7.1 Introduction
The entire world sat up and took notice when, in 2018, President Donald Trump directed the creation of the United States Space Force.Footnote 1 The move was subject to widespread ridicule, including through a Netflix comedy series starring Steve Carell and John Malkovich. In reality, this new sixth branch of the US armed forces was little more than a renamed US Air Force Space Command (a conclusion supported, among other things, by the fact that the ‘chief of space operations’ reports to the Secretary of the Air Force). While there is no denying that the US military is a significant Space actor – it has about 200 operational spacecraftFootnote 2 and an annual Space-related budget of approximately US$20 billionFootnote 3 – this is not new, since militaries have always accounted for a large portion of human Space activity.
Humanity’s ascent into Space began in 1929 when the German Army tested its first rocket, the A-1.Footnote 4 The size and reliability of the German rockets were gradually increased until, in 1942, the first test launches of the A-4 took place.Footnote 5 Two years later, the A-4 was renamed the V-2 and deployed against Paris, London and Antwerp. Although the V-2 was inaccurate and therefore not militarily decisive, it was still a major technological achievement. Capable of carrying a one-tonne payload 320 kilometres, it travelled on a ballistic trajectory that took it far beyond the reach of anti-aircraft guns.Footnote 6 In 1944, a V-2 reached an apogee of about 180 kilometres, making it the first human-made object to reach Space (using the most widely accepted definition of 100 kilometres, often called the Kármán line). More than 3,000 V-2s were launched during the last year of the Second World War.Footnote 7
As the war drew to a close, both the United States and the Soviet Union raced to capture German rocket engineers. The lead engineer, Wernher von Braun, made sure that he fell into American hands. The United States also captured enough parts to build around 80 rockets. In 1949 a modified V-2 launched by the US Army became the first US-launched object to reach Space.Footnote 8 Further modifications led to the Redstone rocket, which carried the first American satellite into orbit in 1958. That same year, the Redstone was deployed in West Germany as a tactical missile armed with a nuclear warhead.Footnote 9 The Soviet Union, meanwhile, had also captured German rocket engineers. In 1957 it launched Sputnik, the world’s first artificial satellite, on an R-7 rocket that was based on the V-2 design. Two years later, the R-7 went into operation as the Soviet Union’s first intercontinental ballistic missile (ICBM).
While militaries have always accounted for a large portion of human Space activity, their use of the Space environment has been constrained by a mutual self-interest in preserving access to it for a broad range of purposes. As James Clay Moltz explains, the United States and the Soviet Union ‘gradually accepted mutual constraints on deployable weapons in return for safe access to the space environment for military reconnaissance, weather forecasting, tracking, early warning, and a range of civilian uses’.Footnote 10 This chapter traces the development of these constraints while also considering several new issues, including the growing need for a new treaty to ban kinetic anti-satellite (ASAT) weapon tests, and the potentially destabilising effects of an imminent extension of military activities to cis-lunar Space. The following chapter will evaluate existing international law, including international humanitarian law, as it concerns the testing and use of ASAT weapons.
7.2 Preserving Safe Access to Space: The 1963 Limited Test Ban Treaty
The sky above the Pacific Ocean turned red for an hour on 9 July 1962, after the United States detonated a 1.4-megaton hydrogen bomb at an altitude of 400 kilometres to test, in part, whether an artificial intensification of the Van Allen radiation belts – where highly charged particles from the solar wind are captured by Earth’s magnetic field – could disable intercontinental ballistic missiles.Footnote 11 The Starfish Prime nuclear test worked much better than expected, generating a powerful electromagnetic pulse that disabled six satellites – one Soviet, one British, and four American. All three countries drew the same lesson from the test: nuclear explosions in Space posed a major and indiscriminate threat to new Space-based technologies.
Some of the technologies had commercial applications. One of the disabled satellites was Telstar 1, which had just begun transmitting the first live television broadcasts between North America and Europe. Other technologies were of fast-growing military importance, including reconnaissance satellites able to track the activities of adversaries from beyond the reach of fighter–interceptor jets and anti-aircraft guns. These reconnaissance satellites enabled the United States and the Soviet Union to avoid a classic ‘security dilemma’ in which a state is compelled to make a choice between building up its military, or not, in response to another state’s suspected but uncertain build-up.Footnote 12 Radiation from the Starfish Prime nuclear test, moreover, not only dispersed along the Van Allen Belts, but persisted there. The presence and persistence of this additional radiation posed a potential threat to human spaceflight at a time when the United States and the Soviet Union were competing to put the first humans on the Moon.
The lessons of Starfish Prime were taken up almost immediately in the 1963 Treaty Banning Nuclear Weapon Tests in the Atmosphere, in Outer Space and under Water.Footnote 13 This Limited Test Ban Treaty (also known as the Partial Test Ban Treaty) was negotiated by the United States, the Soviet Union, and the United Kingdom – the same three countries that had lost satellites as a result of the nuclear test.Footnote 14 For almost six decades now, the treaty’s provisions have been fully complied with, because they serve the interests of every modern military and national Space agency.
The Limited Test Ban Treaty was the first of a series of arms control treaties based on a recognition that keeping weapons out of Space is a prerequisite for avoiding nuclear conflict on Earth. In 1967, the Outer Space Treaty prohibited the deployment in Space of ‘any objects carrying nuclear weapons or any other kinds of weapons of mass destruction’.Footnote 15 In the 1972 Strategic Arms Limitation Talks Agreement (SALT I),Footnote 16 the United States and the Soviet Union limited the number of silos and launch tubes available for ground- and sea-based ICBMs, i.e. missiles that transit Space en route to their targets.
The same talks led to the 1972 Anti-Ballistic Missile Treaty,Footnote 17 in which the United States and the Soviet Union limited missile defence systems to 200 interceptors each, protecting a maximum of two locations.Footnote 18 This ABM Treaty, which also specifically prohibited Space-based anti-ballistic missile systems, was designed to preserve ‘mutually assured destruction’ by ensuring that most ICBMs would reach their targets regardless of whether they were launched as part of a first or a second strike. It also had the consequence of preserving Space as a region through which weapons would travel, rather than one in which armed conflict would take place. SALT I and the ABM Treaty further prohibited interference with ‘national technical means of verification’.Footnote 19 In other words, states party to the treaty could not interfere with any reconnaissance satellites used to verify treaty compliance. This amounted to a ban on the use of weapons of any type against an entire category of satellites.
7.3 ASAT Weapons and Space Debris
Satellites are indispensable tools for providing global security, but travelling as they do on predictable paths, they are also vulnerable. Their use in surveillance, reconnaissance, communications and high-precision targeting, and in the operation of armed drones and ‘fifth-generation’ fighter jets such as the F-35, all make them attractive military targets. Indeed, destroying just a few such satellites should be relatively easy, and could render an enemy’s armed forces both deaf and blind, particularly if a state’s dependence on satellites is asymmetrical to that of its adversaries.
Several types of ASAT weapons were developed during the early years of the Cold War. The first American test took place in 1959 as part of Bold Orion, a program involving air-launched ballistic missiles.Footnote 20 The Soviet Union for its part conducted 20 Space-based ASAT weapon tests between 1968 and 1982.Footnote 21 Practically speaking, any ground-, sea- or air-based missile can be used to destroy a satellite, if it has sufficient range and an accurate enough guidance system. At the same time, any satellite can, at least in principle, also be used as an ASAT weapon – if it has sufficient propulsion, control and targeting to manoeuvre itself onto a collision course with another object. In this chapter, we focus on these kinds of ‘kinetic’ ASAT weapons that employ violent impacts and therefore create Space debris; in the next chapter, we also discuss ‘non-kinetic’ methods of disabling satellites or interrupting their communications, such as cyber actions and jamming.
As the United States and the Soviet Union continued to develop and test both ‘direct-ascent’ and Space-based ASAT weapons, a major problem emerged: any single ‘kinetic’ impact can create tens of thousands to hundreds of thousands of individual pieces of dangerous Space debris. Only the largest of these, corresponding to hundreds to thousands of pieces for a single impact event, are trackable. Most of the debris is too small for Earth- or Space-based sensors to detect, but these small pieces can still be lethal.
