Introduction
Cryoconite holes are depressions found in the ablation zones of glaciers worldwide. They are often cylindrical depressions which trap wind-blown sediment (Foreman and others, Reference Foreman, Sattler, Mikucki, Porazinska and Priscu2007), often isolated from the atmosphere and surrounding supraglacial hydrological system, being thus influenced by different abiotic and biotic factors (Stanish and others, Reference Stanish, Bagshaw, McKnight, Fountain and Tranter2013). The accumulation of sediment drives the development of the holes, as the sediment absorbs solar radiation in the form of heat, melting the surrounding ice. Along with sediment, the depressions may fill with water from the melting of the glacial ice (Foreman and others, Reference Foreman, Sattler, Mikucki, Porazinska and Priscu2007; Cook and others, Reference Cook, Edwards, Takeuchi and Irvine-Fynn2016). As microhabitats, cryoconites are often somewhat isolated from the atmosphere and surrounding supraglacial hydrological system, being thus influenced by different abiotic and biotic factors (MacDonell and Fitzsimons, Reference MacDonell and Fitzsimons2008; Cameron and others, Reference Cameron, Hodson and Osborn2012).
Cryoconite holes usually exhibit high microbial activity punctuating a spatially extensive aquatic ecosystem within the porous near-surface ice layer (Cook and others, Reference Cook, Edwards, Takeuchi and Irvine-Fynn2016), providing opportunities for microbial evolution (Vincent and others, Reference Vincent2000). Such habitats often harbor a very specialized biological community dominated mostly by cyanobacteria, but with an important contribution from microalgae, heterotrophic bacteria, fungi, and viruses (Hodson and others, Reference Hodson2008), and in some cases also small metazoans. Eolian transportation of local terrestrial or aquatic inoculi are believed to be important contributors to the communities that establish (Cameron and others, Reference Cameron, Hodson and Osborn2012; Vinšová and others, Reference Vinšová2015), forming assemblages that have been compared to the truncated food webs seen in Antarctic lakes (Säwström and others, Reference Säwström, Mumford, Marshall, Hodson and Laybourn-Parry2002), with relatively high alpha diversity levels but scarce number of individuals throughout the water column (Cameron and others, Reference Cameron, Hodson and Osborn2012). The microhabitat appears to favor species that can withstand the extreme changes in physical and chemical conditions characteristic of such harsh conditions (Spaulding and others, Reference Spaulding, Smol and Stoermer2010).
As of 2015, 370 microorganism taxa had been reported from cryoconite holes worldwide, 62 of them belonging to different algal groups (Kaczmarek and others, Reference Kaczmarek, Jakubowska, Celewicz-Gołdyn and Zawierucha2016), Diatoms are one of the frequently reported component, of a different floristic composition compared to the surrounding aquatic environments (Stanish and others, Reference Stanish, Bagshaw, McKnight, Fountain and Tranter2013). Particularly, cryoconite holes are known to support aerophytic, halophytic, epipelic and bryophilic diatom species, suggesting multiple origins of colonizing cells (Yallop and Anesio, Reference Yallop and Anesio2010; Vinšová and others, Reference Vinšová2015), with assemblages comparable to those found in acidic lakes and moss-inhabiting communities reported from high-latitude locations (Yallop and Anesio, Reference Yallop and Anesio2010). Most studies describing cryoconite microbiota have been performed in Arctic/Antarctic glaciers, and to date little attention has been paid to equivalent habitats in the Equatorial region. Low-latitude glaciers are generally smaller and affected by larger diurnal irradiance fluctuations, being also more strongly linked to the terrestrial surrounding land, including anthropogenic perturbations (Cook and others, Reference Cook, Edwards, Takeuchi and Irvine-Fynn2016). Harsh climatic conditions, often combined with low nutrient concentrations and extreme UV radiation levels, usually impede biological processes (Mueller and others, Reference Mueller, Vincent, Pollard and Fritsen2001) even though cryoconite holes may harbor true microbial communities structurally and functionally independent from those in their surroundings (Edwards and others, Reference Edwards2013; Vinšová and others, Reference Vinšová2015). In any case, little is known about biogeochemical processes within such habitats (Foreman and others, Reference Foreman, Sattler, Mikucki, Porazinska and Priscu2007), the processes governing the emergence of a functional communities (Edwards and others, Reference Edwards2013) and the effects of environmental variables on cryoconite communities. This paper addresses this last open question (Mueller and Pollard, Reference Mueller and Pollard2004) by assessing the ecological drivers of diatom communities inhabiting cryoconite holes in Antisana Glacier (Ecuador), testing the hypothesis that assemblages of cryoconite taxa are associated with a combination of abiotic factors.
