Book contents
- Global Change and Future Earth
- Special Publications of the International Union of Geodesy and Geophysics Series
- Global Change and Future Earth: The Geoscience Perspective
- Copyright page
- Contents
- Contributors
- Preface
- Acknowledgments
- Abbreviations
- Part I Future Earth and Planetary Issues
- Part II Future Earth and Geodetic Issues
- Part III Future Earth and the Earth’s Fluid Environment
- Part IV Future Earth and Regions
- Part V Future Earth and Urban Environments
- Part VI Future Earth and Food Security
- Part VII Future Earth and Risk, Safety and Security
- Part VIII Climate Change and Global Change
- Name Index
- Subject Index
- References
Part II - Future Earth and Geodetic Issues
Published online by Cambridge University Press: 22 October 2018
- Global Change and Future Earth
- Special Publications of the International Union of Geodesy and Geophysics Series
- Global Change and Future Earth: The Geoscience Perspective
- Copyright page
- Contents
- Contributors
- Preface
- Acknowledgments
- Abbreviations
- Part I Future Earth and Planetary Issues
- Part II Future Earth and Geodetic Issues
- Part III Future Earth and the Earth’s Fluid Environment
- Part IV Future Earth and Regions
- Part V Future Earth and Urban Environments
- Part VI Future Earth and Food Security
- Part VII Future Earth and Risk, Safety and Security
- Part VIII Climate Change and Global Change
- Name Index
- Subject Index
- References
Summary
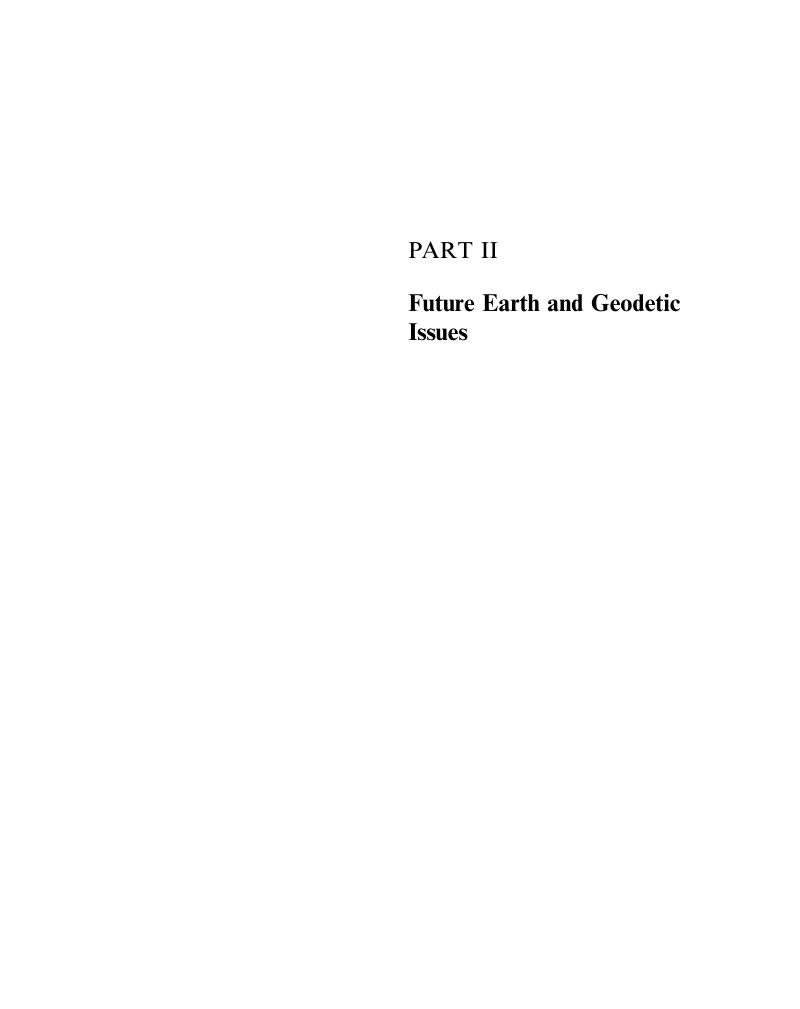
- Type
- Chapter
- Information
- Global Change and Future EarthThe Geoscience Perspective, pp. 55 - 88Publisher: Cambridge University PressPrint publication year: 2018