liver segments were dehydrated and embedded in paraffin, sliced into 5 µm sections and stained with haematoxylin and eosin. Masson’s trichrome staining of hepatic sections was conducted according to product manuals from the Trichrome Stain (Masson) Kit (Sigma-Aldrich). Histological features including hepatic cells arranged, hepatic lobule structure, central veins and radial hepatocyte cords and inflammatory cell infiltration were captured by a light microscope (Olympus BX-51; Olympus).
Serum cytokines
Serum concentrations of IL-1β, IL-17, and IL-22 were detected by ELISA methods using commercial kits (Bioscience) according to the manufacturer’s instructions. Absorbance was measured at 450 nm. Minimal detection limit was 0·3 pg/ml for IL-1β, 15 pg/ml for IL-17 and 2·7 pg/ml for IL-22.
Serum concentrations of cyclooxygenase-2, aspartate aminotransferase (AST), alanine transaminase (ALT), HDL-cholesterol, total cholesterol (TC) and cortisol were determined by commercial colorimetric kits according to kit manuals (Abcam, Cambridge, MA, USA). Absorbances were measured at 450 nm for cyclooxygenase-2, AST and cortisol, 510 nm for TC and 570 nm for HDL-cholesterol and ALT. Minimal limits of detections were 1·2 U/L for cyclooxygenase-2, 10 U/L for AST, 0·12 U/L for cortisol, 0·25 U/L for ALT and 0·1 mmol/ml for HDL-cholesterol. The intra-assay CV was < 10 % and inter-assay CV was < 12 % for each assay.
Quantitative real-time PCR
Total RNA was isolated from hepatic tissues using the Hipure Total RNA Mini Kit (Magen Co, Guangzhou, China). And cDNA was converted using the PrimeScript RT reagent Kit (TaKaRa Bio Inc) according to the manufacturer’s instructions. Quantitative real-time PCR was conducted using the SYBER Green Supermix (TaKaRa Bio Inc) in an Analytik Jena qTOWER 2·2 real-time PCR system (Analytik Jena). The primers for IL-17, IL-4, IL-10, IL-6, NF-κB, p38, ATP-binding cassette transporter A1 (ABCA1), signal transducer and activator of transcription-3 (STAT3), TGF-β1, Smad2, Smad3, Smad4, stearoyl-CoA desaturase 1 (SCD1), PPARγ and carnitine palmitoyltransferase-1 (CPT-1) are shown in Table 2. Each gene was determined three times in each sample. Relative mRNA abundance of target genes was normalised with expression of β-actin and calculated by the 2−ΔΔCT method(Reference Pfaffl, Horgan and Dempfle21).
Table 2. Primer sequences of target and reference genes*
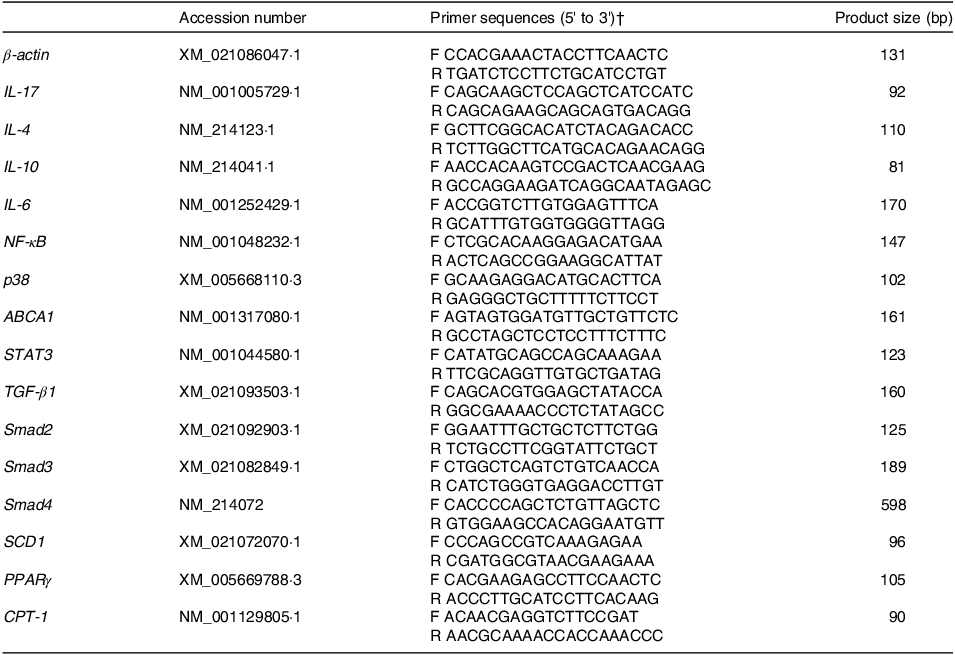
* The qPCR conditions were included one cycle at 94°C for 5 min, 40 cycles at 94°C for 30 s, annealing temperature for 30 s and 72°C for 30 s.
† F, forward primer; R, reverse primer.