Materials and methods
Study area
Antisana glacier (0° 28′ S, 78° 9′ W, Fig. 1) is one of the 17 glaciers listed in Hastenrath, Reference Hastenrath1981, on this active volcano located 40 km east of Quito. Facing NW, it receives wind currents from the Amazon River basin. The glacier covers an elevation gradient between 4700 and 5760 m.a.s.l. along 1.96 km. As of 2002, it covered an area of 0.71 km2 (Francou and others, Reference Francou, Vuille, Favier and Cáceres2004). The glacier is located in the Antisana Water Conservation Area (ACHA), managed by the Water Protection Fund (FONAG) within the Antisana Ecological Reserve (REA). Rainfall occurs throughout the year in the form of snow or rain, specially between February and June. The temperature is constant throughout the year but wind and cloud cover show some seasonality, with a peak from April to September (Francou and others, Reference Francou, Vuille, Favier and Cáceres2004).
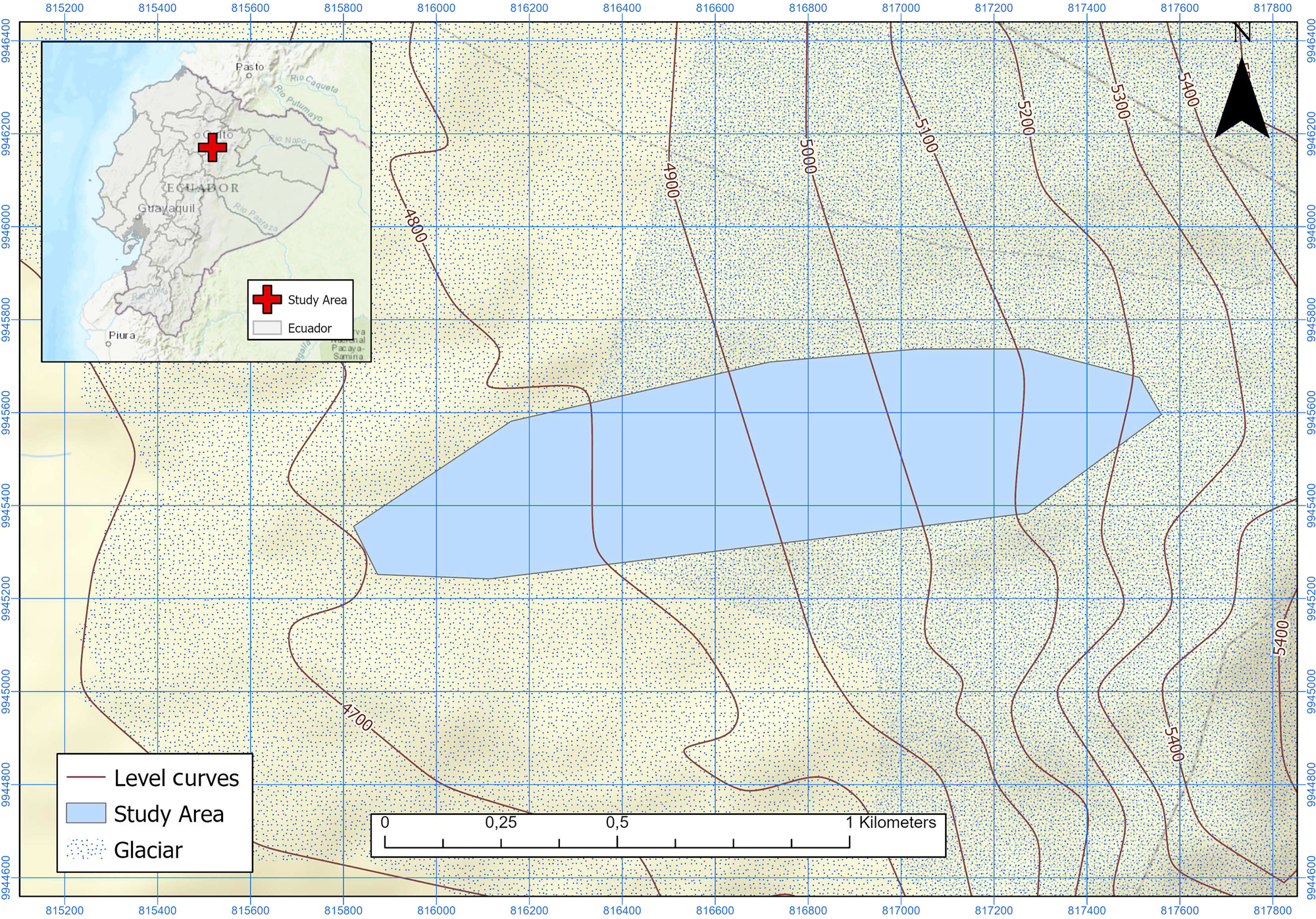
Fig. 1. Study area in Antisana Glacier (Ecuador).