Debris and satellites orbit the Earth at speeds of up to approximately 28,000 kilometres per hour (about 7.8 kilometres per second), with relative speeds of up to twice that. For this reason, even pieces of debris as small as three to five millimetres in diameter can disable operational satellites, including ones belonging to the same country that has tested or used a kinetic ASAT weapon, thus putting its own Space assets at risk.
An even greater problem was recognised in 1978, when Donald Kessler and Burton Cour-Palais, building on ideas from solar system dynamics, identified that every collision, explosion or other debris-generating event in orbit increases the cross-sectional area of the material involved and therefore the risk of further collisions, further fragmentations and so on.Footnote 22 Known today as the ‘Kessler syndrome’, or, more accurately, the ‘Kessler–Cour-Palais syndrome’,Footnote 23 this phenomenon of the runaway proliferation of Space debris has the potential to render entire orbits unsafe for centuries.Footnote 24 The Kessler–Cour-Palais syndrome thus threatens all military and civilian satellites, including those used for weather forecasting, navigation, aircraft and ship communications, financial services, agriculture, forestry, fisheries, environmental science, search and rescue, and disaster relief. Even a limited conflict in Space could be devastating to the global economy, food supply and human security.
Increased debris also poses a risk to human spaceflight. Already, the International Space Station has been boosted out of the way of Space debris on some 30 occasions.Footnote 25 These manoeuvres were in response to larger pieces of debris, since only pieces about ten centimetres in diameter or more can be identified and tracked. Millions of smaller pieces of debris also pose a significant threat, for something as small as a paint fleck or metal chip can penetrate an astronaut’s spacesuit at orbital speeds.
Kessler and Cour-Palais’s article had an almost immediate effect on international co-operation. Just one year after it was published, negotiators from the United States and the Soviet Union came to a preliminary agreement on banning the testing and use of ASAT weapons.Footnote 26 The agreement, however, was never finalised due to the Soviet invasion of Afghanistan in December 1979,Footnote 27 and the subsequent election of Ronald Reagan as US president in November 1980.
7.4 From Reagan to Clinton
The 1980 election of Ronald Reagan was a major setback for international Space co-operation. The new administration was populated with ‘hawks’ who had little respect for multilateralism and international law. In 1983, Reagan announced the Strategic Defense Initiative (SDI). Widely referred to as ‘Star Wars’, this massively expensive programme sought to render ICBMs obsolete through the development of ground-, sea-, air- and Space-based missile defence systems.
Experts on Space security in the United States have long been divided into two camps. The first advocates for the United States to seek to dominate Space, including by displaying an ability to destroy an adversary’s Space assets in response to any aggression. The second camp understands that any armed conflict in Space could render key parts of that region unusable for everyone and recognises that spacefaring states have long been co-operating to prevent this from happening. The first camp benefits from the popular image of Space as an inherently violent region, while the second finds support in the fact that Space remains peaceful after more than six decades of human activity. Indeed, no country has ever deliberately struck another country’s spacecraft.
Although the risk of the Kessler–Cour-Palais syndrome had already been publicly identified, both the Reagan administration and US military leaders were in wilful denial of the Space debris problem. When the US Air Force decided to test an air-launched missile against a satellite in 1985, it did so over the strong objections of NASA scientists. The scientists’ concerns were validated when the test created 285 pieces of trackable debris and many thousands of smaller pieces.Footnote 28 This brief period of wilful denial ended abruptly: further tests were cancelled, and within a year the US Department of Defense was adopting its first debris mitigation guidelines. By 1989, the George H. W. Bush administration was promoting Space debris mitigation with other spacefaring states and international organisations. This included the creation of a new US–Soviet Orbital Debris Working Group.
During the 1991 Gulf War, satellites proved to be of considerable military utility. Earth-imaging satellites enabled US forces to track Iraqi units from Space, while an early global positioning system (GPS) enhanced situational awareness and assisted with precision targeting. These US successes contributed to a global awareness of the value of Space-based assets, and with it of the critical importance of safe access to Earth orbit. In 1993, the Bill Clinton administration led the creation of the Inter-Agency Space Debris Coordination Committee (IADC).Footnote 29 The Russian Space agency (known today as Roscosmos) was a member from the start, and the China National Space Administration (CNSA) joined two years later.
7.5 China’s 2007 ASAT Weapon Test
In 2001, the Reagan-era hawks returned to Washington as part of the George W. Bush administration. They promptly initiated a programme of US Missile Defense: a scaled-back version of SDI focused on ground- and sea-based interceptors. They also withdrew the United States from the ABM Treaty, recognising that their new program would have violated it. These moves created strategic uncertainties for other countries, uncertainties that may have contributed to China’s decision to test a ground-based missile against a defunct satellite in 2007.Footnote 30
Just as the United States’ 1985 test was conducted by its Air Force over the objections of NASA scientists, China’s 2007 test may have been conducted by the Peoples’ Liberation Army without the fully informed support of the Chinese Ministry of Foreign Affairs and other civilian government departments.Footnote 31 The test involved a ground-based missile and a defunct Chinese satellite. It resulted in 3,527 pieces of debris large enough to be logged in the US military’s satellite catalogue and about 150,000 inferred pieces greater than one centimetre. Making things worse, the strike took place at an altitude around 850 kilometres, which meant that some of the debris will remain in orbit for centuries. At the time, the US Air Force Space Command estimated that over 700 satellites, including the International Space Station (ISS), were at risk of being struck by debris from the Chinese test.Footnote 32 Sure enough, in 2013, one of the pieces collided with and disabled a Russian satellite.Footnote 33
7.6 The United States’ 2008 Satellite Intercept
Just one year after China’s ASAT weapon test, the US Navy used a sea-based SM-3 missile defence interceptor to destroy a malfunctioning reconnaissance satellite, USA 193.Footnote 34 The interceptor struck the satellite at an altitude of about 240 kilometres, with the aim of producing no long-lasting debris. That aim, however, was not achieved, with 174 pieces of trackable debris subsequently listed in the US military’s satellite catalogue. While about 90 per cent of that debris de-orbited within two months, it took 20 months before the last pieces did so.
The United States claimed that the intercept had been necessary for public safety reasons, since the satellite was about to re-enter Earth’s atmosphere loaded with highly toxic hydrazine thruster fuel, some of which might have reached the surface. It is possible that China’s ASAT weapon test of the previous year was a factor in the United States’ decision, even though this has been strongly denied.Footnote 35 What is certain, however, is that, since 1985, the US military has been consistent on the issue of Space debris. As General John E. Hyten, the commander of the Air Force Space Command, said in 2015, ‘Kinetic [anti-satellite weaponry] is horrible for the world … the one limiting factor is no debris. Whatever you do, don’t create debris.’Footnote 36
7.7 India’s 2019 ASAT Weapon Test
In 2019, India tested a ground-based missile defence interceptor against a satellite (Microsat-R) that it had launched for that purpose.Footnote 37 It designed the impact to occur about 283 kilometres above the Earth and assured other countries that no long-lasting debris would result.Footnote 38 The actual outcome was quite different.
Figure 7.1 shows the result of the impact, with debris extending above low Earth orbit (LEO) at apogee. It should be stressed that this is just the debris that can be tracked. There are roughly 130 such pieces in the US military’s satellite catalogue, meaning that they were in orbit long enough to be tracked and assigned an identifier. It is reasonable to assume that there was at least one order of magnitude more pieces between one and ten centimetres in size, all of them still potentially lethal to satellites, Space stations and astronauts.

Figure 7.1 Gabbard plot showing the apogee–perigee distribution of tracked fragments resulting from India’s ASAT weapon test (destruction of Microsat-R). The approximate altitude of the ISS is shown with a star. The apogee and perigee of Microsat-R just prior to the impact are shown by the downward and upward triangle. Despite the impact occurring at about 280 kilometres, tracked debris extended above LEO (i.e. above 2,000 kilometres in altitude). Note that if an object (such as an operational satellite) is between the red and blue points on this plot, then the debris crosses that object’s orbital altitude.
