INTRODUCTION
There is an increasing number of hantaviruses documented in Europe [Reference Vapalahti1–Reference Heyman3] and other parts of the world [Reference Schlegel and Johnson4]. All European hantaviruses are thought to be associated with small mammal hosts such as rodents and shrews [Reference Schlegel and Johnson4]. PUUV seems to be the predominant hantavirus species in Europe, which is possibly due to the wide distribution of its principal host, the bank vole (Myodes glareolus) [Reference Heyman3]. It is one of the hantaviruses that cause clinical symptoms in humans [Reference Settergren5].
A PUUV infection can cause a mild to moderate form of haemorrhagic fever with renal syndrome in humans, designated nephropathia epidemica (NE) [Reference Settergren5]. In Germany, an annual maximum of >2000 human infections has been reported since the disease was declared notifiable in 2001 (SurvStat@RKI 2.0, https://survstat.rki.de). In other European countries such as Finland and Sweden as high as >3000 cases were recorded in peak years [Reference Vaheri6].
In temperate Europe, human NE outbreaks are related to beech (Fagus sylvatica) mast that promotes population outbreaks of bank voles [Reference Reil7–Reference Selva9] due to enhanced food supply and resulting increased survival and reproduction (bottom-up regulation). In contrast, bank-vole population dynamics in Northern Europe are rather predator driven (top-down regulation) [Reference Hanski10]. Beech mast seems to be closely related to climate patterns [Reference Imholt11] and has occurred in the last 15 years about every 2–3 years in Central Europe [Reference Reil7, Reference Hansson, Jedrzejewska and Jedrzejewski12]. In years with high bank-vole density more PUUV-infected bank voles are present [Reference Escutenaire13] and more people become infected [Reference Reil7].
The close relationship of beech mast and bank-vole population dynamics and the effect of the latter on human PUUV infection allow weather-based prediction of the general risk of human PUUV infection. Predictive models can also utilize tree seed mast [Reference Clement8, Reference Tersago14], rodent host abundance [Reference Kallio15, Reference Olsson16], other environmental parameters such as vegetation or land use [Reference Zeimes17, Reference Amirpour18] and recent methodological progress [Reference Zeimes19]. Weather-based models have been developed and provide a robust baseline for alerting health officials and high-risk groups such as forestry workers if a substantially increased infection risk is expected [Reference Imholt11, Reference Reil20].
However, such a predictive approach requires information about the presence of PUUV in regional bank-vole populations because high bank-vole abundance only in combination with the presence of PUUV in the rodent host population elevates the infection risk for humans. Information about PUUV seroprevalence in the host population should be reliable, quick to obtain and based on a non-invasive sample collection. Rapid field tests have been designed to be used in the field to quickly screen rodents for the presence of pathogen-specific antibodies [Reference Sirola21]. They provide the necessary data faster than standard diagnostic approaches such as enzyme linked immunosorbent assay (ELISA) for the detection of PUUV-specific antibodies or genetic analyses for the detection of viral genome. In a study by Sirola et al. [Reference Sirola21] such a test (Reagena, Finland) has been validated for bank voles in Finland and resulted in 100% efficacy. This test is based on the PUUV prototype strain from Finland, Sotkamo (SOT [Reference Brummer-Korvenkontio, Henttonen and Vaheri22, Reference Razanskiene23]). No rapid test exists for German PUUV strains nor have the existing tests been systematically validated for German bank voles. In a pilot laboratory study of German bank voles [Reference Essbauer24] 15 of 18 rapid field test results matched ELISA and Western blot test results using a recombinant antigen of a Swedish PUUV strain (Vranica/Hällnäs, VRA [Reference Dargeviciute25, Reference Reip26]). As test efficacy seems to vary according to geographical origin of the vole samples and the antigens used we aimed to evaluate results of the rapid field test regarding its performance in detecting PUUV-specific antibodies in a field trial with bank voles in Germany. Vole blood samples were screened with the rapid field test and analysed with in-house ELISAs using antigens of SOT, a German strain and the VRA strain.
MATERIALS AND METHODS
Bank-vole populations were sampled 2013–2015 in North Rhine-Westphalia (NW) and Baden-Württemberg (BW) because PUUV is present in both federal states [Reference Hofmann27]. Voles were trapped 2–3 times a year, in spring, summer and autumn in forest sites close to Billerbeck (51° 59′ 38″ N, 7° 18′ 59″ E) in NW and near Weissach (48° 49′ 47″ N, 8° 57′ 58″ E) in BW. Bank voles were captured using 49 Ugglan live-traps (Grahnab, Sweden) set in 10 × 10 m grids and a blood sample of 50 µl was collected from each bank vole from the retro-orbital sinus or facial vein using capillary pipettes (50 µl; Ringcaps®, Germany). Ten microlitres of the blood samples were analysed directly after trapping using the rapid field test for rodents (ReaScan® Ab-Dect Puumala IgG, Reagena, Finland). The diluted blood sample (mixed with IgG-coated gold particles) was transferred into a test cassette where the sample flows across a membrane with bounded PUUV antigen. The ReaScan Reader is designed to display a reading of a value directly proportional to the concentration of PUUV-specific antibodies captured by the membrane (ReaScan Ab-Dect Puumala IgG, Instructions for use version 1.2). Definition of reactivity followed the manufacturer's protocol: cut-off values were <5 (negative), 5–15 (equivocal) and >15 (positive). However, equivocal results were counted as positive for further analyses due to a measurable membrane reaction. Testing was done in a car or nearby building.
