Introduction
Toxomerus Macquart, 1855 (Diptera, Syrphidae, Syrphinae), is a New World flower fly genus with most species occurring in the Neotropics (Thompson et al. Reference Thompson, Rotheray, Zumbado, Brown, Borkent, Cumming, Wood, Woodley and Zumbado2010), although Toxomerus floralis (Fabricius, 1798) has been recently introduced in the Afrotropics (Jordaens et al. Reference Jordaens, Goergen, Kirk-Spriggs, Vokaer, Backeljau and de Meyer2015). More than 140 described Toxomerus species are known (Thompson et al. Reference Thompson, Rotheray, Zumbado, Brown, Borkent, Cumming, Wood, Woodley and Zumbado2010), and 39 species have been recorded from Brazil (Borges & Couri Reference Borges and Couri2009; Morales & Marinoni Reference Morales and Marinoni2021). While changes to its taxonomy and inferred evolutionary relationships have been recently accomplished (Metz & Thompson Reference Metz and Thompson2001; Thompson & Thompson Reference Thompson and Thompson2006; Mengual et al. Reference Mengual, Ståhls and Rojo2008, Reference Mengual, Ståhls and Rojo2012; Borges & Couri Reference Borges and Couri2009; Mengual Reference Mengual2011; Thompson Reference Thompson2016), the life history and larval biology of few species have been documented. In common with most tropical Syrphinae species, the larval stages of most Toxomerus species are unstudied, but the species with described larval life histories are either pollen-feeders (Reemer & Rotheray Reference Reemer and Rotheray2009; Nunes-Silva et al. Reference Nunes-Silva, Cordeiro, Obregon, Lima-Neto, Thompson, Viana, Freitas and Kevan2010; Jordaens et al. Reference Jordaens, Goergen, Kirk-Spriggs, Vokaer, Backeljau and de Meyer2015; Dumbardon-Martial Reference Dumbardon-Martial2016) or predaceous on a wide-range of soft-bodied arthropods including aphids, caterpillars, beetle larvae, planthoppers, gall midges, thrips, mealybugs, whiteflies, mites, or insect eggs (Sampson et al. Reference Sampson, Stringer and Spiers2002; Rojo et al. Reference Rojo, Gilbert, Marcos-García, Nieto and Mier2003; Hopper et al. Reference Hopper, Nelson, Daane and Mills2011; Fok et al. Reference Fok, Petersen and Nault2014; Sturza et al. Reference Sturza, Dequech, Toebe, Silveira, Cargnelutti Filho and Bolzan2014; Torres et al. Reference Torres, Lourenção, Costa, Souza, Costa and Tanque2014; Campos et al. Reference Campos, Ueda, Zirondi Filho, Bortolotto, Pasini and Morales2017; Jirón-Pablo et al. Reference Jirón-Pablo, Martínez-Martínez and Sánchez-García2018; Soares et al. Reference Soares, da Silva Paes, de Araújo, de Araújo, Ramos, Picanço and Zanuncio2020).
Observations of Toxomerus basalis (Walker, 1836) larvae and their unusual kleptoparasitic larval behaviour on the sticky leaves of carnivorous sundew plants (Drosera L., Droseraceae) were first reported by Fleischmann et al. (Reference Fleischmann, Rivadavia, Gonella, Pérez-Bañón, Mengual and Rojo2016). The entire larval development of T. basalis, including all instar stages (L1 – L3), occurs on sticky Drosera leaves, where the larvae freely and quickly move between sundew tentacles. They do not adhere to the sticky mucilage, probably because their body is also covered by a thin layer of slime (Fleischmann et al. Reference Fleischmann, Rivadavia, Gonella, Pérez-Bañón, Mengual and Rojo2016). They feed on captured, immobilized insect prey caught by the Drosera leaves, an interaction that can be considered true kleptoparasitism sensu Hamilton (Reference Hamilton2002), as the larvae do not return nutrients to the plant, not even through their excretions, as predatory syrphine larvae do not defecate until they pupate (Rotheray & Gilbert Reference Rotheray and Gilbert2011).
Fleischmann et al. (Reference Fleischmann, Rivadavia, Gonella, Pérez-Bañón, Mengual and Rojo2016) also gave a detailed morphological description of the last larval instar (L3) of T. basalis based on alcohol-preserved material, but did not observe living larvae and adults in situ. In December 2018, additional field observations were made in Minas Gerais, Brazil, by AF and PMG, which revealed new data on the biology of T. basalis, including oviposition, presence of all larval instars, foraging and feeding behaviour of the larvae, and insight into the adult behaviour of both sexes. Presented here is the summary of new data on the biology, behaviour, and entire life history of the larvae and adults of T. basalis, a species endemic to Brazil, increasing knowledge of the kleptoparasitic ‘sundew flower fly’ more than 180 years after the species’ description.
Materials and methods
Larval behaviour of Toxomerus basalis on Drosera leaves was observed by AF and PMG over several days during the 2018 rainy season (early December) at five sites in Minas Gerais, Brazil, both on Drosera species with erect, thread-like leaves (D. spiralis A.St.-Hill and D. magnifica Rivadavia & Gonella), and on flat-rosetted species with semi-erect leaves (D. graomogolensis T.R.S.Silva and D. latifolia (Eichler) Gonella & Rivadavia). Additional observations were made at site 1 by PMG in March 2021 (late rainy season); five adults were collected on that occasion by Danilo Pacheco Cordeiro and deposited at the zoological collection of the Instituto Nacional da Mata Atlântica/Museu de Biologia Professor Mello Leitão (MBML). In total, >50 individuals of T. basalis were studied (12 adults and ca. 40 larvae). All observations were made during clear, sunny weather conditions (with only short rain shower events at sites 1 and 4, during which no flying adults were observed and larvae stayed on the leaves of their host plant). At all sites, adults and larvae were observed from late morning to late afternoon (ca. 11:00 to 17:00). Eggs, larvae, pupae, and adults were documented in situ using a Lumix DMC-FZ50 digital camera (Panasonic, Japan) with macro-filters (achromat close-up lenses +3 to + 8 dioptres, Kenko, Japan, and Raynox, Japan). Short video recordings were made with the same equipment. In addition, simple experiments with freshly captured, living mosquitoes were performed to study the feeding behaviour of T. basalis larvae on Drosera leaves.
Study sites:
1. Brazil: Minas Gerais, Conselheiro Pena municipality, summit of Pico do Padre Ângelo, 19° 19’ 12” S 41° 34’ 42” W, 1530 m a.s.l.
2. Minas Gerais, Diamantina municipality, Distrito de São João da Chapada, Rio das Lajes, 18° 02’ 09” S 43° 47’ 20” W, 1285 m a.s.l.
3. Minas Gerais, Buenópolis municipality, Parque Nacional das Sempre Vivas, 17° 55’ 00” S 43° 47’ 07” W, 1280 m a.s.l.
4. Minas Gerais, Grão Mogol municipality, Estrada Grão Mogol – Montes Claros (MG-307), km 48, 16° 35’ 21” S 42° 54’ 54” W, 730 m a.s.l.