Figure 7.2 demonstrates that much of the debris from India’s ASAT weapon test remained in orbit for months, taking over a year for 90 per cent of the tracked debris to de-orbit. This can be compared with the USA 193 event in 2008, which took place at a lower altitude, and saw 90 per cent of the material de-orbiting in about two months. Neither situation was ideal, and both led to debris that went to high apogees. Regardless, the USA 193 event should have been a clear indication that some long-lived debris would be produced by a weapon test at the altitude of Microsat-R.

Figure 7.2 Fraction of USA 193 and Microsat-R debris de-orbited by the number of months after the events. The USA 193 strike took place at an altitude of about 240 kilometres, leading to a relatively rapid loss of the debris, with about 90 per cent of the trackable debris pieces having de-orbited after about two months. In contrast, the Microsat-R strike occurred at about 280 kilometres, leading to much longer-lived debris, with 90 per cent of the debris de-orbiting only after 13 months.
Initially, the international response to India’s ASAT weapon test was muted, presumably because of the assurances that no long-lasting debris would result. This changed when it became apparent that considerable debris had remained in orbit. Then NASA Administrator Jim Bridenstine was especially critical because (as Figure 7.1 shows) many of the pieces crossed the International Space Station’s altitude. Describing this as ‘a terrible, terrible thing,’ Bridenstine said, ‘it is not acceptable for us to allow people to create orbital debris fields that put at risk our people’.Footnote 39
India’s ASAT weapon test was likely intended to demonstrate to China, its larger and more powerful regional rival, that any attacks on Indian satellites could be responded to in kind.Footnote 40 Regardless of India’s motives, its actions also had the result of heightening international concerns about kinetic ASAT weapon tests, concerns that are only destined to grow as LEO becomes busier.
7.8 Russia’s 2021 ASAT Weapon Test
On 15 November 2021, Russia’s military used a ground-based missile to strike Kosmos 1408, a defunct Soviet-era satellite with a mass of about 1,750 kilograms orbiting at an altitude of about 480 kilometres. Again, due to the high impact energies involved, debris from a kinetic ASAT weapon test such as this inevitably ends up on highly eccentric orbits that cross the orbital altitudes of thousands of other satellites twice per revolution. Over time, as the debris from the test de-orbits, it will all pass through the altitudes of the International Space Station and China’s new Tiangong Space station, placing astronauts, cosmonauts and taikonauts at deadly risk.
Figure 7.3 highlights how debris from this test crosses the orbits of the two Space stations, satellite mega-constellations currently being deployed, and many operational Russian satellites. This last point is especially important: the action was contrary to Russia’s own investments and interests in Space.

Figure 7.3 Orbital trajectories for 340 pieces of debris from the Russian ASAT weapon test. The debris fragments cover a large range of low Earth orbits, crossing critical infrastructure, including the ISS, the Tiangong Space station and Russian satellites. Moreover, due to the high altitude of the test, all of the debris fragments will pass through the orbital space of the ISS and the Tiangong Space station as they decay.
The figure has been produced using Kosmos 1408 debris from two-line elements (TLEs) available through the United States Space Command (USSPACECOM) as of 7 December 2021. The grey curves show the path of each debris piece based on its first available TLE. Only about 340 of the estimated 1,500 tracked debris pieces are included in the figure, for clarity (and were the only ones available as of 7 December 2021).
We estimate that at least 50 operational Russian satellites are at altitudes traversed by the debris from the November 2021 test. Twenty-five of these are operated by the Russian Ministry of Defence, five by Roscosmos and five by Lomonosov Moscow State University. These are not small satellites: 21 of them have masses greater than a tonne. Moreover, at least 30 of these satellites are at altitudes that experienced a significant increase in the debris field due to the test. For reference, we have included the approximate orbital altitudes of select satellites and satellite constellations on the above plot, as well as the two Space stations.
Not included on the plot is the lethal, non-trackable debris, which is of particular concern because it will be more abundant than the trackable debris by at least an order of magnitude. Since small debris cannot be detected, collision avoidance manoeuvers cannot be used to protect against them. And again, at typical relative speeds of about 10 kilometres per second (36,000 kilometres per hour), even a tiny piece can disable a satellite or kill an astronaut, cosmonaut or taikonaut.
To demonstrate the degree to which the 15 November 2021 test endangered Russian and all other orbital assets, we compared the debris field before the test with the debris field shortly after. In Figure 7.4, the blue line shows the percentage increase in the debris density across all altitudes, taken at face value using the catalogued debris as of 27 January 2022. The background grey line shows the satellite density distribution as of 17 November 2021, with Starlink clearly visible.
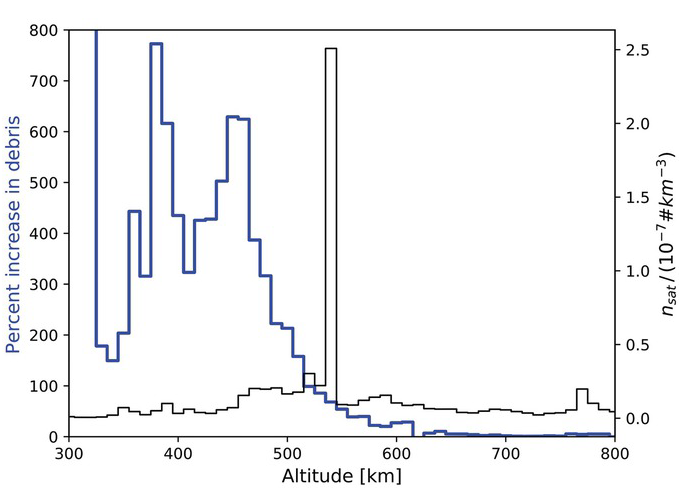
Figure 7.4 The blue, thick line shows the increase in tracked debris across different altitudes due to the Russian ASAT weapon test. The increase is determined by comparing the USSPACECOM satellite catalogues as of 17 November 2021 (just after the event but before event debris was catalogued) and as of 27 January 2022. The grey, thin line shows the satellite number density as a function of altitude, with Starlink clearly visible. Ten-kilometre bins are used for determining the debris and satellite densities, weighted by the time the object spends within a given altitude bin.
It bears repeating that every collision increases the cross-sectional area of the material involved and therefore the risk of further collisions. The most extreme outcome is, again, the Kessler–Cour-Palais syndrome. But there are other serious, less dramatic concerns. For example, even at low-altitude orbits, sudden injections of material will proliferate small debris through knock-on collisions that could significantly interfere with satellite operations and human spaceflight.
Some of the debris from the November 2021 test will de-orbit quickly. Indeed, debris curves in the first plot that appear to be ‘detached’ from their birth altitude have already experienced substantial orbital evolution due to atmospheric drag. However, a significant fraction will remain in orbit for years or even decades, with the precise ‘clearing time’ dependent on the characteristics of the fragments and the behaviour of the atmosphere.
Such debris does not produce a consistent level of threat either. Earth is not a perfect sphere, which results in the orientation of orbits evolving with time, while keeping the same overall inclination. Astrophysicists would say that the orbit ‘precesses’. To visualise this, imagine the edge of a coin as representing an orbit, and a spinning and wobbling coin representing the orbit’s precession. Now, when the orientation of the debris orbit becomes nearly anti-aligned with another satellite’s orbit (i.e. the debris and the satellite are moving towards each other), there can be episodic periods of very high ‘conjunction’ activity (close approaches between objects) involving clusters of debris.
Several such ‘conjunction squalls’ arising from the Russian ASAT weapon test have been predicted by COMSPOC,Footnote 41 one of several new companies offering ‘Space situational awareness’ (SSA) – essentially, knowledge about the Space environment and human activities in Space. Even if these squalls only create a modest increase in the collision risk, the sheer number of conjunctions could overload SSA efforts or trigger excessive collision avoidance manoeuvres by other actors. This strains Space safety and could lead to secondary failures or accidents.