Methodology
From June to December 2018, transects of 200 m in elevation (GPS measurements) were established along the glacier, from 4700 to 5200 m a.s.l. Diatom samples (n = 54) were taken from the cryoconite holes with a frozen surface layer (Fig. 2b) which was broken with a help of an ice axe. Sediment and water were pumped out with a 50 mL syringe and stored in 100 mL sterilized bottles with transeau solution (deionized water, ethanol and formalin in a 6:3:1 ratio), and placed in a cooler Bicudo and Menezes (Reference Bicudo and Menezes2006) at −4°C in complete darkness, then transported to the Limnology Laboratory of the Faculty of Engineering and Applied Sciences of the International University SEK (UISEK). Each cryoconite hole was measured (width, length and depth) and physicochemical variables (temperature, pH, conductivity, alkalinity, hardness, and the concentrations of dissolved oxygen, nitrites and nitrates) were measured in situ with a HANNA® multiparametric probe (Hanna Instruments Czech s.r.o., Czech Republic) and an Tetra Easy Strips® field kit. Samples were processed in the lab by adding 30% hydrogen peroxide and 37% hydrochloric acid at 90°C for 24 h. Permanent microscopy slides were prepared following European standards (ECS, 2003), with the improvements proposed by Blanco and others (Reference Blanco, Álvarez and Cejudo2008). About 400 diatoms were identified (following mainly the collections Bibliotheca Diatomologica, Iconographia Diatomologica and Diatoms of Europe) and counted in each slide with an EUROMEX® PC/DIC light microscope equipped with a CMEX-PRO 18 camera at 1000×.

Fig. 2. Sampling sites (cryoconite holes situated in the ablation zone of the glacier).
Measured variables were divided in three sets hereafter called ‘morphometry’ (hole area, depth and volume), ‘elevation’, and ‘physicochemistry’ (the other physical and chemical variables, see Table 1). The differential response of diatom assemblages to these sets of variables was assessed by means of a nonparametric distance-based multivariate analysis for a linear model using forward selection (DISTLM) (Anderson, Reference Anderson2004), which performs a multivariate multiple regression analysis to test the null hypothesis of no relationship between the predictor and the response variables matrices. The relationship between biotic and abiotic variables was investigated also by redundancy analysis (RDA), which is a multivariate canonical method that assumes a linear response of taxa abundances to environmental gradients (Ter Braak, Reference Ter Braak1994). Diatom counts were Hellinger-transformed (square root of the relative abundance) and singletons (species' occurrence in a single sample) were removed from the dataset prior to analyses. Since the number of individuals varied strongly between sampling units, species richness was estimated (Se) using a nonasymptotic approach based on extrapolation of measured richness with a common sample size (here set to an average abundance of 20 individuals), by means of iNEXT software (Hsieh and others, Reference Hsieh, Ma and Chao2013) that uses the expression (Chao and others, Reference Chao2014):

where N is the number of individuals in the sample, S is the observed richness, and Ni is the number of individuals of the ith taxon. Species diversity was measured by Fisher's alpha because it is insensitive to unequal and/or small sample sizes (Spear, Reference Spear2017). To test the effects of the abiotic predictors (Table 1) on these diversity metrics, we performed a generalized linear model where a minimal adequate model was selected based on a ‘best subsets’ routine using Akaike's information criterion (AIC) (StatSoft, 2012). Variables selected were used as environmental predictors in the RDA analysis (see above). Statistical analyses were conducted using Statistica v. 13.0 (StatSoft, 2012) and PAST v. 4.04 software (Hammer and others, Reference Hammer, Harper and Ryan2001).