Western blot analysis
Liver tissue samples were powdered and homogenised in RIPA buffer (HX1862, Huaxingbio Science, Beijing, China) and then centrifuged at 14 000 × g for 15 min at 4°C to collect supernatants. Protein concentration of supernatants was detected using the BCA method(Reference Smith, Krohn and Hermanson22). Proteins (80 μg) from each sample were loaded onto SDS polyacrylamide gels, blotted onto polyvinylidene difluoride membranes and blocked at room temperature for 1 h in blocking buffer with 5 % skimmed milk. Then the membranes were incubated with primary antibodies over night at 4°C. After three washes with 1 × TBST buffer, the PVDF membranes were incubated with DyLight 800-conjugated secondary antibody (#9145, Cell Signaling Technology, Danvers, Lincoln, 1:10 000) for 1 h. Band densities were measured with Odyssey Clx (LI-COR Biotechnology) and quantified using the Image J software. All protein expressions were normalised to β-actin, GAPDH or Tubulin. Primary antibodies used in the present study were: IL-22 (ab193813; Abcam, 1:500), STAT3 (#12640, Cell Signaling Technology, 1:1000), phosphorylated-STAT3(Tyr705) (p-STAT3(Tyr705), #9145, Cell Signaling Technology, 1:500), alpha-smooth muscle actin (α-SMA, ab5694, Abcam, 1:1000), adenosine 5‘-monophosphate-activated protein kinase (AMPK, #2795 Cell Signaling Technology, 1:1000) and phosphorylated-AMPK (p-AMPK, #2535, Cell Signaling Technology, 1:1000).
Statistical analysis
Normal distribution was validated data by QQ plot for the value of serum and liver cytokines, and mRNA and protein expression, and then the values were analysed by ANOVA using the GLM procedure of the SAS software (Version 9.2, SAS Institute). A 2 × 2 factorial design was conducted for data, and the statistical model included the effects of diet (basal diet v. PQQ diet), challenge (0·9 % NaCl v. LPS) and their interactions. Data were expressed as means ± pooled sem. Once a significant or a trend for diet × LPS interaction occurred, post hoc testing was conducted using Tukey’s range test. Statistical significance was set at P ≤ 0·05, a trend was considered when 0·05 < P < 0·10, and n= 6. The artworks were drawn by GraphPad Prism (version 6).
Results
Pyrroloquinoline quinone supplementation regulated expression of serum factors in weaned piglets challenged with lipopolysaccharide
There was a LPS challenge × PQQ diet interaction found for serum IL-1β, IL-22 and HDL-cholesterol (P < 0·05), and a trend for LPS challenge × PQQ diet interaction was observed for ALT (P = 0·05) and AST (P = 0·07). Compared with piglets in the CTRL group, serum concentration of inflammatory factors like IL-1β and IL-22 level, as well as liver-injury markers including ALT and AST was increased, and energy metabolism marker HDL-cholesterol was decreased in the CTRL + LPS group (P < 0·05, Table 3). The stimulatory effects of LPS on serum level of IL-1β, IL-22, ALT and AST were reduced and HDL-cholesterol were increased in the PQQ + LPS group (P < 0·05). Additionally, there were no LPS challenge × PQQ diet interactions found for serum IL-17, TC and cortisol. And there was no difference for these serum inflammatory factors and energy metabolism markers between CTRL and PQQ groups.
Table 3. Effects of PQQ supplemented on serum cytokines in weaned piglets challenged with LPS*
(Mean values with their standard error of the means)
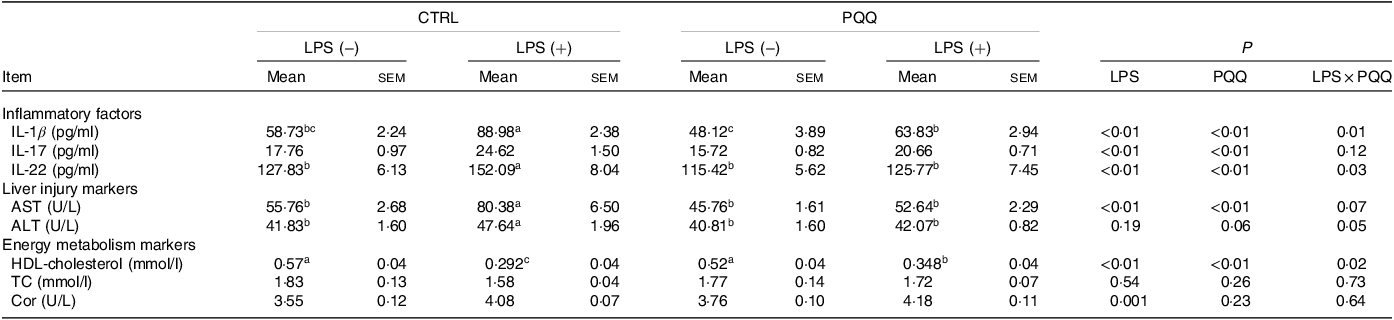
PQQ, pyrroloquinoline quinone; LPS, lipopolysaccharide; AST, aspartate aminotransferase; ALT, alanine transaminase; TC, total cholesterol; Cor, cortisol.
* Values are means ± sem; n= 6. Means within a row lacking a common letter are different (P < 0·05).
Pyrroloquinoline quinone supplementation improved liver morphology in weaned piglets challenged with lipopolysaccharide
Changes of liver morphology are presented in Fig. 1. In both CTRL and PQQ groups, hepatic cells of the piglets arranged neatly, the hepatic lobule structure was intact, central veins and radial hepatocyte cords were visible and there was little inflammatory cell infiltration in the liver tissue. By contrast, irregular lobular architecture with unclear central vein, disordered arrangement of hepatic cell cords, hepatocyte caryolysis, hepatocyte karyopycnosis and a large number of inflammatory cells infiltration were presented in the CTRL + LPS group; while in the PQQ + LPS group, normal lobular architecture with clear central vein and a small amount of inflammatory cell infiltration and hepatocyte caryolysis were showed in liver.