As we have explained above, the Russian military is not the only military to have tested a kinetic ASAT weapon in a manner that was certain to create long-lasting debris. But the action was more imprudent in 2021 than it was in 1985, 2007 or even 2019. This is because the fast-changing orbital environment has made such activities significantly more dangerous than before. There are currently over 7,000 satellites (active and defunct) in LEO, as compared to just over 3,000 three years ago. And this number is projected to grow quickly – to over 100,000 satellites by 2030 – largely because of the construction and completion of numerous satellite mega-constellations. It is thus clear that any additional kinetic ASAT weapon tests, by anyone, will threaten the interests of all spacefaring states – including Russia, India and China. It is therefore imperative that the international community move forward on this issue, with all deliberate speed.
7.9 Diplomatic Initiatives
In 1979, the United States and the Soviet Union negotiated the preliminary text of a treaty that would have banned the testing and use of ASAT weapons (as we noted above).Footnote 42 More recently, in 2008 and again in 2014, China and Russia jointly introduced a draft Treaty on the Prevention of the Placement of Weapons in Outer Space, the Threat or Use of Force against Outer Space Objects (PPWT) at the Conference on Disarmament.Footnote 43 The draft treaty is quite simple, centring on a commitment to not ‘place any weapons in outer space’, with a ‘weapon in outer space’ being defined in the 2014 draft document as
any outer space object or its component produced or converted to eliminate, damage or disrupt normal functioning of objects in outer space, on the Earth’s surface or in the air, as well as to eliminate population, components of biosphere important to human existence, or to inflict damage to them by using any principles of physics.Footnote 44
The China–Russia draft treaty also reaffirms within the Space context the UN Charter’s Article 2(4) prohibition on the threat or use of force, with a prohibition on ‘the threat or use of force against outer space objects of States Parties’.Footnote 45 As with the UN Charter, the draft treaty makes clear that this prohibition ‘shall by no means affect the States Parties’ inherent right to individual or collective self-defence, as recognized by Article 51 of the UN Charter’.Footnote 46 The right of self-defence is part of customary international law, and its implications for ASAT weapons are explored in the next chapter.
The United States, along with some non-governmental experts, criticised the China–Russia draft treaty for failing to provide verification measures and for not addressing direct-ascent ASAT weapons.Footnote 47 Such criticisms were relied on by the United States to justify blocking negotiations on the draft treaty in the Conference on Disarmament, which operates on a consensus basis.
In our assessment, the criticisms of the China–Russia draft treaty were misplaced, for two reasons. First, the ability to detect the use and even the deployment of kinetic ASAT weapons is constantly improving because of technological improvements in Space situational awareness. These improvements are being driven, in part, by the fast-growing need to identify and track satellites and Space debris to prevent accidental collisions. Numerous ground-based sensors and some Space-based sensors are now dedicated to this purpose.Footnote 48 There is no need for a treaty provision requiring countries to acquire verification tools that they already possess and are quickly improving.
Second, direct-ascent ASAT weapons are in fact dealt with in the China–Russia draft treaty through the prohibition on the ‘threat or use of force against outer space objects of States Parties’. Although this is a prohibition of the use of such weapons rather than of their deployment, the prohibition needs to be focused in this way because missile defence interceptors can be used as ASAT weapons, as China, India and Russia have already demonstrated through tests and, in the case of the United States, through the destruction of a defunct re-entering satellite loaded with toxic thruster fuel. In other words, one could not prohibit the existence of direct-ascent ASAT weapons without also banning surface-based missile defence systems such as the SM-3 missiles on US Aegis class naval vessels, which is something the United States would never accept.
For these reasons, it seems likely that US opposition to the China–Russia draft treaty was politically motivated, and not by concerns about the scope and likely effectiveness of the instrument. Of course, there would have been political reasons behind China and Russia proposing the treaty in the first place, including concerns about the United States possibly developing a Space-based missile defence system after its denunciation of the 1972 Anti-Ballistic Missile Treaty in 2002. Political reasons may also explain why China and Russia have not taken their draft treaty to another forum, one where decisions are not based on consensus and the United States is unable to block negotiations. That said, it is possible that China and Russia regard the United States as a necessary partner in any treaty on Space weapons, in which case moving to another forum would offer them no advantage.
The China–Russia draft treaty is consistent with a resolution that has been adopted, annually and with near unanimity, by the United Nations General Assembly on the Prevention of an Arms Race in Outer Space (PAROS).Footnote 49 It is also consistent with a resolution on No First Placement of Weapons in Outer Space, which the General Assembly has adopted on five occasions since 2015.Footnote 50
Several individual countries have also made specific proposals that align with the China–Russia draft treaty. After India’s 2019 ASAT weapon test, the German delegation to the Legal Subcommittee of the UN Committee on the Peaceful Uses of Outer Space (COPUOS) called for a ‘legally binding prohibition of the intentional destruction of space objects resulting in the generation of long-lasting debris, including in situations of armed conflict’.Footnote 51
Other proposals have focused on banning the testing of kinetic ASAT weapons rather than their deployment or use. In January 2020, Canada’s representative at the Conference on Disarmament spoke about the desirability of negotiations on specific arms control issues in Space, ‘such as negotiating a potential end to ASAT testing causing space debris’.Footnote 52
The Russian diplomat Alexey Arbatov has proposed that Russia and the United States take the first step with a bilateral agreement against the ‘testing of anti-satellite systems involving the actual destruction of target satellites (space objects)’.Footnote 53 Such a treaty is possible, Arbatov says, because the two countries have a shared interest in limiting the development of weapons that threaten satellites designed to provide early warning of nuclear strikes.Footnote 54 He calls for the inclusion of clear transparency and co-operation requirements and argues that verification could be provided by existing missile detection systems, modified, if necessary, so that they can also monitor for kinetic ASAT weapon tests.Footnote 55
Ross Liemer and Christopher Chyba have proposed that kinetic ASAT weapon tests be prohibited above a set altitude, to prevent the creation of Space debris without banning testing completely.Footnote 56 They suggested that the altitude cap could be set by the Inter-Agency Space Debris Coordination Committee (IADC), and that Space powers could negotiate this partial test ban in a venue such as the Conference on Disarmament. They argued that an altitude cap, rather than an outright ban on testing, is more likely to garner broad international support because it would not discriminate against those countries that currently lack kinetic ASAT weapons, since they could develop and test their new systems below the set altitude. Liemer and Chyba also argued that an altitude cap would enable countries to maintain existing ASAT weapon and missile defence capabilities, and to destroy their own satellites in the event of a hazardous uncontrolled re-entry by doing so at a low altitude where Space debris would not be created. Finally, they asserted that a widely supported total test ban (i.e. one that covered all altitudes) would be difficult to achieve if it forbade ‘debris-producing ASAT tests [by all countries] but permitted midcourse-interception ballistic missile defence tests, which China and the USA may view as essential to their national security’.Footnote 57
However, even low-altitude tests will result in Space debris, because the large amount of energy imparted in the impacts can kick small pieces of debris into highly elliptical orbits, as the Indian ASAT weapon test and even the USA 193 event demonstrated. Most of this debris might not stay in Space very long, because of the effects of gas drag at perigee,Footnote 58 but the debris could stay on mega-constellation- and Space station-crossing orbits for weeks to months. Going to lower and lower orbits will reduce the de-orbit timescale, but it does not eliminate initial high-altitude debris. And while debris with a high area-to-mass ratio might de-orbit in days or weeks, pieces with a lower area-to-mass ratio will remain in Space for longer. This sort of variation is to be expected, since the debris resulting from any single ASAT weapon test will have a wide range of characteristics.
The idea of an altitude cap for ASAT weapon tests thus depends on states finding common ground on an acceptable risk threshold. Explosions at an altitude of, for example, 150 kilometres will ensure very rapid de-orbits, but not without any risk due to the lofting into orbit of uncontrolled material, however temporary. For all these reasons, anything less than an absolute ban on kinetic ASAT weapon testing will leave some risk, including for astronauts, cosmonauts and taikonauts.