5. Minas Gerais, Botumirim municipality, Rio do Peixe, 16° 55’ 10” S 43° 00’ 28” W, 780 m a.s.l.
Results and discussion
Adults of Toxomerus basalis were observed at study sites 1, 2, and 4, and larval instars 1-3 were recorded at all five study sites in Minas Gerais at upland elevations between 730–1530 m a.s.l. (Figures 1–5). Larvae were found only in larger Drosera populations and exclusively on the large-leaved Drosera species D. graomogolensis, the semi-erect-leaved species D. latifolia, and D. magnifica and D. spiralis, which both have thread-like leaves. Despite exhaustive searches, no Toxomerus larvae or adults were detected on the closely related and morphologically similar thread-leaved D. graminifolia A.St.-Hil., which grows at higher elevations in southern Minas Gerais; the several smaller, sympatric species that occurred at sites 3 and 5 (D. communis A.St.-Hil., D. grantsaui Rivadavia, D. hirtella A.St.-Hil., D. montana A.St.-Hil., and D. tomentosa A.St.-Hil.); the larger-leaved D. ascendens A.St.-Hil., which grows in drier microhabitats than the other species (Gonella et al. Reference Gonella, Rivadavia, Sano and Fleischmann2014). The other sites (1, 2, and 4) each had a single Drosera species, which was inhabited by T. basalis larvae.
Notes on the biology and phenology of Toxomerus basalis adults
Males of many flower fly species guard small territories or lek on or near flowers, on sunlit vegetation, in flight, or close to oviposition sites (Rotheray & Gilbert Reference Rotheray and Gilbert2011). There are several studies that document syrphid territoriality (Fitzpatrick & Wellington Reference Fitzpatrick and Wellington1983), including some species with predatory larvae (Heinrich & Pantle Reference Heinrich and Pantle1975). Toxomerus basalis adult behaviour was similar to other predatory species reported previously. Adult male T. basalis were observed on a suitable perch (taller plants, exposed twigs, grasses and herbs) close to their Drosera host plants (Figure 2i and j). We observed that males regularly stray from their lookout posts to feed at flowers and to observe Drosera plants (presumably searching for females). Females were observed patrolling suitable host plants (Video 1).

Figure 1. Oviposition of Toxomerus basalis. (a) female ovipositing on the tip of the abaxial (lower, non-carnivorous) surface of a developing leaf of Drosera latifolia. (b)–(d) Freshly deposited egg attached to the abaxial (lower) surface of an unfolding leaf of Drosera magnifica. (e)+(f) same female individual as pictured in (a) on plants growing in close proximity to Drosera. (e) Probing a leaf of Ericaulon sp. (Eriocaulaceae) – oviposition did not occur. (f) After oviposition on Microlicia sp. (Melastomataceae), with freshly laid egg visible (left arrow) – the same plant already held another Toxomerus egg (right arrow). (g) Overview of a colony of Drosera latifolia and associated plants, with oviposition sites documented under a, e, and f indicated. Image combined from two different photographs showing two different sides of that vegetation island that was patrolled by the Toxomerus basalis female. a, e–g: Diamantina, Distrito de São João da Chapada, Rio das Lajes, Minas Gerais, Brazil, 06 December 2018. b–d: summit of Pico do Padre Ângelo, Conselheiro Pena, Minas Gerais, Brazil, 04 December 2018. Photos by A. Fleischmann.

Figure 2. Life cycle stages of Toxomerus basalis documented in situ in Minas Gerais, Brazil. (a) first-instar larva L1 (b) L2 larva (c) L3 larva, figures 1a–c to the same scale. (d) L3 larva attaching to pupate on a freshly enrolling Drosera leaf (arrow), note another puparium (arrow with *) on a developing leaf in bud on the same plant. (e) Puparium. (f) L3 larva starting to pupate on a leaf base. (g) Puparium with emerging pharate adult – note the translucent puparium exoskeleton with opening fissures visible at the anterior end. (h) empty puparium exuvia on a developing Drosera leaf. a–c on Drosera spiralis, Parque Nacional das Sempre Vivas, Buenópolis, Minas Gerais, 06 December 2018. d–h on Drosera magnifica, Pico do Padre Ângelo, Minas Gerais, 04 December 2018. (i) + (j) adults resting on exposed perches near their Drosera hosts: (i) female, Rio das Lajes, 06 December 2018. (j) male, summit of Pico do Padre Ângelo, 04 December 2018. Photos by A. Fleischmann.

Figure 3. Biology and feeding habit of Toxomerus basalis larvae on Drosera leaves, documented in situ in Minas Gerais, Brazil (see also video material in the Electronic Appendix to this article). (a) L3 larva hiding in the rosette centre of Drosera upon disturbance. (b) L3 resting on the abaxial leaf tip. (c) L2 larva approaching a captured, still-alive spider, not feeding on it as the prey is not yet fully immobilized. (d) L3 feeding up a small, captured prey item, note where the tentacles have bent around the prey (arrow) but not commenced its digestion, as the tentacles heads have not yet darkened. (e) L3 larva crawling to captured, immobilized nematoceran prey. (f)–(h) L3 feeding on an immobilized but still living nematoceran that had been freshly placed on a leaf of Drosera graomogolensis (tentacles have not yet started bending over the prey), Botumirim, Rio do Peixe, Minas Gerais, 09 December 2018. * indicates anterior respiratory process visible during feeding. a on Drosera latifolia, Rio das Lajes, 06 December 2018. b, d, e on Drosera spiralis, Parque Nacional das Sempre Vivas, 06 December 2018. c on Drosera magnifica, summit of Pico do Padre Ângelo, 04 December 2018. Photos by A. Fleischmann.

Figure 4. Biology of Toxomerus basalis adult flies associated with Drosera, documented in situ in Minas Gerais, Brazil. (a)–(d) + (f) Adult male sheltering beneath a leaf of Drosera graomogolensis during rain shower, Grão Mogol, Minas Gerais, 08 December 2018: (a)–(c) resting and showing cleaning behaviour, (d) leaving its carnivorous refuge by crawling over the sticky (yet rain-washed) leaves. (e) adult female feeding on pollen from a flower of Drosera latifolia, Rio das Lajas, 06 December 2018. Photos by A. Fleischmann.