Table 1. Descriptive statistics of the abiotic predictors measured in the sampling locations

Results
A total of 278 diatom taxa were identified to the species or subspecific levels, 110 of them could not be assigned to any known species due to the scarce number of individuals present in the samples. Twelve of them likely represented species new to the science. Cryoconite holes contained from 2 to 503 diatoms, with a median of 17.4 valves L−1. Se varied from 1 to 13 taxa per hole and Fisher's alpha values ranged from 0 to 33.19. Dominant taxa included Psammothidium germainii (Manguin) Sabbe, the species complex Planothidium lanceolatum (Brébisson ex Kützing) Lange-Bertalot and Sellaphora atomoides (Grunow) Wetzel & Van de Vijver, which accounted for 29, 12 and 7%, respectively, of counted individuals. In terms of occupancy, these taxa were present in 83, 48 and 44%, respectively, of sampling locations. Abiotic parameters (Table 1), nutrient levels (nitrates, nitrites) were below detection limits in all cases and thus removed from subsequent analyses. Elevation was considered to be bimodal (Fig. 3), with a boundary ~ 4760 m.a.s.l., this division was preserved for RDA and correlation analyses.

Fig. 3. Mixture analysis of elevation data. Minimal AIC scores were obtained for n = 2 sets of measurements.
Statistical analysis showed that elevation is the main driver of diatom assemblages in Antisana cryoconite holes, with a change in composition toward simplified, poorer communities along this gradient. The minimal adequate models found explaining variations in diversity scores across samples included elevation, hole area and volume in the case of Se (AIC: 182.4, p = 0.016 with a identity link function and a Poisson distribution), and only elevation in the case of Fisher's alpha (AIC: 394.9, p = 0.027 with a identity link function and a normal distribution), with no significant contribution from other variables. Both metrics correlated negatively with elevation, but significantly only for sites above 4760 m.a.s.l. (r = −0.96, p = 0.002, Fig. 4). DISTLM analysis revealed that, at the community level, diatoms responded only to elevation (Table 2). Finally, RDA results (adjusted R 2 = 0.03, F = 1.5, p = 0.1, Fig. 5) suggested a difference in assemblage composition between taxa occurring below and above 4760 m.a.s.l.
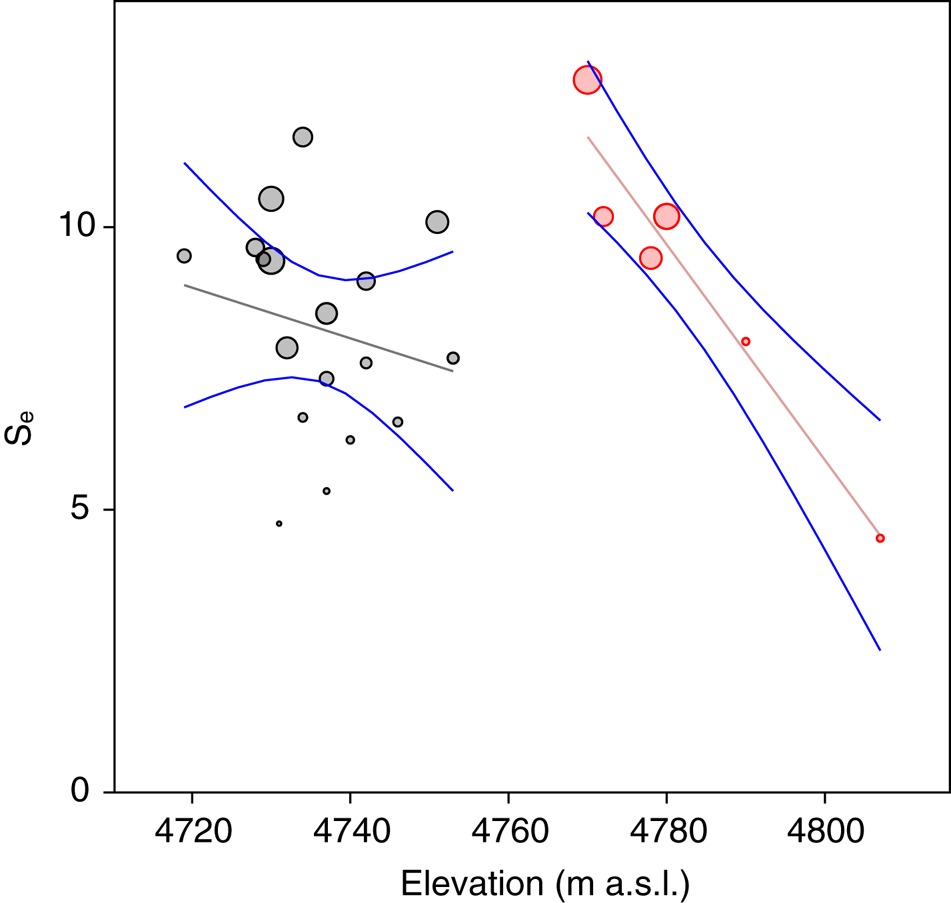
Fig. 4. Expected richness (Se) plotted against elevation. Bubble diameter is proportional to Fisher's alpha value. Gray: locations <4760 m a.s.l. Red: locations >4760 m a.s.l.