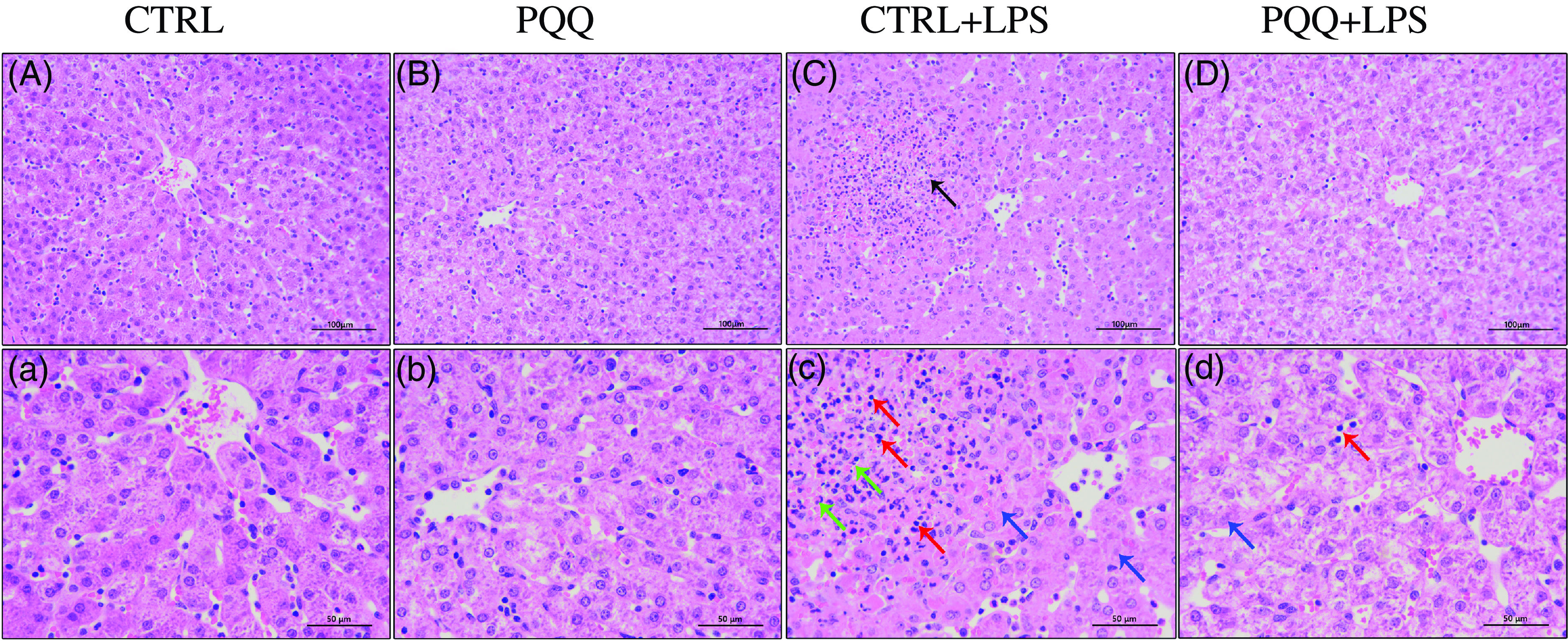
Fig. 1. PQQ supplementation regulated liver morphology in piglets challenged with LPS A–D, Haematoxylin–eosin staining was aim to evaluate the liver morphology, scale bar 100 μm. a–d, Haematoxylin–eosin staining was aimed to evaluate the liver morphology, scale bar 50 μm. Black arrow represents inflammatory lesion, red arrow represents infiltration of inflammatory cells, green arrow represents hepatocyte karyopycnosis, blue arrow represents hepatocyte caryolysis. CTRL, piglets received diet without PQQ and injected with 0·9 % NaCl; PQQ, received diet with PQQ and injected with 0·9 % NaCl; CTRL + LPS, piglets received diet without PQQ and injected with LPS; PQQ + LPS, received diet with PQQ and injected with LPS. n= 6. PQQ, pyrroloquinoline quinone; LPS, lipopolysaccharide.
Pyrroloquinoline quinone supplementation decreased mRNA and protein abundance of inflammatory pathway in weaned piglets challenged with lipopolysaccharide
There was a LPS challenge × PQQ diet interaction found for liver mRNA level of IL-6, IL-17 and STAT3 (Table 4), and protein level of IL-22 and p-STAT3 (Fig. 2, P < 0·05). Compared with piglets in the CTRL group, the mRNA expression of IL-6, IL-17 and STAT3 and protein expression of IL-22 and p-STAT3 were increased in piglets of the CTRL + LPS group (P < 0·05). Notably, compared with the CTRL + LPS group, the mRNA abundance of IL-6, IL-17 and STAT3 and protein level of IL-22 and p-STAT3 in liver were decreased in the PQQ + LPS group (P < 0·05). There was no LPS challenge × PQQ diet interaction found for liver mRNA level of IL-4, IL-10, NF-κB, p38 and protein expression of STAT3 among the four groups.