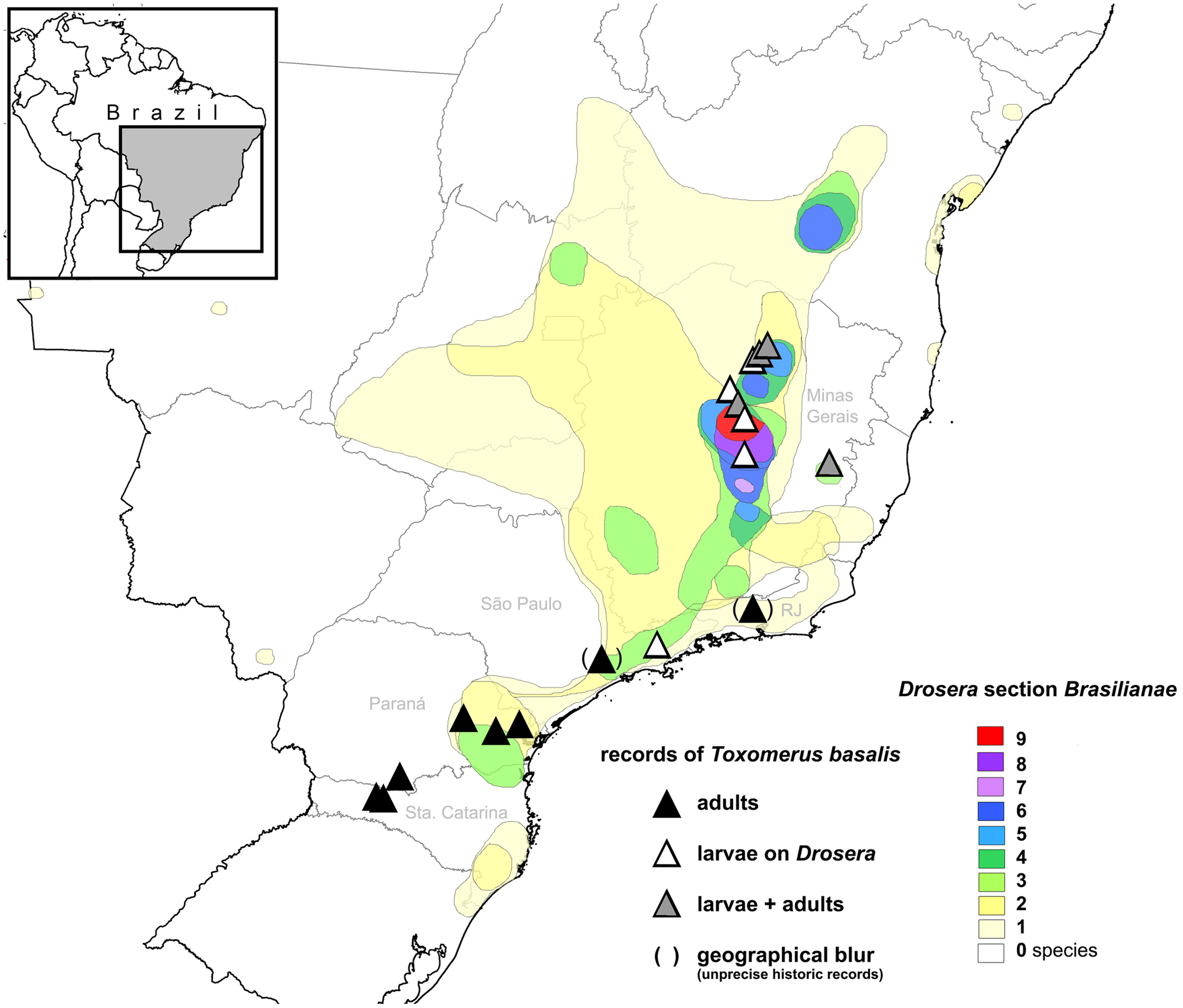
Figure 5. Known distribution of Toxomerus basalis based on museum specimens examined, field studies (AF and PMG), literature records (Borges & Couri Reference Borges and Couri2009; Mengual Reference Mengual2011; Fleischmann et al. Reference Fleischmann, Rivadavia, Gonella, Pérez-Bañón, Mengual and Rojo2016), and database records (SpeciesLink: http://www.splink.org.br/ [accessed 17 February 2021]). Distribution range and species numbers of the confirmed larval host plants from Drosera section Brasilianae indicated (based on Gonella et al. Reference Gonella, Sano, Rivadavia and Fleischmann2022). Map drawn by A. Fleischmann.
Adults of both sexes were regular floral visitors on Drosera flowers where they fed on pollen (Figure 4e). Drosera flowers are a theoretically rather poor food resource for Toxomerus adults. The ephemeral flowers of all Brazilian species lack nectar and open only for a few hours on a single day (AF pers. obs.; Gonella et al. Reference Gonella, Rivadavia, Sano and Fleischmann2014; Cross et al. Reference Cross, Davis, Fleischmann, Horner, Jürgens, Merritt, Murza, Turner, Ellison and Adamec2018). Moreover, T. basalis adults rest on the Drosera plants, searching for shelter among or underneath the sticky leaves (not only ovipositing females, but also males, see Figure 4a–d and f). At site 4, a male adult fly was observed sheltering under a Drosera graomogolensis individual during a rain shower (Figure 4a). It approached the Drosera while AF was taking photographs of the plant and landed directly on an old, rain-washed and hence not-very-adhesive leaf (Figure 4d) before crawling underneath the rosettes, resting on the non-glandular abaxial side during the rain shower, where it groomed and cleaned itself (Figure 4b, c and f).
Toxomerus basalis adults seem to have some capacity to escape entrapment by the sticky Drosera leaves provided they do not come into contact with too many tentacles. When AF was trying to catch an adult male at Pico do Padre Ângelo for closer examination, the fly escaped but one wing briefly came into contact with the sticky tentacles of a Drosera magnifica leaf. It struggled but was able to free itself and move to the safety of the lower surface of the leaf where it cleaned its body, rested, and eventually flew back to its lek.
Interestingly, a female of Toxomerus lacrymosus (Bigot, 1884) was documented sitting on the lower leaf surface of Drosera magnifica at Pico do Padre Ângelo (Figure 6). That image additionally shows a freshly deposited syrphid egg on the leaf above the female. It was impossible to determine whether the egg was laid on the Drosera by this female or by a previous visitor. However, at least some predatory Syrphinae avoid potential oviposition sites where they detect intra- or interspecific competitors to reduce the effect of intraguild interactions (Benestad-Hågvar Reference Benestad-Hågvar1973; Branquart et al. Reference Branquart, Hemptinne, Bauffe and Benfekih1997; Almohamad et al. Reference Almohamad, Verheggen and Habruge2009, Reference Almohamad, Verheggen, Francis, Lognay and Haubruge2010). Accordingly, the presence of an egg close to this T. lacrymosus female suggests either that it was laid by her or that she was not ovipositing. In support of the latter point, it does appear that the adult syrphid was caught by the plant as (exceptional) prey and then escaped to the lower leaf surface (note that its right wing and left abdomen margin are adhering to the mucilage of some Drosera tentacles; Figure 6). If this inference is true, then it is reasonable to infer that the egg and the puparium visible in the centre of that plant both belong to T. basalis (which has been documented from the same location by Fleischmann et al. Reference Fleischmann, Rivadavia, Gonella, Pérez-Bañón, Mengual and Rojo2016 and in this study).