Fig. 5. RDA triplot showing the relationship between diatom taxa (codes available in Supplementary material), sampling sites (dots omitted, fitted to 50% confidence ellipses) and abiotic variables (green vectors). Gray: locations <4760 m a.s.l. Red: locations >4760 m a.s.l. EMIN, Eunotia minor; ENS1, Encyonema aff. turgidum; GPNA, Gomphonema punae; LHUN, Lemnicola hungarica; NIPF, Nitzschia paleaformis; NLAN, Navicula lanceolata; OMES, Odontidium mesodon; PBUD, Pinnularia borealis var. undata; PGER, Psammonthidium germainii; PLFR, Planothidium frequentissimum; PMNT, Planothidium minutissimum; PTLA, Planothidium lanceolatum; SEAT, Sellaphora atomoides; SSGR, Sellaphora saugerresii.
Table 2. Results of the DISTLM conditional (sequential) test after running 999 random permutations

Analysis based on Helliger distances. ‘Physicochemistry’ and ‘Morphometry’ include variables 5–10 and 1–3, respectively, in Table 1.
Discussion
Diatom diversity and taxonomic composition
Most studies performed to date, including microscopy, culture-based, and molecular approaches, refer considerable diversity levels in cryoconite microbial assemblages (Cameron and others, Reference Cameron, Hodson and Osborn2012; Edwards and others, Reference Edwards2013; Cook and others, Reference Cook, Edwards, Takeuchi and Irvine-Fynn2016). In particular, diatom richness may contrast with that observed for other algae such as chlorophytes (Säwström and others, Reference Säwström, Mumford, Marshall, Hodson and Laybourn-Parry2002). According to Stanish and others, Reference Stanish, Bagshaw, McKnight, Fountain and Tranter2013, cryoconite holes harbor diatom assemblages with representatives of the regional diatom community but with floristic singularities usually absent in surrounding habitats. Actually, while cosmopolitanism is the common rule in nondiatom cryoconite microbes (Cook and others, Reference Cook, Edwards, Takeuchi and Irvine-Fynn2016), a number of diatom species (e.g. Muelleria cryoconicola) are known to inhabit exclusively cryoconite holes (Van de Vijver and others, Reference Van de Vijver, Mataloni, Stanish and Spaulding2010). The diatom flora found in Antisana cryoconite holes consists on both widespread species (e.g. P. lanceolatum, Odontidium mesodon (Kützing) Kützing) and taxa with restricted geographic distributions. For instance, the most abundant taxon throughout the samples, P. germainii, is a well-known aerophilous species believed to be restricted to the Antarctic and sub-Antarctic regions (Van de Vijver and others, Reference Van de Vijver, Kopalová and Zidarova2016), although rarely reported from cryoconite habitats (e.g. Broady, Reference Broady2005) under ‘Achnanthes cf. germainii’. On the contrary, diatom genera usually found in cryoconite holes such as Hantzschia, Humidophila, Muelleria, Orthoseira (Vinšová and others, Reference Vinšová2015) or Mayamaea (Gesierich and Rott, Reference Gesierich and Rott2012) were scarce or absent in Antisana Glacier.
As in the case documented for the Nordenskiöld Glacier (Spitsbergen, High Arctic) (Vinšová and others, Reference Vinšová2015), the co-occurrence of many different diatom genera but with low abundances may indicate that these assemblages are not true biological communities but merely formed as a result of eolian deposition. However, the dominance of particular species in certain sampling locations (e.g. P. germainii) suggests adaptation to environmental conditions at the community level (Cook and others, Reference Cook, Edwards, Takeuchi and Irvine-Fynn2016). This would imply that the cryoconite habitat favors particular diatom traits, e.g. selecting species that can survive freeze-thaw cycles (Stanish and others, Reference Stanish, Bagshaw, McKnight, Fountain and Tranter2013). In any case, the lack of ecological information about most of the taxa found in our survey prevents any conclusion on this regard.