Table 4. Effects of PQQ supplementation on liver mRNA level of inflammatory cytokines and energy metabolism genes in piglets challenged with LPS*
(Mean values with their standard error of the means)
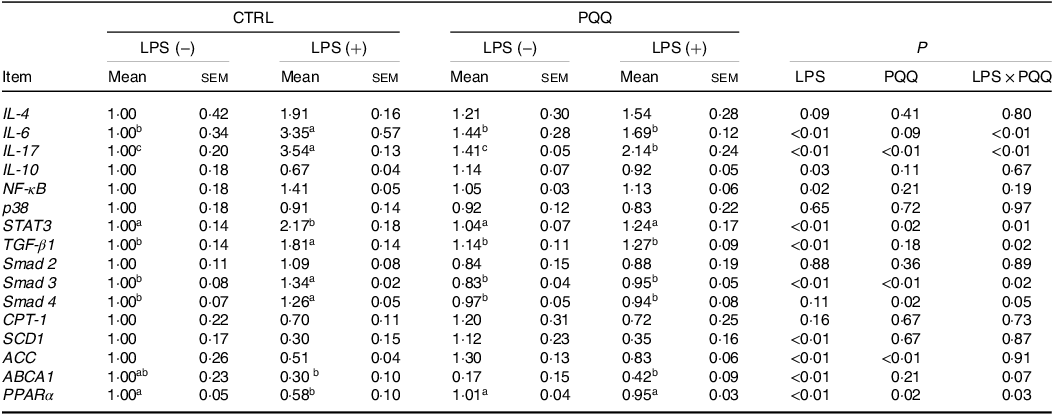
PQQ, pyrroloquinoline quinone; LPS, lipopolysaccharide.
* Values are means ± sem; n= 6. Means within a row lacking a common letter are different (P < 0·05). The relative mRNA abundance of the target genes was normalised with expression of β-actin.
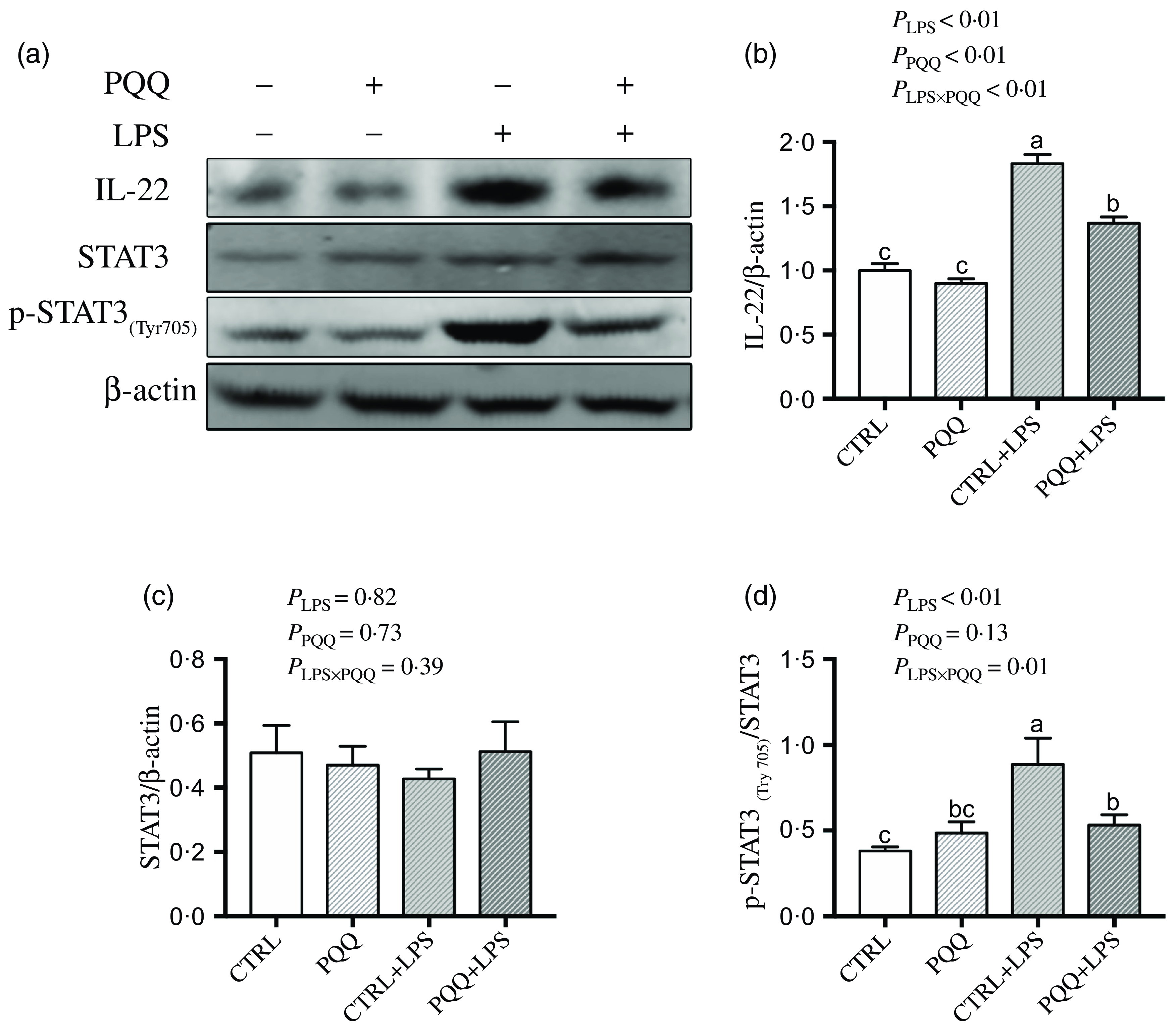
Fig. 2. PQQ supplementation regulated liver protein level of inflammatory pathways in piglets challenged with LPS. A, Protein levels of STAT3 and p-STAT3 in the liver, and the densitometric values were normalised to β-actin. B–D, Statistical analysis of the data in A. CTRL, piglets received diet without PQQ and injected with 0·9 % NaCl; PQQ, received diet with PQQ and injected with 0·9 % NaCl; CTRL + LPS, piglets received diet without PQQ and injected with LPS; PQQ + LPS, received diet with PQQ and injected with LPS. Means within a row lacking a common letter are significantly different (P < 0·05); n= 6. PQQ, pyrroloquinoline quinone; LPS, lipopolysaccharide.