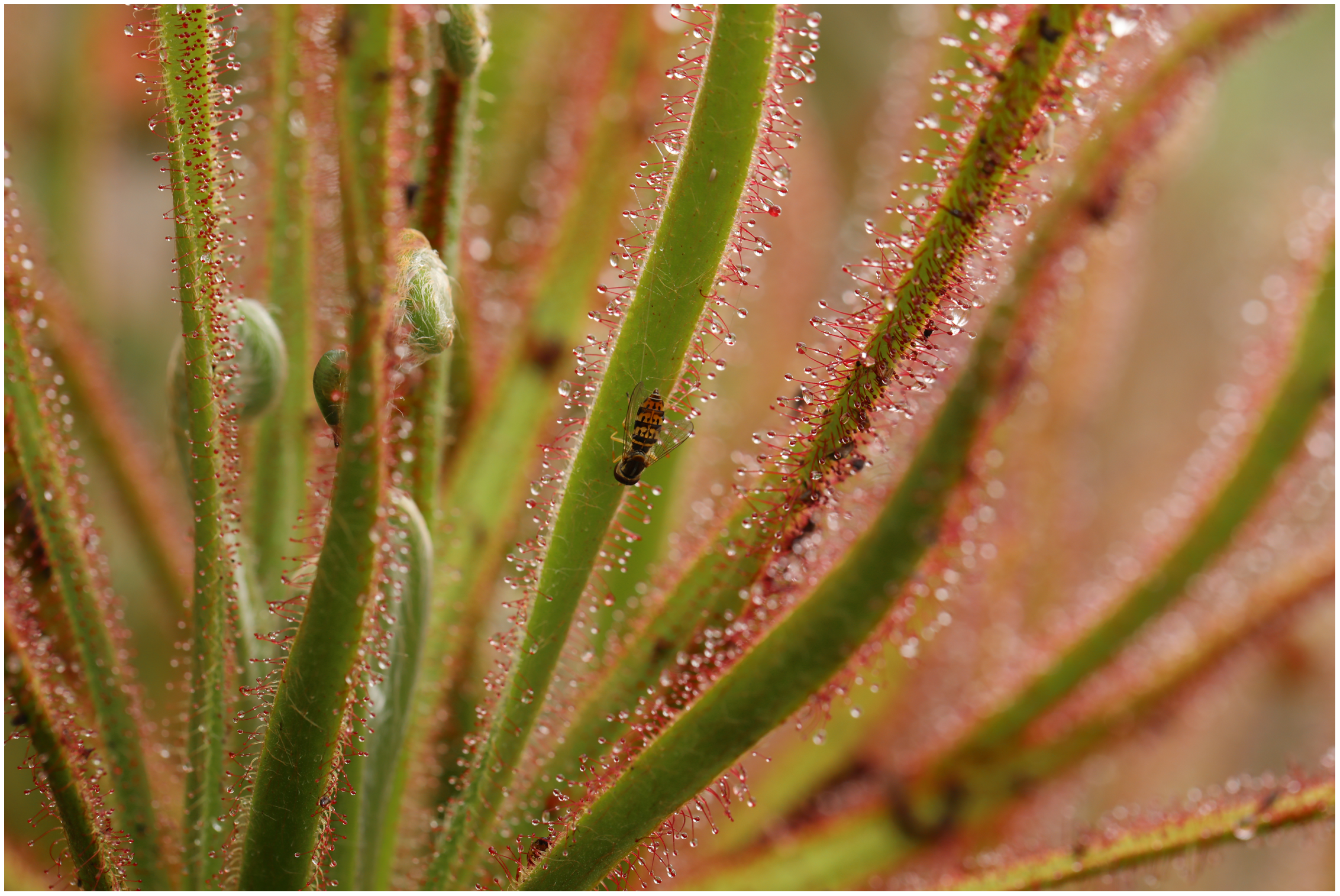
Figure 6. A female of Toxomerus lacrymosus apparently captured by the sticky tentacles of Drosera magnifica on Pico do Padre Ângelo, but which escaped on lower leaf surface. Note a syrphid egg (probably from T. basalis) on the same leaf, right above the female. Photo by L. Medeiros.
Based on field observations (the present study and Fleischmann et al. Reference Fleischmann2016) and collection dates of museum specimens, T. basalis is apparently a multivoltine species with continuous development and multiple generations per year. Adults, eggs, larvae, and pupae were found at the same time in the same habitat, sometimes on the same individual plants. Records and reports of adults and larvae are known from both dry and rainy seasons (Fleischmann et al. Reference Fleischmann, Rivadavia, Gonella, Pérez-Bañón, Mengual and Rojo2016).
Oviposition and female host plant choice
Oviposition behaviour and host plant recognition of T. basalis was unknown to Fleischmann et al. (Reference Fleischmann, Rivadavia, Gonella, Pérez-Bañón, Mengual and Rojo2016) and is reported here for the first time. Five individuals of females laying eggs were observed (two at site 2 in 2018, two at site 1 in 2018, and one at site 1 in 2021). Females oviposit directly on Drosera leaves (observed multiple times at sites 1 and 2 in 2018 and 2021); the eggs are laid individually on the non-sticky adaxial (lower) surface of freshly unfolding juvenile leaves, or leaf buds (Figure 1a–d, Video 2), or occasionally (observed once at site 2) on other vegetation growing next to the Drosera (Figure 1f and g). Exposed leaves seem to be preferred as an oviposition site, as freshly laid eggs and oviposition were observed only on the uppermost, young leaves. A female ovipositing on the lower leaf surfaces of Drosera latifolia at Rio das Lajes, Diamantina (Figure 1a), also probed the leaf of a nearby, glabrous Eriocaulaceae with her abdomen, but did not lay an egg (Figure 1e). She laid on another Drosera leaf and was also observed laying a single egg on a nearby Microlicia sp. (Melastomataceae) with eglandular hairy leaves, which, already had a fly egg identical to that on the nearby Drosera (both plants used for oviposition were in direct physical contact; Figure 1f). The female fly on the host plant was observed alternately extending and withdrawing its proboscis and moving its abdominal tip, presumably to test the suitability of the oviposition site guided by gustatory, visual, and chemical cues, as observed during oviposition in other syrphids (Dixon Reference Dixon1959; Rotheray & Gilbert Reference Rotheray and Gilbert2011).