Geoffrey Forden has suggested several ways in which a ban on kinetic ASAT weapon tests could be achieved.Footnote 59 One way would be for states to ‘pledge to avoid creating persistent space debris by following the guidelines of the Inter-Agency Space Debris Coordination Committee’. This approach has the attraction of not requiring a renegotiation of the required behaviour. There are two ways in which similar pledges have created legal obligations in the past. First, ‘unilateral declarations’ can be binding on countries, as the International Court of Justice explained in the Nuclear Test Cases: ‘When it is the intention of the State making the declaration that it should become bound according to its terms, that intention confers on the declaration the character of a legal undertaking, the State being thenceforth legally required to follow a course of conduct consistent with the declaration.’Footnote 60 The threat of Space debris is so very great that one could imagine a spacefaring state unilaterally declaring its intention to treat the IADC guidelines as legally binding.Footnote 61 And while these kinds of declarations are unusual, Forden has to be credited with foresight and perhaps even influence because, in April 2022, the United States announced that it would unilaterally impose an ASAT weapon test ban on itself.Footnote 62 We will discuss this development in the next chapter.
A more usual law-making path would involve a number of spacefaring states agreeing to make the IADC guidelines legally binding, among themselves, through a new treaty. Guidelines and other forms of ‘soft law’ are often transformed into hard law through the treaty-making process, with the subsequent adoption of all the provisions of the UN General Assembly’s Declaration of Legal Principles Governing the Activities of States in the Exploration and Use of Outer Space into articles of the Outer Space Treaty being a case in point.
Forden’s second suggestion was ‘to ban one spacecraft from approaching another orbiting spacecraft at excessive speeds’.Footnote 63 He suggested a threshold of 100 metres per second for spacecraft located within 100 kilometres of each other. As Forden explained,
These speeds and distances are great enough not to interfere with much of the normal operating procedures in space and yet would still obstruct the development of the tracking, guidance, and control of any ASAT weapon. At the same time, they do not prevent the testing and deployment of ground-based missile defenses because the target is not in orbit.
It is important to recognise that Forden made this suggestion in 2007, when LEO was not as crowded as it is today. ‘Conjunctions’ – when two satellites come within a few kilometres of each other at high relative speeds – now occur every few minutes,Footnote 64 a situation that will only be exacerbated by mega-constellations.
Proposals also exist for a prohibition on all ASAT weapon testing, including the testing of non-kinetic ASAT weapons, to prevent a broader arms race in Space.Footnote 65 Unfortunately, such proposals immediately encounter problems with respect to verification, namely the detection of cyber and other non-kinetic tests, as well as the potential dual-use character of some Space systems, such as those proposed for the active removal of Space debris. That said, there are many multilateral treaties that have been adopted despite verification problems, with the Convention against Torture being a case in point.Footnote 66 We know that the existence of such a treaty can shape state practice, both by ‘marshalling shame’ and by forcing violators to conceal and deny their actions.Footnote 67
In any event, these proposals for a prohibition on all ASAT weapon testing provide additional evidence of growing concerns about ASAT weapons and their negative consequences, especially Space debris and the Kessler–Cour-Palais syndrome. Together with all the other evidence considered above, they suggest that an intermediate step – a treaty banning kinetic ASAT weapon tests – could receive widespread support.
Now it would be easy to assume that the November 2021 Russian ASAT weapon test will set back efforts to negotiate a ban on kinetic testing. But there are several reasons to hope that this will not be the case. First, it is possible that Russia wished to test (and, perhaps more importantly, demonstrate) the effectiveness of its new PL-19 Nudol missile system as an ASAT weapon before negotiating a test ban with other spacefaring states.Footnote 68
Second, it is possible, perhaps even likely, that the Russian military conducted the test without the support or even the knowledge of the Russian Space agency (Roscosmos) and the Russian Foreign Ministry.Footnote 69 If so, the influence of the latter two branches of the Russian government may have been strengthened by the fact that the test created over a thousand pieces of trackable and many thousands of pieces of untrackable debris in orbits that create non-trivial risks for valuable Russian satellites and cosmonauts on the International Space Station. Although it is difficult to assess the internal politics of the Vladimir Putin regime, we know that Roscosmos is concerned about Space debris and that the Russian Foreign Ministry has participated constructively in COPUOS and UN General Assembly negotiations on this issue.Footnote 70
Third, Russia has strenuously denied that their November 2021 test created risks for operational satellites or the Space stations. Such a position is not supported by the measured distribution of Kosmos 1408 debris, as we discussed above. Yet the denial itself constitutes an acknowledgement, by the Russian government, that the deliberate creation of dangerous debris is unacceptable today. For international lawyers, such denials are significant as evidence of opinio juris, the subjective element of customary international law. They can also count as ‘state practice’ that, in terms of its law-creating effect, is just as significant as the action being denied.
Again, the prohibition on torture, which is widely accepted as having achieved the heightened, peremptory status of a jus cogens rule of customary international law, provides a powerful example of how such rules can develop. As Anthony D’Amato wrote,
It seems … important to ask whether the states that engage in torture are (a) disclosing that they are torturing people, (b) proclaiming that what they are doing is legally justified, and (c) implicitly inviting other states to do likewise on the ground that, if torture is legally permissible for them, it is legally permissible for all states.Footnote 71
D’Amato went on to explain that ‘hiding, cover-up, minimization, and non-justification … betoken a violation of law’ and therefore constitute legally relevant state practice in support of a rule prohibiting the actions in question.Footnote 72 Denials are, in short, the tribute that vice pays to virtue – and they can have law-creating effects.
Russia, by denying that it created dangerous debris in November 2021, was strengthening, not weakening, a possible new customary rule against testing ASAT weapons in ways that create long-lasting debris. In the next chapter of this book, we will consider the rest of the state practice and evidence of opinio juris that could be contributing to such a new rule today, including the United States’ announcement, in April 2022, that it would unilaterally impose an ASAT weapon test ban on itself.Footnote 73
Before doing so, however, we turn to a related and often overlooked issue, namely the possibility that missile defence tests may also be contributing to the crisis of Space debris.
7.10 Missile Defence and Space Debris
Increasingly, the same type of ground-based missiles that can be used as ASAT weapons are being developed, tested, and deployed for ballistic missile defence. The SC-19 missile used by China to strike a satellite in 2007 was designed primarily for missile defence, as was the SM-3 missile used by the United States in 2008, the PDV Mk-II missile used by India in 2019, and the PL-19 Nudol missile used by Russia in 2021.
Of all these ballistic missile defence programmes, that of the United States is the most advanced of any country. The US Air Force currently has 44 Ground-Based Midcourse Defense interceptors in Alaska and California that are designed to impact incoming ICBMs as they transit LEO. The US Navy has a much larger number of SM-3 missiles deployed on 41 Aegis class cruisers and destroyers. SM-3 missiles are also deployed at two ‘Aegis Ashore’ sites in Romania and Poland. While SM-3s are designed for intercepting intermediate-range ballistic missiles, they have been successfully tested for use against ICBMs.
We have already discussed the Space debris hazard posed by ground-based missiles when tested or used as ASAT weapons. However, the testing or use of the same type of missiles against incoming ballistic missiles can also create Space debris, at least under certain conditions, if the strike occurs above the atmosphere during the mid-course phase of a ballistic missile’s flight. This issue of ballistic missile defence and Space debris has not previously been publicly addressed, though it may well have been analysed and discussed within government circles.
It would be easy to make two assumptions about ballistic missile defence and Space debris:
(1) The trajectories of ballistic missiles are notably different from low Earth orbits (Figures 7.5 and 7.6), and it would thus seem reasonable, at first glance, to assume that any fragmentation of the missile will only result in pieces that will enter the atmosphere essentially on the same trajectory as before the fragmentation.
(2) A ballistic missile defence interception can take place at relatively low altitudes (e.g. 225 kilometres), where fragments will be subject to strong gas drag forces. It thus seems reasonable, at first glance, to assume that no long-lived Space debris generation will occur.
Both these assumptions require scrutiny.