Pyrroloquinoline quinone supplementation attenuated liver fibrosis and decreased the expression of fibrosis promoting factors in weaned piglets challenged with lipopolysaccharide
Masson staining showed fibrosis between the portal area and the interlobular area in liver of the piglets in the CTRL + LPS group compared with piglets in other three treatments (Fig. 3A–D and a–d).
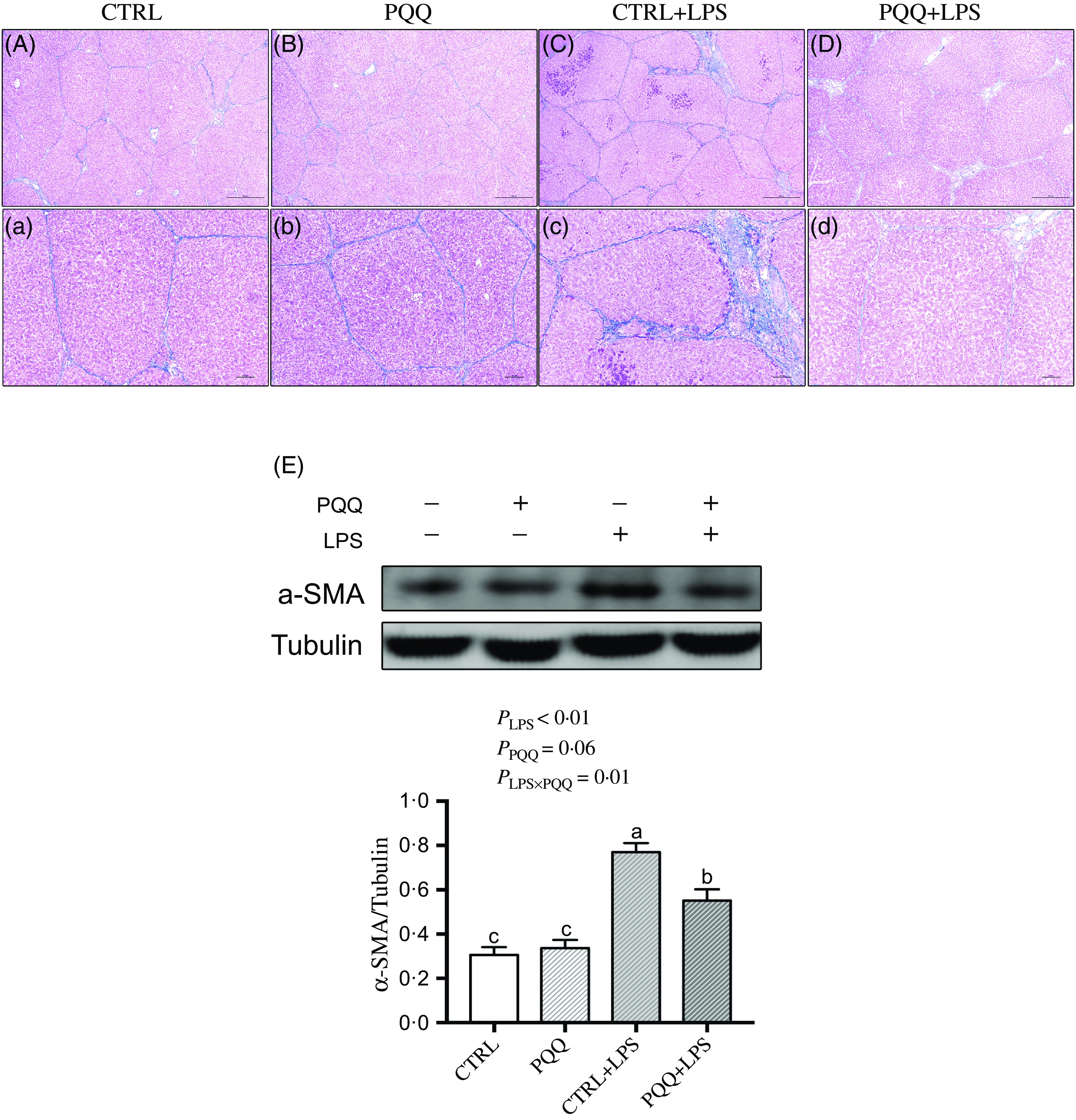
Fig. 3. PQQ supplementation regulated liver fibrosis in piglets challenged with LPS. A–D and a–d, Masson’s trichrome staining was aimed to evaluate the progress of liver fibrosis, scale bar in A–D was 500 μm, scale bar in a–d was 100 μm. E, Protein levels of α-SMA in the liver, and the densitometric values were normalised to tubulin. CTRL, piglets received diet without PQQ and injected with 0·9 % NaCl; PQQ, received diet with PQQ and injected with 0·9 % NaCl; CTRL + LPS, piglets received diet without PQQ and injected with LPS; PQQ + LPS, received diet with PQQ and injected with LPS. Means within a row lacking a common letter are significantly different (P < 0·05); n= 6. PQQ, pyrroloquinoline quinone; LPS, lipopolysaccharide.
