Semiconductor nanocrystals (NCs) are promising materials for applications in light-emitting diodes, field-effect transistors, photodetectors, and solar cells, including generation-III devices that exploit effects such as carrier multiplication. Semiconductor nanocrystals have a variety of novel properties that can be controlled by the dimensions, shapes, and internal structures of the NCs, but poor understanding of charge transport in films generated from these particles hinders progress in realizing NC-based devices. Recently, researchers at Los Alamos National Laboratory, New Mexico, have fabricated optical field-effect transistors (OFETs) based on lead sulfide (PbS) NCs and have used them to elucidate the charge-transport mechanism in the dark and under illumination. Through spectrally resolved studies of gate-voltage–dependent photoconduction, they demonstrated the existence of a mid-gap band (MGB) which plays an important role in charge conductance both in dark and under illumination.
As reported in the September 27 online edition of Nature Communications (DOI: 10.1038/ncomms1492), P. Nagpal and V.I. Klimov fabricated OFET devices, with a channel comprising PbS NCs treated with ethanedithiol, and gold gate and source electrodes (see Figure). While hole injection was much more efficient than electron injection in the dark, illumination of these devices with monochromatic light resulted in a dramatic increase in the source–drain current even at low light intensities, demonstrating a higher mobility of charges responsible for photoconduction compared to carriers responsible for charge transport in dark. In the case of white light illumination, application of a negative gate voltage resulted in an overall increase in photocurrent across the entire spectrum. Simultaneously, two new spectral features at ~0.9 eV and ~1.5 eV were detected in the photocurrent spectrum. These features were explained by the involvement of the “hidden” MGB states.
The researchers concluded that in dark, charges were transported by a weakly conducting network of MGB states. This transport could be explained in terms of either electron or hole conductivity depending on whether the Fermi level was near the bottom or the top of the MGB, respectively. Under illumination, charge transport was dominated by quantum-confined NC states that formed a more conductive network as a result of greater overlap of electronic wave functions. In this case, MGB still played an important role as its occupancy, that could be varied by gate bias, controlled recombination dynamics of the band-edge charges.
Klimov said, “This study has broad implications for electronic and optoelectronic applications of semiconductor NCs, and specifically suggests that design guidelines should be different for devices operating in the dark (diodes and transistors) and under illumination (photodetectors and solar cells).” He further added, “These findings also help to rationalize many previously unexplained observations such as [the]dramatic effect of surface treatments on ‘dark’ conductivity of NC films, relatively weak sensitivity of conductance to size polydispersity of the NCs, and a significant deviation of the photovoltage of NC-based solar cells from the nominal bandgap energy.”
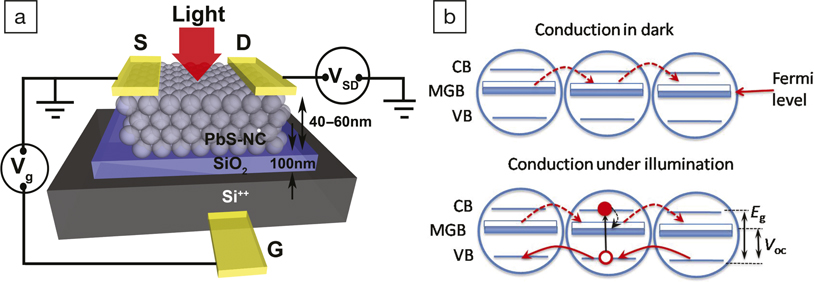
(a) A schematic of the optical field-effect transistor made from PbS nanocrystals (NCs) treated with ethanedithiol. (b) “Dark” conductance in NC films is mediated by mid-gap band states whereas photoconductance is dominated by photogenerated holes transported by quantum-confined NC states. MGB is mid-gap band, CB is conduction band, and VB is valence band. Reproduced with permission from Nat. Commun. (DOI: 10.1038/ncomms1492). © 2011 Macmillan Publishers Ltd.