Figure 7.5 Ballistic trajectories for three different profiles, showing a depressed, an efficient and a high-altitude trajectory. The blue curve represents the surface of the Earth, while the dot-dashed curve is at an altitude of 100 kilometres and the dotted line is at 300 kilometres.

Figure 7.6 Simplified example of the flight times corresponding to the depressed, efficient and high-altitude trajectories in Figure 7.5. The times are based on Keplerian arcs, so they do not account for the time needed to accelerate the ICBMs to burnout (at around 100 kilometres). Nonetheless, this introduces a timing difference of only one to a few minutes, so the overall scale of the flight times is preserved.
Multiple factors will affect whether ballistic missile defence tests produce orbital debris and the degree of danger such debris will cause: the shape of the ballistic missile trajectory, the point of impact between interceptor and ballistic missile, and their relative velocities, which will then affect the nature and number of fragments produced, as well as the change in velocity (Δv) of each fragment, which in turn will affect trajectories for debris that achieve orbital velocities. The analysis of these factors and the danger they pose to the Space environment is complex but telling.
The mid-course phase of an ICBM’s flight begins when it is no longer under power and is following a ballistic trajectory. This can begin at a range of altitudes, depending on the flight profile. However, the maximum altitude reached along its arc is typically between 300 and 1,300 kilometres. Recall that the International Space Station orbits at an altitude of around 400 kilometres, while the majority of commercial and military satellites orbit at altitudes between 300 and 800 kilometres. Even a ‘depressed’ trajectory, which requires more energy but might reduce the exposure to missile defence interceptors, will see the missile spend most of its flight above 200 kilometres, where – as we have seen above and will revisit below – an impact can still create Space debris that imperils satellites higher up.
US ballistic missile defence tests have been conducted at several different altitudes and speeds. Variation in test conditions is important to ensure effectiveness across a range of scenarios, such as the time of day, altitude and closing speeds (the relative speed of the two objects as they approach each other). Quite a few tests have taken place at an altitude of 225 kilometres, although the highest and fastest test interception to date has been FTG-15, which took place in 2017 at an altitude of 740 kilometres (after the target missile had begun its descent from a peak altitude of 1,250 kilometres). A schematic of the FTG-15 interception is shown in Figure 7.7.

Figure 7.7 Depiction of the FTG-15 interception test. Credit: Laura Grego and David Wright, ‘Incremental Progress but No Realistic Capability: Analysis of the Ground-Based Midcourse Missile Defense Test FTG-15’ (2018) Union of Concerned Scientists Report, online: www.ucsusa.org/resources/analysis-gmd-missile-defense-test-ftg-15.
In the case of FTG-15, the closing speed of the target missile and the interceptor was approximately 10.2 kilometres per second.Footnote 74
Despite the missiles being suborbital, we know from the discussion above concerning ASAT weapon tests that debris can be given very high-velocity ‘kicks’ during the explosion that results from the collision. Should these kicks be in the ‘right’ direction, orbital speeds can in principle be achieved. To explore the conditions in which debris might be placed into orbit, we make the following ‘toy model’ – a term used in physics for a deliberately simplistic model with many details removed, to enable a concise explanation.
We assume an FTG-15-like high-altitude trajectory with an apogee of 1,250 kilometres and a collision with the kill vehicle at 740 kilometres. The trajectory follows a Keplerian orbital arc for simplicity, and is given a speed of approximately 5.2 kilometres per second. The total break-up mass is assumed to be one tonne, and we further assume that the collision is sufficiently energetic to be catastrophic, i.e. the target and the ‘kill vehicle’ are both completely destroyed. We use the NASA ‘standard break-up model’ for collisional catastrophic fragmentation to determine the number of fragments, the distribution of area-to-mass ratios, and the change in velocity (Δv) for each simulated debris particle (which depends on the area-to-mass ratios in the break-up model).Footnote 75 The Δv is applied to the velocity of the debris at the moment of the collision (the ‘instantaneous velocity vector’), with each debris piece being given a random direction (assuming ‘isotropy’ – i.e. no variation based on direction). The new total velocities can then be used to determine new ‘instantaneous orbits’, i.e. the orbits resulting immediately after the explosion. If the perigees of the fragments – the lowest part of their orbits – are at a high enough altitude, they are potentially long-lived debris.
Figure 7.8 demonstrates that the FTG-15 interception in 2017 likely produced long-lived debris. Most of the material does re-enter the atmosphere, as would typically be expected, including the trackable debris. However – and this is the critical point – some centimetre-size debris is given sufficient Δv to place these small objects into orbit. And while the long-lived debris may only be a small fraction of the total fragmentation debris from the impact event, which consists of tens of thousands of pieces, the debris is still potentially lethal to satellites, Space stations and astronauts.


Figure 7.8 Surviving debris resulting from the catastrophic break-up of a missile during an FTG-15-like ICBM interception test. Fragments are determined according to the NASA standard break-up model. The model only considers fragments between the sizes of one centimetre and one metre. Almost 47,000 debris pieces are produced in the model, 19 of which survive in LEO and about 100 on altitudes below 22,000 kilometres (GPS orbits at approximately 20,000 kilometres). The vast majority of the fragments re-enter the atmosphere, but, clearly, long-lived Space debris is produced by such tests (taken to be fragments with perigees higher than 250 kilometres)
Now let us consider a different profile altogether, namely a depressed trajectory. This toy model has an apogee altitude of 350 kilometres and an interception altitude of 225 kilometres (similar range). If we likewise assume a one-tonne target and a catastrophic collision, long-lived debris is again generated among the centimetre-size population. We have not reproduced plots for this second toy model here, because they look similar to those in Figure 7.8.
It thus seems plausible that ballistic missile defence tests will result in long-lived debris, despite the missiles themselves being suborbital. The fraction of surviving material is much less than that seen in ASAT weapon tests, due to the underlying dynamics. The debris is also limited to smaller pieces, although these are still potentially lethal. The duration that this Space debris will survive in orbit depends on the resulting area-to-mass ratios. However, since the surviving debris has very high apogee, it could spend considerable time in orbit.
So why has Space debris not previously been discussed in the context of ballistic missile defence testing? It may be that the absence of any long-lived trackable debris leads to the impression that such tests will never create any long-lived debris. However, if the NASA standard break-up model applies, our analysis shows that pieces of smaller, untrackable debris can indeed survive – and therefore pose a lethal risk. Admittedly, the severity of this risk is reduced as a result of the long-lived debris being relegated to the so-called ‘tails’ of the distributions, making these events relatively minor contributors to Space debris. But they are purposeful additions of Space debris, and they exacerbate an already dangerous situation in orbit.
Ultimately, the detailed trajectories of the missile and the interceptor, and the specifics of the break-up, will control the amount of material that enters orbit. There is no single threshold for this. But one thing is clear: If we wish to avoid the creation of long-lived Space debris, we need to conduct ballistic missile defence tests at low altitudes only and manage the energy of the impacts.
7.11 Are Debris Concerns Outweighed by the Purpose of Missile Defence Systems?
A second reason why Space debris is rarely discussed in the context of ballistic missile defence may concern the purpose of these systems, which is to prevent nuclear warheads from reaching the Earth’s surface and causing mass destruction there. In other words, it may be assumed that any concerns about Space debris are outweighed by the number of lives that would be lost if an incoming missile were not intercepted.
This assumption can be questioned from the outset with a historical fact. The only US ballistic missile defence system built during the Cold War protected ICBM silos in North Dakota. Its purpose was not directly to save lives, but to ensure that the United States maintained a ‘second strike’ capability in the event of a Soviet first strike. Today, US ballistic missile defence interceptors in Alaska, California and Eastern Europe, and on naval vessels, are considered by many to be a destabilising factor. This is because they might cause potential adversaries to develop new technologies, such as hypersonic cruise missiles that can evade attempted interceptions, or to deploy more missiles to ensure that any missile defence system would be overwhelmed by the sheer number of incoming targets.