Additionally, there was a LPS challenge × PQQ diet interaction found for liver mRNA level of TGF-β1, Smad3 (Table 4) and protein expression of α-SMA (Fig. 3E, P < 0·05), and a trend for LPS challenge × PQQ diet interaction was observed for Smad4 (P = 0·05). Compared with the CTRL group, the mRNA level of TGF-β1, smad3 and smad4 and protein abundance of α-SMA were increased in piglets from the CTRL + LPS group (P < 0·05). Compared with the CTRL + LPS group, the mRNA level of TGF-β1, smad3 and smad4 and protein abundance of α-SMA were decreased in the PQQ + LPS group (P < 0·05, Table 4).
Pyrroloquinoline quinone supplementation regulated liver energy metabolism in weaned piglets challenged with lipopolysaccharide
Next, we evaluated the expression of genes in energy metabolism pathways in liver. There was a LPS challenge × PQQ diet interaction found for liver mRNA level of PPARα (Table 4) and protein level of p-AMPK (Fig. 4, P < 0·05). Moreover, there was a trend for a LPS challenge × PQQ diet interaction for ABCA1 (P = 0·07). Compared with CTRL groups, the mRNA level of PPARα and the protein level of p-AMPK were decreased in liver of piglets from the CTRL + LPS group (P < 0·05). Interestingly, the down-regulated concentration of p-AMPK (Fig. 4) was attenuated in liver of the piglets from the PQQ + LPS group. There was no LPS challenge × PQQ diet interaction found for liver mRNA level of CPT-1, SCD1, ACC and protein level of AMPK.
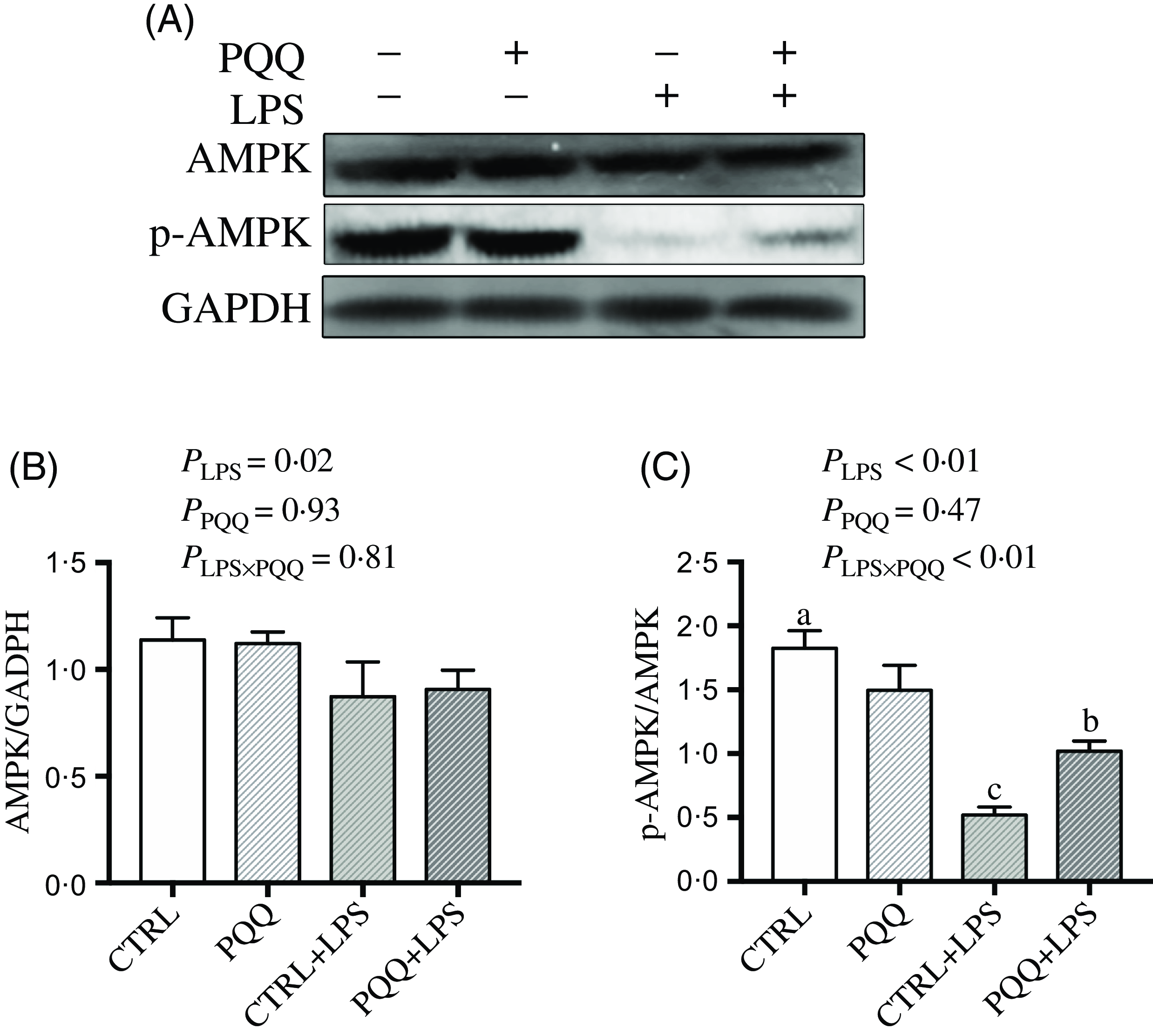
Fig. 4. PQQ supplementation regulated AMPK signalling in piglets challenged with LPS. A, Protein levels of AMPK and p-AMPK in the liver, and the densitometric values were normalised to GADPH. B and C, Statistical analysis of the data in A. CTRL, piglets received diet without PQQ and injected with 0·9 % NaCl; PQQ, received diet with PQQ and injected with 0·9 % NaCl; CTRL + LPS, piglets received diet without PQQ and injected with LPS; PQQ + LPS, received diet with PQQ and injected with LPS. Means within a row lacking a common letter are significantly different (P < 0·05); n= 6. PQQ, pyrroloquinoline quinone; LPS, lipopolysaccharide.
Discussion
Inflammation induced by LPS often causes liver and intestine injury, and then resultant growth retardation in piglets around weaning. Dietary PQQ supplementation in weaned piglets could decrease intestinal inflammatory injury(Reference Huang, Ming and Wang16,Reference Zhang, Li and Cao23) and regulate redox status and glycolipid metabolism as our previous studies(Reference Huang, Shi and Li15,Reference Huang, Fan and Han24) . However, few reports extend those results and focused on the effect of PQQ for liver injury in weaned piglets challenged with LPS.