In Syrphidae, the choice of oviposition sites is the key parameter determining the future survival of offspring, which have a limited dispersal capacity compared with adults (Almohamad et al. Reference Almohamad, Verheggen and Habruge2009), but also to minimize interspecific competition (Almohamad et al. Reference Almohamad, Verheggen, Francis, Lognay and Haubruge2010). It is well-known that members of Syrphinae with predaceous larvae oviposit near their potential prey (e.g., aphid colonies), but also on suitable host plants before any obvious prey colonies have established (Almohamad et al. Reference Almohamad, Verheggen and Habruge2009, and references therein). In predatory Syrphinae, volatiles emitted from the larval prey, but often also from the prey’s preferred host plants, are used as semiochemicals for female oviposition choice and additionally represent effective infochemical sources for the hatched syrphid larvae (Almohamad et al. Reference Almohamad, Verheggen and Habruge2009). In the case of Toxomerus, adults of some species are attracted by methyl salicylate (Rodriguez-Saona et al. Reference Rodriguez-Saona, Kaplan, Braasch, Chinnasamy and Williams2011; Salamanca et al. Reference Salamanca, Souza and Rodriguez-Saona2018; Legaspi et al. Reference Legaspi, Miller, Kanga, Haseeb and Zanuncio2020), a herbivore-induced plant volatile (HIPV); however, Drosera does not emit it, unlike some other carnivorous plants (Jürgens et al. Reference Jürgens, El-Sayed and Suckling2009). Thus, flower fly females are able to recognize and distinguish different plant species while looking for those which their larval prey inhabit. Host plant recognition in predaceous Syrphidae is often multifactorial (Almohamad et al. Reference Almohamad, Verheggen and Habruge2009; Amiri-Jami et al. Reference Amiri-Jami, Sadeghi, Gilbert, Moravvej and Asoodeh2016), but predominantly guided by visual and olfactory cues. Our observations indicate that male and female adults of T. basalis can recognize Drosera plants among surrounding vegetation. Colour vision has been documented for Syrphidae, with food-foraging individuals being especially attracted towards yellowish colours (Peschken Reference Peschken1964; An et al. Reference An, Neimann, Eberling, Algora, Brings and Lunau2018), while ovipositioning aphidophagous species show site recognition for aphid-infested plants which often display chlorotic colouration (Sutherland et al. Reference Sutherland, Sullivan and Poppy2001; Almohamad et al. Reference Almohamad, Verheggen and Habruge2009). Coincidentally, the colour pattern of the leaves of Drosera species (and other carnivorous plants) is quite similar, with bright yellowish-green or reddish tones prevailing in most species, often in sharp contrast with the duller green of the surrounding vegetation. The bright colour pattern of Drosera leaves and those of other carnivorous plants is presumably connected to prey attraction (Darwin Reference Darwin1875; Lloyd Reference Lloyd1942; Zamora Reference Zamora1995; Schaefer & Ruxton Reference Schaefer and Ruxton2008; Fleischmann Reference Fleischmann2016), and this might also be perceivable among Toxomerus adults (Tagawa et al. Reference Tagawa, Watanabe and Yahara2018 even argue that some Syrphidae are able to distinguish Drosera leaves from flowers and other vegetation, apparently avoiding the former). Many carnivorous plants have reddish or pale yellowish-green colour schemes that are surprisingly similar to those of damaged, unhealthy, or stressed plants, and some of these plants may even use that coloration to attract their prey (Lloyd Reference Lloyd1942; Zamora Reference Zamora1995; Schaefer & Ruxton Reference Schaefer and Ruxton2008). This is especially true of reddish trap colours, which were found to enhance the overall trapping success of carnivorous plants, with particular regard to dipteran prey (Schaefer & Ruxton Reference Schaefer and Ruxton2008), while the pale yellowish-green leaves of other carnivorous plants have been shown to effectively attract fungus gnats (Diptera, Mycetophilidae; Zamora Reference Zamora1995). From an evolutionary perspective, the bright yellowish-green or reddish colour of the carnivorous plant leaves, similar to the coloration of some herbivore-infested plants, might have played a role in the switch of a predatory syrphid to a kleptoparasitic habit on a carnivorous plant – by providing a similar stimulus to female oviposition site choice.
Flower flies, including Toxomerus species, are floral visitors of Drosera plants in the Neotropics (Freitas & Sazima Reference Freitas and Sazima2006; AF and PMG, pers. obs.). Smaller syrphids like Toxomerus basalis (with an adult body length of 5.5–6.5 mm; Borges & Couri Reference Borges and Couri2009; Mengual Reference Mengual2011) would easily fall in the prey size spectrum of large-leaved Drosera species (Gibson Reference Gibson1991), yet they are only rarely captured as prey (pers. obs., Murza et al. Reference Murza, Heaver and Davis2006; Tagawa et al. Reference Tagawa, Watanabe and Yahara2018). An avoidance/hesitation strategy of the flower fly with respect to the sticky leaves has been theorized to explain the low number of captured adults of the Drosera pollinating syrphid Sphaerophoria sp. in Japan (Tagawa et al. Reference Tagawa, Watanabe and Yahara2018, identified as ‘Sphaerophoria menthastri Linnaeus, 1758’, but corresponding to Sphaerophoria indiana Bigot 1884; K. Ichige, pers. comm.). Of course, in the case of the kleptoparasitic Toxomerus basalis, the adults do not avoid Drosera, rather, both sexes actively approach even the sticky leaves of sundew plants. Apparently, there is some type of recognition of the sundew plant by those syrphid species studied which show minor pollinator-prey conflict (Murza et al. Reference Murza, Heaver and Davis2006; El-Sayed et al. Reference El-Sayed, Byers and Suckling2016; Tagawa et al. Reference Tagawa, Watanabe and Yahara2018; Ojeda et al. Reference Ojeda, Carrera, Paniw, García-Moreno, Barbero and Palma2021), or simply divergent attractants to leaves and flowers (Cross et al. Reference Cross, Davis, Fleischmann, Horner, Jürgens, Merritt, Murza, Turner, Ellison and Adamec2018).
Remarkably, we observed larvae of T. basalis exclusively on Drosera species from Drosera section Brasilianae Rivadavia, Gonella & A. Fleischm., in accordance with the larval host species list by Fleischmann et al. (Reference Fleischmann2016). This is a monophyletic group of tetraploid Drosera species (Fleischmann et al. Reference Fleischmann, Gonella and Rivadavia2018) with slightly divergent morphology from the sympatric diploid species of Drosera section Drosera. Fleischmann et al. (Reference Fleischmann, Rivadavia, Gonella, Pérez-Bañón, Mengual and Rojo2016) and this study did not find a single Toxomerus larva on species from D. section Drosera. No other Drosera species have been identified as larval hosts of T. basalis beyond those listed by Fleischmann et al. (Reference Fleischmann2016).
Drosera species have a specific chemical composition of secondary plant metabolites, especially among acetogenic naphthoquinones (Culham & Gornall Reference Culham and Gornall1994; Schlauer & Fleischmann Reference Schlauer and Fleischmann2016, Reference Schlauer and Fleischmann2022; Schlauer et al. Reference Schlauer, Carow and Fleischmann2019), but also other volatile compounds, some of which might even be involved in prey attraction in certain species (Fleischmann Reference Fleischmann2016). Nevertheless, the semiochemicals responsible for oviposition site recognition by T. basalis females are indisputably not the characteristic naphthoquinones, as their distribution pattern and presence differ greatly between those species of D. section Brasilianae that have been documented as larval hosts by Fleischmann et al. (Reference Fleischmann, Rivadavia, Gonella, Pérez-Bañón, Mengual and Rojo2016) and in this study: Drosera latifolia and D. villosa A.St. Hil. contain both naphthoquinone regioisomers 7-methyljuglone and plumbagin, while D. spiralis, D. magnifica, and D. × fontinalis Rivadavia contain only the former (while sympatric diploid Drosera species of D. section Drosera contain only the latter), and D. chrysolepis Taub., D. camporupestris Rivadavia and D. graomogolensis are devoid of any acetogenic naphthoquinones (Schlauer et al. Reference Schlauer, Carow and Fleischmann2019; Schlauer & Fleischmann Reference Schlauer and Fleischmann2022). Hence, the naphthoquinone patterns are too variable in the documented host species to serve in host recognition, but other characteristic Drosera volatiles might be perceived by gravid Toxomerus females, allowing them to recognize their larval host plants.