Still, one can envisage scenarios where intercepting an incoming ballistic missile would save many lives, for instance if North Korea launched a missile towards a city in the United States. It may be instructive to consider whether the purpose of the interception would feed into a legal analysis. Consider the right of self-defence: an incoming nuclear warhead would meet the threshold of an ‘armed attack’, and the scale of destruction prevented by a defensive interception would very likely fulfil the criteria of necessity and proportionality – even if some long-lasting Space debris were likely to result. For as the analysis above shows, a ballistic missile interception will produce significantly less Space debris than a strike against a satellite. The same conclusion would result from an analysis of the jus in bello, which is also known as either the ‘law of armed conflict’ or ‘international humanitarian law’ and concerns the conduct of armed conflicts that are already under way. A jus in bello analysis involves the principles of military necessity, distinction and proportionality. We will come back to the right of self-defence and the jus in bello in much greater depth in the next chapter, within the context of ASAT weapons.
But use is one thing, and testing is another. The two toy models above suggest that kinetic mid-course missile defence tests will create a small amount of untrackable long-lasting Space debris and therefore increase the risks to satellites, Space stations and astronauts. Such tests should therefore be avoided. Indeed, there is a strong argument for including the topic of ballistic missile defence testing in any negotiations on a treaty banning kinetic ASAT weapon testing. That ban should probably include mid-course missile defence tests above certain relative-speed and combined-mass thresholds, all with the goal of preventing long-lasting Space debris.
7.12 Militarisation of Cis-lunar Space
Cis-lunar Space is about to be developed due to an emerging Space race between China and the United States. Although the race is currently between civilian Space agencies, all the conditions exist for yet another security dilemma.Footnote 76
Cis-lunar (Latin for ‘this side of the moon’) Space is the volume that extends from Earth’s geosynchronous orbits and encompasses the Moon, the Moon’s orbits, the Earth–Moon Lagrange points, and certain types of transfer orbits .Footnote 77 The Lagrange points, or ‘L points’, are defined as locations where the combined gravitational acceleration due to the Earth and the Moon allows a small object, such as a spacecraft, to orbit the Earth at the same rate as the Moon. Due to these unique features, L points will be important for future lunar activities as locations where communications and monitoring equipment, refuelling depots and even Space stations can be maintained at relatively low energy output. Even though cis-lunar Space is very large, extending more than ten times further than geosynchronous orbit, the optimal regions near the five Lagrange points are limited in size. Consequently, they are highly desirable and potentially contested locations (see Figure 7.9).

Figure 7.9 A depiction of the Lagrange points for a simple dynamical model involving two massive bodies (M1 > M2), such as the Earth and the Moon. The curves and colours represent constraints on the motion of a third essentially (i.e. by comparison) ‘massless’ body, such as a spacecraft. The image itself is in the ‘rotating frame’; that is, M1 and M2 appear to be stationary even though they are orbiting each other about their centre of mass. X marks the spot for the L1, L2 and L3 Lagrange points. L4 and L5 are shown as the darker ‘islands’ on the plot. An object at exactly those points will appear to be stationary in this rotating frame. In practice, the objects are placed on orbits that oscillate about the L points. This example sets M2 to be one-third the mass of M1 to accentuate the structure (the actual Moon-to-Earth mass ratio is about one to 81).
Due to the distances involved, Earth-based telescopes and radar are not adequate for monitoring spacecraft in cis-lunar Space. Nor can they monitor the far side of the Moon. Sensors to provide Space situational awareness will be required in cis-lunar Space itself.
The Lagrange point known as L2 offers an ideal location to place a spacecraft for surveillance or as a communications relay. Satellites are not placed directly at L2 because it is an unstable equilibrium point, like a pencil placed on its tip. But they can maintain a ‘halo’ orbit around L2 without expending much fuel. Since these orbits are physically large, satellites in L2 halo orbits are able to communicate with Earth at the same time as they observe operations on the far side of the Moon. In 2019, China became the first nation to use L2 in support of a lunar lander, called the Chang’e 4.
So far, only civilian science spacecraft have ventured into cis-lunar Space. However, the United States’ Air Force Research Laboratory recently announced plans to build two spacecraft to do just that. The first, known as the Cislunar Highway Patrol Satellite (CHPS), will be placed at one of the L points. From there, it will track other spacecraft in cis-lunar Space and lunar orbits.Footnote 78 The second, known as the Defense Deep Space Sentinel (D2S2), is described as a highly manoeuverable spacecraft able to conduct ‘rendezvous/proximity operations’ as well as ‘space object removal and recovery, and other applications in defensive space operations’.Footnote 79
Arguably, any improved Space situational awareness in cis-lunar Space would be a good thing, providing more information as to what other Space actors are doing – and what they are not. Yet such a role could just as easily be fulfilled by civilian spacecraft that could also engage in scientific studies, including detecting and tracking asteroids and comets for the purposes of planetary defence.
As for D2S2, there is nothing inherently wrong with Space debris removal and recovery technology. But such technology is inherently ‘dual-use’ and could be employed to interfere with other spacecraft. For this reason, the necessary and daunting task of cleaning up Space debris should be led by national Space agencies. D2S2 is also suspect because there is no current need for debris removal in cis-lunar Space, and there is unlikely to be any such need for decades to come. The situation in LEO is, of course, quite different, as we explained above.
Perhaps just as important are two closely related questions: (1) where, exactly, would the debris be moved to? And (2) should any individual state be allowed to make these decisions on its own? Answers to these questions will be required at some point, since the two other most feasible options – leaving derelict spacecraft to drift uncontrolled in cis-lunar Space or redirecting them so that they crash into the lunar surface – are unsustainable practices. A further option, sending debris into a heliocentric (i.e. Sun-centred) orbit, might seem like a better idea but would present its own challenges, including that the debris might come back to the Earth–Moon system.Footnote 80
Meanwhile, the Defense Advanced Research Projects Agency (DARPA), within the United States Department of Defense, has announced that it is starting up a Novel Orbital and Moon Manufacturing, Materials and Mass-Efficient Design (NOM4D) program, which ‘seeks to pioneer technologies for adaptive, off-earth manufacturing to produce large space and lunar structures’.Footnote 81 According to DARPA, ‘The NOM4D program will pioneer new materials and manufacturing technologies for construction on orbit and on the lunar surface’. Although the program does not presently include an actual military base, the announcement on DARPA’s website was originally accompanied by an artist’s depiction that included a sizeable building, a landing pad and at least six vehicles – with the building and one of the vehicles sporting the letters DARPA on their sides (see Figure 7.10).Footnote 82
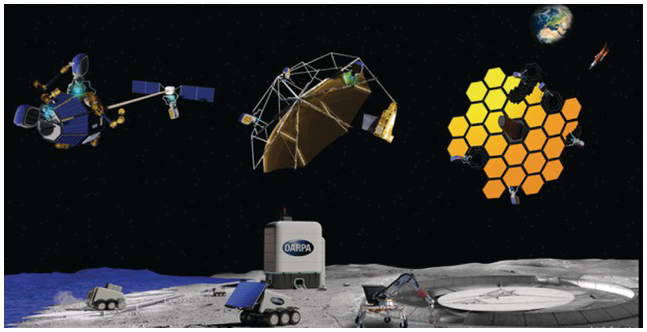
Figure 7.10 Artist’s illustration of plans for a new DARPA program to develop designs and materials for building large structures in orbit and on the moon.
Even if DARPA is not planning to actually engage in surface operations, it is easy to imagine other countries reading the announcement as a statement of intent to militarise the Moon. The NOM4D program will consequently create uncertainty with regard to US plans and therefore, quite possibly, create security dilemmas for both China and Russia. As Jessica West commented, ‘Blurring of civil, military, and commercial capabilities and intentions in space is exactly what the U.S. accuses other countries such as China of doing. It doesn’t build trust, and it doesn’t build confidence. And these two qualities are already in short supply. I don’t see how this ends well.’Footnote 83
Then, in April 2021, DARPA awarded three contracts aimed at the development of a nuclear thermal propulsion (NTP) system for fast, highly manoeuverable, long-duration spacecraft that would be deployed in cis-lunar Space. The contracts, awarded to General Atomics, Blue Origin and Lockheed Martin, are part of the Demonstration Rocket for Agile Cislunar Operations (DRACO) programme. According to programme manager Major Nathan Greiner, it aims to ‘provide agile, responsive maneuverability (potentially across vast distances) within the cislunar domain for a variety of missions’ and ‘conduct space domain awareness within the cislunar domain in a timely fashion’.Footnote 84 As the DARPA website explains,
Rapid maneuver is a core tenet of modern Department of Defense (DoD) operations on land, at sea, and in the air … [The] NTP system has the potential to achieve high thrust-to-weight ratios similar to in-space chemical propulsion and approach the high propellent efficiency of electric systems. This combination would give a DRACO spacecraft greater agility to implement DoD’s core tenet of rapid maneuver in cislunar space.