Inflammatory liver injury is seen as damage to liver morphology with the appearance of lesions, such as cell degeneration, lymphocytic infiltration, and necrosis(Reference Yi, Hou and Wang25). In this research, after LPS challenged for 7 d, we found obvious inflammatory lesions, hepatocyte caryolysis, hepatocyte karyopycnosis and lymphocyte infiltration liver, which implied LPS challenge damaged liver integrity and was consistent with previous study(Reference Zhang, Xu and Zhu26). Key markers of liver injury(Reference Kunutsor, Apekey and Khan27) such as ALT and AST increased in serum of pig injection with LPS also were found in this study. Similar results have been described previously(Reference Wu, Liu and Pi28). Besides, liver inflammation induced by LPS often is associated with perturbed lipid metabolism(Reference Luo, Cheng and Du29–Reference Arner, Bernard and Appelsved31). Serum HDL-cholesterol and TC are important markers of liver lipid metabolism condition(Reference Gu, Yu and Liu32). In the present study, LPS challenge induced fever, anorexia, inactivity, shivering and reduced serum HDL-cholesterol and TC level on day 14. These results indicated LPS caused abnormal lipid metabolism and liver injury occurred. Therefore, we can reasonably deduce that LPS challenge induced inflammatory liver injury, and consequently damages liver abnormal lipid metabolism.
Liver inflammation stimulated by LPS that was caused primarily by activating Kupffer cells and lymphocytes(Reference Seki, Tsutsui and Nakano33) and stimulating macrophage division into proinflammatory (M1) and wound-healing (M2) types(Reference Mosser and Edwards34). Activated Kupffer cells and M1 macrophages release inflammatory cytokine, including IL-1β and IL-6(Reference Luster, Germolec and Yoshida35). Then IL-17 and IL-22 are produced mainly by lymphocytes, which are activated through transcription factors STAT3 phosphorylated via an IL-6 dependent way(Reference Ruiz de Morales, Puig and Daudén36). STAT3, as one of the most important signal transducers in STAT family, is inactive in all types of non-stimulated cells(Reference He and Karin37). Once stimulated, upstream Janus kinases transduce a signal to phosphorylate the tyrosine residue (Tyr705) of STAT3, which leads to p-STAT3(Tyr705) entering the nucleus to stimulate the production of proinflammatory cytokines(Reference Yu, Pardoll and Jove38). In our present study, proinflammatory cytokines level such as IL-1β, IL-6, IL-17 and IL-22, and p-STAT3(Tyr705) expression were increased either in serum or in liver of the piglets challenged with LPS, which indicates that liver inflammation occurred. Notably, these differentially expressed genes may represent promising candidates for understanding the mechanism underlying the inflammatory liver injury induced by LPS.