Life history of Toxomerus basalis
As already reported by Fleischmann et al. (Reference Fleischmann, Rivadavia, Gonella, Pérez-Bañón, Mengual and Rojo2016), the larvae spend their entire development as commensals on the sticky leaves of the plant (Figure 2a–c), but they finally pupate attached to the non-sticky lower surface of the carnivorous leaves (Figure 2d–h). Before pupation begins, the larvae settle and empty their gut contents, changing their body colour to a dark brown (Figure 2f), while the puparia themselves are homogeneously bright green (Figure 2d and e; Fleischmann et al. Reference Fleischmann, Rivadavia, Gonella, Pérez-Bañón, Mengual and Rojo2016). This has been interpreted in other Toxomerus species as a form of camouflage (Reemer & Rotheray Reference Reemer and Rotheray2009). As in other Syrphinae, the puparium of T. basalis becomes translucent during preimaginal development and, consequently, the overall appearance is dark blackish-brownish (Figure 2e–f and h; Fleischmann et al. Reference Fleischmann2016). The translucent puparium reveals the adult head just before emergence (Figure 2g).
The duration of the pupal stage of T. basalis is short, between three and five days at most. This was evidenced by puparia observed on Drosera magnifica at Pico do Padre Ângelo in 2018 (temperatures on the summit of Pico do Padre Ângelo during the survey period in early December 2018: min. 12–16°C, max. 22–26°C; PMG pers. obs. and estimated from weather recordings for the region (CPTEC/INPE 2019)). Some of the puparia were attached to the abaxial (lower) surface at the outer part of an enfolding leaf, pointing outwards, with the adult flies inside close to emergence (Figure 2g). The leaves of D. magnifica are circinate in bud (Gonella et al. Reference Gonella, Rivadavia and Fleischmann2015), that is, enrolled like a mainspring (croizer), and a leaf of that Drosera species takes about one week to unfold from bud based on observations of the first author from cultivated material. Thus, the position where the puparium was attached was accessible to the pupating larva no more than about 3–5 days prior to the observation. Note a mature Toxomerus larva ready-to-pupate on an enfolding Drosera leaf and a puparium at a similar position on another leaf in Figure 2d.
Short pupal stages of 3–5 days agree with those reported from another tropical Toxomerus species (Jordaens et al. Reference Jordaens, Goergen, Kirk-Spriggs, Vokaer, Backeljau and de Meyer2015), although they seem to be on the short range for the genus. Based on captive rearing of the likewise tropical Toxomerus dispar (Fabricius, 1794) under lab conditions, the duration of the pupal stage ranged from three to eight days (average = 5.12 days) (M.N. Morales & S.R., pers. obs.).
Biology and feeding behaviour of the larvae
Fleischmann et al. (Reference Fleischmann, Rivadavia, Gonella, Pérez-Bañón, Mengual and Rojo2016) noted that the larvae of Toxomerus basalis usually occurred solitarily on their host plants, but this observation was not corroborated during the most recent field studies, where up to five (1–2 on average) larvae were found, in addition to eggs and puparia on larger host plants (such as large individuals of D. magnifica). The larvae were usually found resting without movement on the leaves, where they are perfectly camouflaged, at least on Drosera species with green laminas (Figures 2a–c and 3b–e; Fleischmann et al. Reference Fleischmann, Rivadavia, Gonella, Pérez-Bañón, Mengual and Rojo2016). On host plants with red leaves, the greenish larvae are more conspicuous, at least to the human eye (Figure 3a and f). The larvae moved quickly on the leaves when disturbed, escaping towards the rosette centre of their host plant wherein they hide, firmly squeezing themselves into the narrow spaces created by enfolding Drosera leaves (Figure 3a). After some time (30 minutes to several hours), the larvae started to crawl back onto the sticky lamina again. The larvae approached freshly captured prey from their resting position (possibly attracted by the vibrations caused by struggling prey on the leaves). Most larvae were observed feeding on larger prey items (similar in size to the larvae or larger) and, more rarely, also on some of the numerous, smaller captured prey (Figure 3d, note that the bent tentacles indicate the position where the almost fully consumed prey was situated). Some larvae were also found foraging across the leaves of their host plant and moving between different sundew individuals in dense populations of their host plants, notably in (most likely clonal) patches of D. graomogolensis at Rio do Peixe, Botumirim (Video 3). This is in line with the normal hunting behaviour observed in starved predatory larvae of other syrphines (Rojo et al. Reference Rojo, Hopper and Marcos-García1996; Vosteen et al. Reference Vosteen, Gershenzon and Kunert2018).
Fleischmann et al. (Reference Fleischmann, Rivadavia, Gonella, Pérez-Bañón, Mengual and Rojo2016) reported that larvae of T. basalis fed on prey captured by the adhesive traps of Drosera and hypothesized that this might happen apparently only after the trapped insects were dead (presumed saprophagy). This assumption was found to be false based on these new, detailed field observations. The larvae appeared to ignore old prey and seemed to feed only on relatively freshly caught insect prey that was not dead, just immobilized (Figure 3c–h). All feeding larvae observed (based on five observations at sites 1, 3, and 5) were eating freshly caught, living prey, rather than dead insects or prey that was already pre-digested by the plant. Moreover, the larvae did not approach moving or struggling prey but waited at some distance on the leaves (a similar behaviour was observed in kleptoparasitic/mutualistic capsid bugs (Hemiptera, Miridae) living on Drosera and Roridula (Roridulaceae); AF pers. obs). Prey was first probed by Toxomerus larvae with their mouthparts (Figure 3c) but not touched further if it still showed some movement. Only fully immobilized prey items were consumed by the larvae (Figure 3f–h; Video 4).
