Book contents
- Developmental Origins of Health and Disease
- Developmental Origins of Health and Disease
- Copyright page
- Contents
- Contributors
- Preface
- Section I Overview
- Section II Exposures Driving Long-Term DOHaD Effects
- Section III Outcomes
- Section IV Mechanisms
- Section V Interventions
- Section VI Public Health and Policy Implications of Interventions
- Index
- References
Section III - Outcomes
Published online by Cambridge University Press: 01 December 2022
- Developmental Origins of Health and Disease
- Developmental Origins of Health and Disease
- Copyright page
- Contents
- Contributors
- Preface
- Section I Overview
- Section II Exposures Driving Long-Term DOHaD Effects
- Section III Outcomes
- Section IV Mechanisms
- Section V Interventions
- Section VI Public Health and Policy Implications of Interventions
- Index
- References
Summary
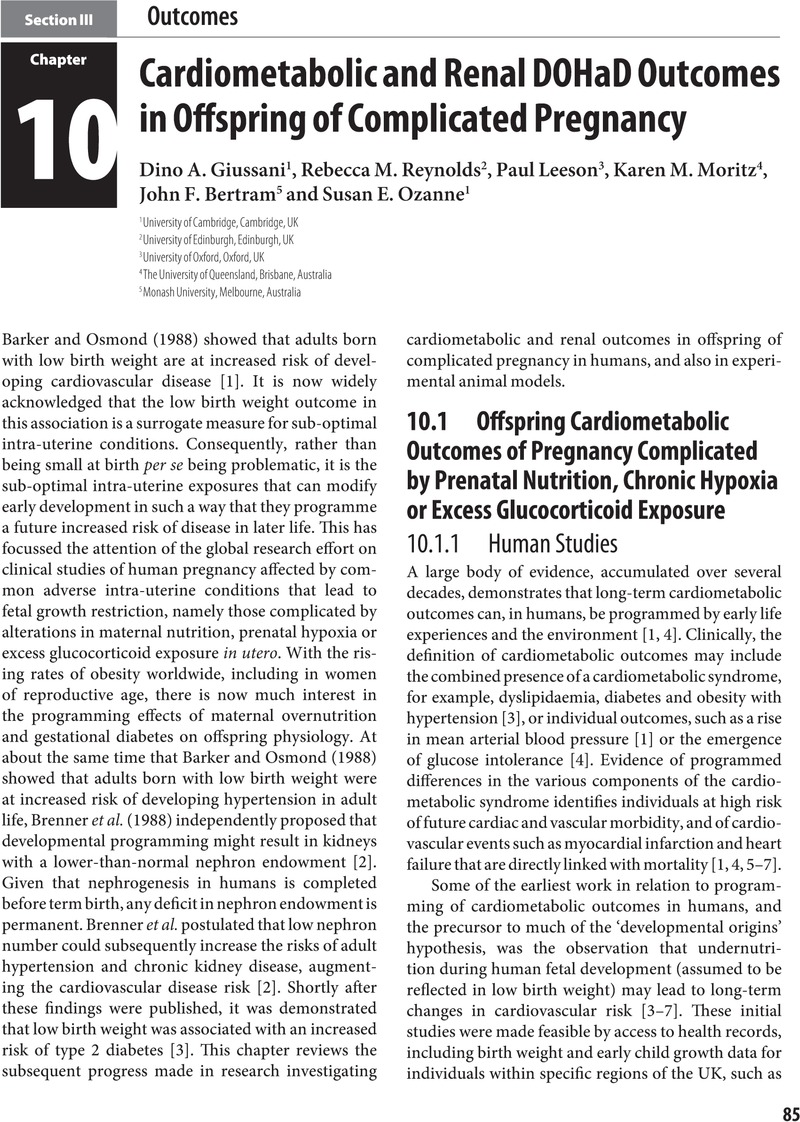
- Type
- Chapter
- Information
- Developmental Origins of Health and Disease , pp. 85 - 131Publisher: Cambridge University PressPrint publication year: 2022