Hepatic inflammation caused by STAT3 pathway activation often is associated with activation of hepatic stellate cells, which lead to liver fibrosis(Reference Chakraborty, Šumová and Mallano39). As components of latent complex, TGF-β production is primarily mediated by STAT3(Reference Celada, Kropski and Herazo-Maya40). TGF-β1 is the predominantly expressed isoform for TGF-β and is an initiation factor that induces differentiation of hepatic stellate cells into myofibroblasts in mammals(Reference Wan and Flavell41). Elevated TGF-β1 in extracellular spaces binds to transmembrane TGF-β receptors and transmits a signal to phosphorylated Smad2 and Smad3. Oligomers are formed with activated Smad2, Smad3 and Smad4 and then translocated into the nucleus to release the fibrosis marker α-SMA(Reference Dai, Xu and Zhang42,Reference Yoshida43) . In the present study, we observed that expression of TGF-β1, Smads and α-SMA increased, and Masson’s trichrome staining showed fibrosis between portal and interlobular areas in liver of piglets challenged with LPS. These results illustrate the model of liver inflammation injury in piglets induced by LPS was established successfully.
PQQ supplementation improves liver health in mice(Reference Huang, Chen and Miao44), rats(Reference Singh, Pandey and Saha45) and hens(Reference Wang, Zhang and Xu46). In the present study, examined parameters (change of liver morphology, inflammatory indexes, fibrosis factors expression in serum and liver) were effectively attenuated after LPS injection in piglets whose diet was supplemented with PQQ. Therefore, we deduce that PQQ supplementation ameliorates liver damage by regulating hepatic inflammatory and fibrosis responses. LPS-induced proinflammatory cytokine elevation in liver was associated with NF-κB (Reference Baldwin47), p-38 (Reference Lu and Cederbaum48) and STAT3 (Reference Brenner, Galluzzi and Kepp49). In the present research, increased mRNA concentrations of ST AT3 but not NF-κB and p-38 were attenuated in diets supplemented with PQQ for piglets injected with LPS. Based on this result, we also found up-regulated protein expression of p-STAT3 was decreased in LPS-challenged liver of piglets fed PQQ diet. Thus, we confirmed PQQ reduced liver inflammation induced by LPS challenge in piglets might partly by inhibiting STAT3 signalling under the present experimental conditions. Notably, STAT3 signalling promotes hepatic stellate cells to differentiate into fibrogenic myofibroblasts by IL-17 stimulation(Reference Meng, Wang and Aoyama50). Presently, PQQ supplementation in liver was without obvious fibrosis lesions, and reduced levels of TGF-β1, Smads and α-SMA in piglets challenged with LPS. Therefore, we have demonstrated that PQQ inhibited progress of liver fibrosis in weaned piglets challenged with LPS, which was associated with activation of the STAT3/TGF-β1 signalling pathway. In our current study, regretfully, the expression of genes or proteins is detected to study the role of the STAT3/TGF-β1 pathway in inflammatory liver injury induced by LPS. If we use corresponding inhibitors or activators to block or activate this pathway and check the expression of downstream genes or proteins, adequate information would be provided to confirm their roles in LPS-induced liver injury, which awaits further experiments.
Accompanied by attenuating hepatic inflammation and fibrosis in diets supplemented with PQQ of piglets challenged with LPS, down-regulated lipid metabolism index was restored. Interestingly, lipid parameters are often related to activation of energy metabolism pathways in which AMPK is one kind of a metabolite-sensing protein kinase(Reference Kemp, Mitchelhill and Stapleton51). When AMPK is phosphorylated by upstream kinases(Reference Arner, Bernard and Appelsved52), it transduces a signal sensed by PPARα (Reference Bronner, Hertz and Bar-Tana53) and finally regulates serum levels of HDL-cholesterol(Reference Chinetti, Lestavel and Bocher54). In our research, we found decreased protein abundance of p-AMPK, and mRNA level of PPARα was up-regulated in piglets of the PQQ + LPS group compared with the CTRL + LPS group. These results suggested lipid metabolism was regulated in diet supplemented with PQQ of piglets after LPS injection.
Conclusions
In conclusion, combining results from serum and liver, dietary supplementation with PQQ alleviated liver inflammation and fibrosis, then regulating liver energy metabolism might partly via STAT3/TGF-β1 signalling in weaned piglets challenged with LPS. These findings contribute to better understand the effect and mechanism of PQQ on alleviating inflammatory liver injury in piglets and young animals.
Acknowledgements
The authors thank Prof. Shuai Zhang (State Key Lab of Animal Nutrition, College of Animal Science & Technology, China Agricultural University) for the guidance of statistical analysis in this manuscript.
This study was financially supported by the National Natural Science Foundation of China (32202681); the Natural Science Foundation of Fujian Province of China (2022J01131269); the Education and Scientific Research Project for Young and Middle-aged Teachers of Fujian Provincial Education Department (Science and Technology, grant no. JAT210079) and the Earmarked Fund for Modern Agro-industry Technology Research System of China (CaRS-43).
All authors contributed to the study conception and design. Material preparation and data collection performed by Xuanci Yu, Chenyu Shi and Mengshi Wang, and the study was designed by Fenglai wang. The first draft of the manuscript was written by Caiyun Huang. The financial support was secured by Caiyun Huang and Ang Li. All authors commented on previous versions of the manuscript. All authors read and approved the final manuscript, and the research question, key design features and analysis plan were prepared before the study.
The authors declare that the research was conducted in the absence of any commercial or financial relationships that could be construed as a potential conflict of interest.
All protocols used in the present experiments were approved by Animal Care and Use Committee (ACUC) in Fujian Agriculture and Forestry University (ID: PZCASFAFU22017).