Our new photographic evidence demonstrates that only freshly captured prey were eaten by the Toxomerus larvae, while those prey on which digestion by the plant had commenced were avoided. The colour of the gland head and stalk of the Drosera tentacle darkens through aggregative activity in the cell vacuoles during prey digestion, and this colour change is noticeable even to the naked eye (Darwin Reference Darwin1875; Gardiner Reference Gardiner1886; Huie Reference Huie1897; Lloyd Reference Lloyd1942). This colour change is appreciable in the macro-photographs of Drosera leaves taken in situ in Brazil, where tentacles were bent over captured prey in a state of digestion (pers. obs.), but there is no colour variation in tentacles enclosing arthropod prey fed upon by Toxomerus larvae (Figure 3d–f). The consumed prey, therefore, had to have been freshly captured by the plant. The feeding behaviour of T. basalis larvae on Drosera is documented in Video 4 and does not differ from the feeding behaviour of other predaceous Syrphinae larvae, that is, the head skeleton pierces the prey and the mandibles then macerate the internal tissues, completely sucking out the body fluids (Rotheray & Gilbert Reference Rotheray and Gilbert2011).
Why is there no tentacle reaction induced by the movement of the T. basalis larvae on the Drosera leaves?
Drosera tentacles are thigmonastic (responding to touch or physical contact) and chemonastic + chemotropic (responding to and towards the presence of certain organic compounds), normally reacting quite quickly to the movements (mechanical stimuli) and/or presence (chemical stimuli) of prey on the lamina (Darwin Reference Darwin1875; Pfeffer Reference Pfeffer1885; Lloyd Reference Lloyd1942; Williams & Pickard Reference Williams and Pickard1972).
In a small experimental setup, AF placed small, freshly captured, living mosquitoes on the leaves of Drosera graomogolensis plants at Rio do Peixe to observe the foraging and feeding behaviour of the larvae of Toxomerus basalis. The Drosera tentacles bent around the prey after some minutes (naturally captured prey as well as artificially placed mosquitoes), but not around Toxomerus larvae resting or moving on and among the leaves, directly touching the tentacle stalks and heads. Note mucilage threads between the tentacles, indicating the movement of the Toxomerus larva (Figure 2c). So interestingly, the larval movements on the leaves do not cause the Drosera tentacles to bend.
It is possible that the larvae’s salivary secretions (slime) not only protect them from adhering to the Drosera mucilage (as postulated by Fleischmann et al. Reference Fleischmann, Rivadavia, Gonella, Pérez-Bañón, Mengual and Rojo2016) but also ‘camouflage’ the larvae from being recognized chemically as prey. A combination of electrical signalling and phytohormone signals is responsible for recognition of living prey by mechanical and chemical stimuli on the leaves of Drosera (Krausko et al. Reference Krausko, Perutka, Šebela, Šamajová, Šamaj, Novák and Pavlovič2017). Once prey is sensed by electrophysiological signals, tentacle movement in Drosera is induced by endogenous plant signals from phytohormones like jasmonates (Nakamura et al. Reference Nakamura, Reichelt, Mayer and Mithöfer2013), that is, substances normally involved in plant herbivore defence (Pavlovič & Mithöfer Reference Pavlovič and Mithöfer2019). Numerous insects including dipteran larvae have found ways to overcome the defensive strategies of plants, for instance by producing antagonists for chemical defence (e.g., Alba et al. Reference Alba, Glas, Schimmel and Kant2011; War et al. Reference War, Taggar, Hussain, Taggar, Nair and Sharma2018). Thus, it is theoretically possible that certain chemical compounds present in larval exudates are capable of blocking the transmission of electrical signals in the tentacles or that they inhibit the jasmonate signalling cascade.
However, the absence of a tentacle response to the presence of the flower fly larvae may have a simpler explanation: in his benchmark experiments on carnivorous plants, Darwin (Reference Darwin1875) found that drops of water do not cause any Drosera tentacle movement. It is possible that the fluid larval exudates acts similarly, provided it does not contain significant quantities of organic or inorganic particles. Thus, the salivary exudate used as lubricant for motion (Fleischmann et al. Reference Fleischmann, Rivadavia, Gonella, Pérez-Bañón, Mengual and Rojo2016) potentially has the additional side-effect of pushing the tentacles into a state of insensitivity (A. Pavlovič, pers. comm.); a film of water surrounding the tentacles can cause membrane hyperpolarization, which makes it more difficult to trigger action potentials (Williams & Pickard Reference Williams and Pickard1972). Based on the observation that the tentacles do not respond to the presence of Toxomerus larvae resting or moving on the Drosera leaves, whereby they touch both the tentacle stalks and the sensitive tentacle heads (Figures 2c and 3d–f; Video 3), it is indeed more likely that upstream signalling events from jasmonates are affected, that is, the initial electrical signals (A. Pavlovič, pers. comm.). It should be noted that the tentacle bending clearly visible around a Toxomerus larva pictured in Figure 1b and c of Fleischmann et al. (Reference Fleischmann, Rivadavia, Gonella, Pérez-Bañón, Mengual and Rojo2016) was caused by the photographer accidentally touching the leaf during photography (A. Peres, pers. comm.).
To date, the reported function of the oral secretions of syrphid larvae is to increase velocity as a lubricant during motion of the apodal larvae (Hindayana et al. Reference Hindayana, Meyhöfer, Scholz and Poehling2001; Fleischmann et al. Reference Fleischmann, Rivadavia, Gonella, Pérez-Bañón, Mengual and Rojo2016) and also as a chemical defence against predators (Eisner Reference Eisner1972; Blum Reference Blum1981; Hindayana et al. Reference Hindayana, Meyhöfer, Scholz and Poehling2001; Betz & Kölsch Reference Betz and Kölsch2004). Unfortunately, the chemistry of these secretions has not been studied since their early mention by Eisner (Reference Eisner1972), but it has been assumed to be proteinaceous (Blum Reference Blum1981). If this were true for all syrphid larvae, including Toxomerus basalis, then the salivary secretion would be ill-suited for ‘chemical camouflage’ on Drosera, whose tentacles are able to respond chemonastically and chemotropically to even small concentrations of proteins (Darwin Reference Darwin1875; Lloyd Reference Lloyd1942). These observations suggest that the physical properties of the thin layer of slime covering the larvae could also protect them from causing leaf reactions that initiate the digestion process, as Drosera tentacles are insensitive to the presence of fluid (Darwin Reference Darwin1875; Pfeffer Reference Pfeffer1885).
Another way to prevent tentacle reactions in the Drosera host would be to employ an avoidance strategy, for example through careful movements such as those performed by some other arthropods living on adhesive plants (Voigt & Gorb Reference Voigt and Gorb2010). Pfeffer (Reference Pfeffer1885) established that mere contact with the gland head of a Drosera tentacle does not provoke a response, but that even minute friction on its surface does. Hence, living/moving prey causes much stronger tentacle movement than dead insect bodies placed onto the leaves (Darwin Reference Darwin1875). However, such special avoidance behaviour or careful, adapted motion was not observed in the Toxomerus larvae studied on Drosera leaves, which freely touch both tentacle stalks and touch-sensitive gland heads without effect during their movement (Videos 3, 4; also notice the larval cuticle touching the mucilage-laden tentacle head in Figure 2c).
Generally, the adhesive leaves of Drosera do not seem to be a suitable habitat for other dipteran larvae. Thus, it is apparently not the much-reduced apodal and acephalous dipteran larval habit which protects them. In cultivation experiments, larvae of Drosophila melanogaster Meigen, 1830, which were manually placed on the sticky laminas of Drosera species, were able to move for some time but did cause tentacle reactions, with most of them being immobilized and then digested by the leaves after some time (AF pers. obs.).
