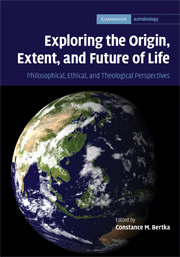
Book contents
- Frontmatter
- Contents
- List of contributors
- Acknowledgements
- 1 Astrobiology in a societal context
- Part I Origin of life
- 2 Emergence and the experimental pursuit of the origin of life
- 3 From Aristotle to Darwin, to Freeman Dyson: changing definitions of life viewed in a historical context
- 4 Philosophical aspects of the origin-of-life problem: the emergence of life and the nature of science
- 5 The origin of terrestrial life: a Christian perspective
- 6 The alpha and the omega: reflections on the origin and future of life from the perspective of Christian theology and ethics
- Part II Extent of life
- Part III Future of life
- Index
- References
2 - Emergence and the experimental pursuit of the origin of life
from Part I - Origin of life
Published online by Cambridge University Press: 29 December 2010
- Frontmatter
- Contents
- List of contributors
- Acknowledgements
- 1 Astrobiology in a societal context
- Part I Origin of life
- 2 Emergence and the experimental pursuit of the origin of life
- 3 From Aristotle to Darwin, to Freeman Dyson: changing definitions of life viewed in a historical context
- 4 Philosophical aspects of the origin-of-life problem: the emergence of life and the nature of science
- 5 The origin of terrestrial life: a Christian perspective
- 6 The alpha and the omega: reflections on the origin and future of life from the perspective of Christian theology and ethics
- Part II Extent of life
- Part III Future of life
- Index
- References
Summary
The origin of life involved many, many emergences.
Harold MorowitzThe experimental investigation of life's origin commenced in earnest more than a half-century ago with the pioneering work of Miller, who synthesized many of life's molecular building blocks under plausible prebiotic conditions. Despite an initial euphoric sense that the origin mystery would soon be solved, scientists quickly realized that the transition from a geochemical to a biochemical world would not easily be deduced by the scientific method.
The great challenge of origins research lies in replicating in a laboratory setting the extraordinary increase in complexity that is required to evolve from isolated molecules to a living cell. The principal objective of this review is to describe some of the efforts by origin-of-life researchers to induce such increases in complexity. A unifying theme of these studies, and hence a useful organizing framework for this review, is the principle of emergence – the natural process by which complexity arises.
Emergence as a unifying concept in origins research
The origin of life may be modeled as a sequence of so-called “emergent” events, each of which added new structure and chemical complexity to the prebiotic Earth. Observations of numerous everyday phenomena reveal that new patterns commonly emerge when energy flows through a collection of many interacting particles.
- Type
- Chapter
- Information
- Exploring the Origin, Extent, and Future of LifePhilosophical, Ethical and Theological Perspectives, pp. 21 - 46Publisher: Cambridge University PressPrint publication year: 2009
References
- 1
- Cited by