In other words, it is hoped that nuclear thermal propulsion will provide both high power and high efficiency, and thus manoeuverability and longevity. The plan is to launch the DRACO spacecraft in 2025.
The US military’s plans for cis-lunar Space are being justified as protection for NASA’s Artemis Program,Footnote 85 which will include a Space station in a special type of halo orbit around the Moon as well as permanent human presence on the surface. China is seen as the principal threat to the programme,Footnote 86 even though it is unclear what would motivate that country to interfere with NASA’s activities.
Extracting resources from the Moon, other than rock for construction and water for survival and fuel, is unlikely to be economically viable for quite some time. The remote location and extreme environment combine to make any activities extremely expensive. And while helium-3 and precious minerals do exist on the Moon, their concentrations are so low that large-scale mining and processing would be required. All this suggests that China’s lunar programme is focused on pursuing scientific knowledge and stoking national pride – just as the United States’ Apollo programme did in the 1960s.
US military leaders are also using the perceived threat from China as an argument for developing new rules of lunar access and behaviour from a position of strength. In August 2020, Steven Butow, who leads the Space Portfolio within the Defense Innovation Unit,Footnote 87 said, ‘Much of our law that we follow today is established on precedents. And one of the things we don’t want to do, is we don’t want to let our peer competitors and adversaries go out and establish the precedent of how things are gonna [sic] be done in the solar system, beginning with the Moon.’Footnote 88
However, there is already an extensive body of international law that applies in cis-lunar Space and on the Moon, including the UN Charter and the Outer Space Treaty. Article IV of the latter categorically prohibits all military activities on the Moon and other celestial bodies, with its second paragraph reading,
The moon and other celestial bodies shall be used by all States Parties to the Treaty exclusively for peaceful purposes. The establishment of military bases, installations and fortifications, the testing of any type of weapons and the conduct of military manoeuvres on celestial bodies shall be forbidden. The use of military personnel for scientific research or for any other peaceful purposes shall not be prohibited. The use of any equipment or facility necessary for peaceful exploration of the moon and other celestial bodies shall also not be prohibited.
It should be noted that the second paragraph of Article IV is both detailed and categorical, with military installations, the testing of any type of weapon and military manoeuvres all specifically ‘forbidden’. Article IV’s second paragraph is thus quite different from the references in Articles IX and XI to the ‘peaceful exploration and use of outer space’.
The last two sentences of the second paragraph of Article IV do allow for some military involvement in lunar activities, provided it is limited to the use of personnel, such as US military personnel serving as NASA astronauts. Moreover, facilities and equipment can be established and used on the Moon, provided they are for peaceful purposes only and are not part of a military base, installation or fortification. Thus collaboration between a military and a civilian Space agency does not necessarily contravene Article IV’s second paragraph, while a military carrying out an independent programme on the Moon likely does. As Christopher Johnson explained in the context of the NOM4D programme,
If DARPA (or its contractors) are conducting activities on the Moon which are temporarily peaceful in nature (like refining in situ resources into fuel or other useful material), this is still a MILITARY activity, and therefore pretty clearly prohibited. It’s not done under the banner of NASA, or part of an Artemis program with international partners, or any principally civil activity – it’s just the US military conducting activities, with military aims and objectives in mind. On the surface of the Moon, this is strictly proscribed and prohibited.Footnote 89
We can only conclude that the NOM4D programme is inconsistent with the United States’ legal commitments. In addition to taking steps that risk creating security dilemmas for China and Russia, US military leaders are challenging foundational treaties designed to promote peace and security in international affairs.
7.12.1 Other Risks
The US military’s plans for cis-lunar Space will also create serious risks outside the security domain, including for the future of radio astronomy. TerrestrialFootnote 90 and satellite-borneFootnote 91 signal contamination of radio observations already limits the radio astronomy that can be done from Earth. Interference from Earth orbit is growing, due to the construction of mega-constellations of communications satellites – as discussed in Chapters 2 and 3. Not surprisingly, the idea of placing radio observatories on the far side of the Moon has been around for quite some time and is now seeing concepts in development.Footnote 92 The Breakthrough Listen project is particularly interested in using the Moon as a unique and unspoiled opportunity for conducting search for extraterrestrial intelligence (SETI) science.Footnote 93
However, spacecraft in lunar orbits, orbiting about L points or stationed elsewhere in cis-lunar Space could cause radio interference for these Moon-based observatories in bands that have already been lost to Earth-based facilities. Even a lunar surface-based communication network could cause substantial interference unless designed with the protection of radio astronomy in mind.Footnote 94
Radio interference is a foreseeable issue that could be adequately mitigated, but to succeed in this, all lunar actors will have to respect radio quiet zones. They will also have to limit the number of satellites as well as the portions of the spectrum and the directions of the beams they use. Having militaries racing to position their own spacecraft in cis-lunar Space could complicate this necessarily co-operative exercise.
There are also potential risks involving congestion and debris. Although cis-lunar Space is very large, the locations of greatest utility are restricted in size and therefore have physical carrying limits, even if we do not yet know what they are.
Many of the orbits are unstable and therefore subject to uncontrolled self-cleaning, including halo orbits about L points as well as many lunar orbits. By self-cleaning, we mean that the objects cannot remain at their location without active management and will eventually enter a new orbital trajectory, which could include one that crashes into the Moon or meanders in cis-lunar Space. For this reason, the few lunar orbits that are stable will attract human activity, which could lead to congestion, collisions and debris – creating operational hazards both in those orbits and on the lunar surface. Collisions or fragmentation events will create even more debris, just as they do in Earth orbit. Debris streams could even develop between lunar and Earth orbits. Challenges such as spacecraft disposal in the cis-lunar environment therefore need to be addressed in advance, with safe procedures being followed by all spacefaring states. This situation calls for restraint and close international co-ordination, which competing militaries are rarely able to provide.
7.12.2 Possible Solutions
Space situational awareness in cis-lunar Space should be shared freely, as a public good that will help to prevent accidents. Eventually, some kind of mechanism will be needed to monitor the use of L points and lunar orbits and possibly to assign ‘slots’ to prevent congestion, as occurs in geosynchronous orbit today. International planning for this kind of co-ordination should begin now. At the same time, the US government should terminate the CHPS, D2S2 and NOM4D programmes, or reassign them to NASA to avoid the potentially destabilising militarisation of cis-lunar Space.
The United States should also support the negotiation of a treaty prohibiting weapons in cis-lunar Space, including dual-use technologies operated by militaries. Space has long been a focus for arms control, beginning with the 1963 Limited Test Ban Treaty that prohibited nuclear explosions in Space.Footnote 95 In 1967, the Outer Space Treaty banned all weapons of mass destruction from being stationed in Space and designated the Moon ‘exclusively for peaceful purposes’.Footnote 96 Today, there is a similar opportunity to keep weapons out of cis-lunar Space. The demarcation of Earth orbit and cis-lunar Space provides a clear line upon which such a commitment could be based.
We can still develop the Moon and its associated orbits. But as we have learned from decades of human activity in Earth orbit, developing Space in a sustainable way requires foresight, planning and co-operation. Space must be recognised as an environment that is worth preserving, and as one in which fast-paced alterations can have a myriad of unintended consequences. From avoiding security dilemmas, to maintaining radio quiet zones, to co-ordinating the use of orbital slots, in cis-lunar Space, we still have the chance to get things right.