Geographic record and possible regionally divergent larval biologies of T. basalis
Toxomerus basalis seems to be limited to southeastern Brazil (Figure 5). Borges & Couri (Reference Borges and Couri2009) reported the species from Rio de Janeiro, São Paulo, and Santa Catarina states in Brazil based on adult specimens studied in natural history collections. Fleischmann et al. (Reference Fleischmann, Rivadavia, Gonella, Pérez-Bañón, Mengual and Rojo2016) provided records of Toxomerus basalis for Minas Gerais and São Paulo states based on larvae observed in situ on Drosera. Additional records of T. basalis from the state of Paraná are presented here from museum specimens housed at the Universidade Federal do Paraná, Curitiba (UFPR) (Figure 5). Based on the occurrence of suitable habitat and Drosera host plants, future records of Toxomerus basalis in the Chapada Diamantina of Bahia state are likely. This area is strongly biogeographically connected to the Espinhaço Mountain Range of Minas Gerais, where most of the larval records of this species have been made so far (Figure 5) and where T. basalis is frequent and common in wet, Drosera-rich campos rupestres habitats (AF and PMG pers. obs., Fleischmann et al. Reference Fleischmann, Rivadavia, Gonella, Pérez-Bañón, Mengual and Rojo2016). Most records of T. basalis overlap with the distributional range of Drosera section Brasilianae (Figure 5), especially with the upland centres (between 600–1500 m a.s.l.) of the section’s species diversity and occurrences of large-leaved species from that lineage (Gonella et al. Reference Gonella, Sano, Rivadavia and Fleischmann2022).
However, the south Brazilian records of T. basalis adults from Nova Teutônia (Santa Catarina state) and adjacent south-central Paraná [based on several museum specimens housed at the National Museum of Natural History (Washington DC, USA), the Canadian National Collections (Ottawa, Canada) and the UPFR] are somewhat puzzling because that area is lowland (ca. 350 m a.s.l.) and far from any known Drosera populations. The closest known record of a Drosera is ca. 200 km away (Gonella et al. Reference Gonella, Sano, Rivadavia and Fleischmann2022) and belongs to a small species, namely D. communis (Drosera section Drosera), not falling within the host range of T. basalis larvae. Additionally, the specimens from Nova Teutônia were collected by the renowned entomologist Fritz Plaumann around his residence in the Upper Uruguay river region far away from any suitable Drosera habitat. Therefore, T. basalis in that region either utilizes a different sticky (non-carnivorous) plant as host for its kleptoparasitic larval habit or has a completely different larval biology. Alternatively, the taxa represent two cryptic flower fly species with different life histories. Interestingly, the records from Nova Teutônia were originally identified by F. C. Thompson as Toxomerus rhea (Hull, 1949), originally described as Mesogramma rhea Hull, 1949. Recently, Borges & Couri (Reference Borges and Couri2009) synonymized T. rhea under T. basalis based on the comparison of type material in a taxonomic revision of the Brazilian species of Toxomerus. They studied three male and one female co-types of Mesogramma rhea and compared male genitalia with the holotype of Syrphus basalis Walker, 1837. Mengual (Reference Mengual2011) examined several specimens from Nova Teutônia and male genitalia compared favourably to the drawings from Borges & Couri (Reference Borges and Couri2009). It seems rather unlikely that adult T. basalis migrate over long distances to find new habitats, such as from Drosera-rich larval habitats several hundred kilometres distant, given the territorial behaviour of both sexes. The most likely explanation of T. basalis adults in Drosera-free habitats in southern Brazil is either a dietary strategy shift of the larvae [such as the one known from Toxomerus floralis with predaceous larvae in the Neotropics, but larvae that are pollinivorous in Africa (Jordaens et al. Reference Jordaens, Goergen, Kirk-Spriggs, Vokaer, Backeljau and de Meyer2015), or as the well-known pollinivorous larvae of T. politus (Say, 1823) that are also capable of feeding on moth eggs (Jirón-Pablo et al. Reference Jirón-Pablo, Martínez-Martínez and Sánchez-García2018)], or – probably more plausibly – the use of a different sticky, insect-trapping plant as larval habitat. This must constitute a glandular non-carnivorous species, as there are no other sticky carnivorous plants in the region (PMG, pers. obs.). More than 100 sticky, non-carnivorous plant genera casually trap insects (‘defensive killing’) and many of them are visited by opportunistic or adapted predatory/saprophagous arthropods (LoPresti et al. Reference LoPresti, Pearse and Charles2015). A single observation of a syrphid larva similar to those of T. basalis was cited by Fleischmann et al. (Reference Fleischmann, Rivadavia, Gonella, Pérez-Bañón, Mengual and Rojo2016) from an adhesive species of the non-carnivorous legume Chamaecrista (L.) Moench, but the species identity of that larva could not be determined. Despite intensive searching in habitats of T. basalis and Drosera during our detailed field studies in Minas Gerais in December 2018, no syrphid larvae were found on other co-occurring plants with adhesive leaves or on inflorescences, including Chamaecrista and Vellozia Vand., which have resinous glands compared to the physically and chemically different polysaccharide mucilage of Drosera. Therefore, based on current knowledge, it is not possible to ascertain whether T. basalis is able to use other glandular plants as larval host.
Supplementary material
To view supplementary material for this article, please visit https://doi.org/10.1017/S0266467422000128
Acknowledgements
We thank Fernando Rivadavia for sharing his initial discovery of this kleptoparasitic relationship, Lucian Medeiros and Luiz França for company to Pico do Padre Ângelo in 2018 and 2021; Mirian N. Morales (Lavras, Brazil) for sharing unpublished information on the duration of the Toxomerus pupal stage; Luciane Marinoni (UFPR) for helpful correspondence confirming the presence of Toxomerus basalis in Paraná; Andrej Pavlovič (Olomouc, Czech Republic) for helpful correspondence on the physiology of Drosera tentacle movement; and Danilo Pacheco Cordeiro for company during fieldwork and information on T. basalis collections deposited at MBML. Alastair Robinson (Melbourne, Australia), the editor, Francis Gilbert, and an anonymous reviewer are thanked for helpful comments on the manuscript. 2018 fieldwork in Minas Gerais by AF and PMG was supported by travel funding from the project ‘SNSB-Innovativ 2018’ by the SNSB – Staatliche Naturwissenschaftliche Sammlungen Bayerns (Bavarian Natural History Collections, Germany) to AF. PMG thanks The Mohamed bin Zayed Species Conservation Fund for financial support for field work at Serra do Padre Ângelo (grant 192522325). Fieldwork was conducted under research permit granted by the Authorization and Information System in Biodiversity (SisBio), from the Chico Mendes Institute for Biodiversity Conservation (ICMBio) (#67026-1) to PMG.