Environmental factors
Statistical analyses revealed that diatom assemblages in Antisana Glacier did not respond to water chemical conditions, nor the hole size, but varied along a short (100 m) elevation gradient. This finding supports the results described for Qaanaaq Glacier, NW Greenland by Uetake and others, Reference Uetake2016, who found clear altitudinal distribution patterns of microalgae even within a single small glacier. Despite elevation may be mirroring temperature, snow-cover (Mueller and Pollard, Reference Mueller and Pollard2004) or cryoconite stability and age (Vonnahme and others, Reference Vonnahme, Devetter, Žárský, Šabacká and Elster2016), such gradients are very likely absent in the limited sample size covered by our study. Besides, altitude did not correlate with any other measured parameter. In our case, elevation might be merely reflecting the position of sampling locations within the glacier, that is, the distance to surrounding habitats or glacier terminus (Weisleitner and others, Reference Weisleitner2020), which are key determinants of taxonomic composition in cryoconite holes. For instance, Stanish and others, Reference Stanish, Bagshaw, McKnight, Fountain and Tranter2013, described also a decay in species richness along a distance gradient from the coast, confirming that cryoconite diatoms exhibit high spatial variability (Vonnahme and others, Reference Vonnahme, Devetter, Žárský, Šabacká and Elster2016; Weisleitner and others, Reference Weisleitner2020) that persists across a global scale (Cameron and others, Reference Cameron, Hodson and Osborn2012). The independence between temperature and altitude can also reflect the effect of the sampling time range used in this study.
A number of studies, in contrast, show other variables as the major drivers of community structure and composition in these habitats, in particular nutrient levels (Foreman and others, Reference Foreman, Sattler, Mikucki, Porazinska and Priscu2007; Vonnahme and others, Reference Vonnahme, Devetter, Žárský, Šabacká and Elster2016) or hole size (area, depth) (Weisleitner and others, Reference Weisleitner2020). Despite we did not measure particular nutrient concentrations, the lack of response to water conductivity, which is to be considered an excellent proxy of ecological status in freshwaters (Blanco and others, Reference Blanco, Olenici, Ortega, Jiménez-Gómez and Guerrero2020), argues against the consideration of Antisana diatoms as a true biocenosis. Mueller & Pollard (Mueller and Pollard, Reference Mueller and Pollard2004) describe a similar situation in White Glacier (Nunavut Territory, Canada), with an insignificant association of diatom data to abiotic predictors attributed in this case to micro-habitat patchiness (absence of an ice cover and thus a community homogenization).
Conclusion
As in the case studied by Cameron et al. (Cameron and others, Reference Cameron, Hodson and Osborn2012), the reasons for the high taxon diversity observed within Antisana Glacier cryoconite holes are unclear. Our results point to the hypothesis that the diatom assemblages collected may constitute merely eolian deposits from surrounding environments. In this regard, there is still not a robust evidence in favor of any dominant source or mode of delivery (Cook and others, Reference Cook, Edwards, Takeuchi and Irvine-Fynn2016). The most evident result depicted here is the negative correlation between altitude and diatom diversity (not associated with any other measured gradient), that may be reflecting a distance-decay pattern from the source of diatom inoculi. In any case, we agree with Kaczmarek et al. (Kaczmarek and others, Reference Kaczmarek, Jakubowska, Celewicz-Gołdyn and Zawierucha2016) who conclude that, since biological activity within cryoconite holes does contribute to glacier degradation (Wharton and others, Reference Wharton1985), in a context of global warming it is very important to monitor the abundance and diversity of diatoms and other organisms growing on these singular habitats.
Supplementary material
The supplementary material for this article can be found at https://doi.org/10.1017/jog.2021.108
Acknowledgements
The authors are thankful to the ‘Fonag’, EPMAPS” and ‘Ministerio del Ambiente’, Ecuador, for collection permission No. MAE-DNB-CM 2018-0028-0093. The research was funded by Proyecto de Investigación DII-UISEK-P041516_3, (SC) ‘Índice Biótico de Calidad de Agua para el Ecuador’, Universidad Internacional SEK and Convenio Marco de Cooperación entre la Universidad de León, España y La Universidad Internacional SEK, Ecuador. The valuable contribution of the referees and editors is kindly acknowledged.