The remaining 40 µl of the blood samples were stored at −20 °C until analyses with in-house ELISA (for details see Mertens et al. [Reference Mertens28]), which were done using yeast-expressed recombinant antigens of PUUV strains from Bavaria, Germany (BAWA [Reference Mertens29]), Sweden (VRA) and the homologous SOT strain.
All samples were initially analysed using the German BAWA antigen (n = 341 samples). Additional testing was performed using VRA (n = 104 samples), which was replaced by SOT (n = 237 samples) as soon as SOT was available. Due to restrictions in sample volume not all samples could be tested with all antigens. Equivocal results were regarded positive for further evaluation.
Sensitivity and specificity of the rapid field test compared to the in-house ELISAs were calculated based on the contingency table of the McNemar test. Test efficacy was estimated by calculating the mean of sensitivity and specificity. Optical density determined in ELISAs of positive (and equivocal) samples was compared between BAWA and VRA (n = 10 samples) as well as BAWA and SOT (n = 25 samples) antigens. Data were analysed with a t test using SPSS Statistics for Windows v. 22.0 (IBM Corp., USA).
RESULTS
In NW and BW 111 and 230 bank voles, respectively (total 341) were trapped and blood samples collected. In the rapid field test, 23/341 bank voles were PUUV positive and test results of 11 bank voles were equivocal. As described above, equivocal results of the rapid field test were regarded positive even if the cut-off value for PUUV positive evaluation was not exceeded because a measurable test membrane reaction was recorded. Further, this decision is supported by equivocal results of the rapid field tests corresponding to positive test results in ELISA in 82% of the cases. Hence, we regarded 34/341 animals to be seropositive resulting in a seroprevalence of 10% (Table 1).
Table 1. Contingency table (McNemar test) for validation of rapid field test and in-house ELISAs for detection of PUUV-specific antibodies in bank voles
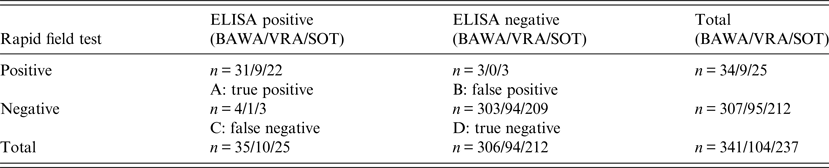
PUUV antigens used for in-house ELISA: BAWA, German strain Bavaria; VRA, Swedish strain Vranica/Hällnäs; SOT, Finnish strain Sotkamo.
In the in-house ELISAs, 35/341 (10%) samples were positive using BAWA, 10/104 (10%) samples using VRA and 25/237 (11%) samples using SOT (Table 1). Thus, PUUV detection using the rapid field test resulted in 7% false positives and 1% false negatives compared to ELISA (mean values in comparison to all three antigens) (Table 2).
Table 2. Evaluation of results of the antibody detection by rapid field test and in-house ELISAs using PUUV strains BAWA, VRA and SOT

BAWA, German strain Bavaria; VRA, Swedish strain Vranica/Hällnäs; SOT, Finnish strain Sotkamo.
Letters A, B, C, D refer to Table 1.
Depending on the PUUV strain used in the ELISA rapid test sensitivity was 88–90% and specificity 99–100%. Test efficacy varied slightly between 93% and 95% according to the PUUV strain used.
The comparison of ELISA test results for anti-PUUV-positive bank voles demonstrated >50% higher optical density values for BAWA compared to VRA antigen (T 9 = 2·88, P = 0·02). In contrast, values were similar for BAWA and SOT antigens (T 24 = 1·90, P > 0·05) (Fig. 1).

Fig. 1. Mean optical density values of the ELISA for detection of PUUV-specific antibodies in bank voles based on positive (and equivocal) samples analysed with antigens BAWA and VRA (n = 10 samples) as well as BAWA and SOT (n = 25 samples). BAWA, German strain Bavaria; VRA, Swedish strain Vranica/Hällnäs; SOT, Finnish strain Sotkamo.
DISCUSSION
Serological PUUV testing of bank voles is needed for scientific investigations as well as for surveillance and public health-related measures. For all these applications, quick and reliable test results are highly beneficial. In this regard, the rapid field test considered here is a suitable tool for German PUUV strains although the test is based on an antigen of the Finnish SOT PUUV strain.
Test efficacy of the rapid field test (93–95%) was similar to the in-house ELISAs, independent on the recombinant antigen used. This is adequate for most purposes of rapid PUUV detection in the field. However, there were slight differences in the reactivity of antigens used in ELISAs (BAWA, VRA, SOT) with blood samples from bank voles in Germany. This may be due to geographical and hence genetic variation of PUUV strains from Finland, Sweden and Germany. Optical density values in ELISAs were almost identical for SOT but considerably lower for VRA compared to values obtained using antigen from the German BAWA strain. This supports a reliable application of the Finnish rapid field test for German bank-vole samples.
Sirola et al. [Reference Sirola21] validated the rapid field test with 105 fresh blood samples collected in Finland at a seroprevalence of 15% and reported 100% sensitivity, specificity and efficacy. The slightly better performance of the rapid field test in their study is most probably due to the perfect match of Finnish bank-vole blood samples and the rapid field test based on the Finnish PUUV strain.
Despite the rapid field test failing to achieve optimal results, PUUV antibodies can be sufficiently detected in field conditions. The use of a rapid field test would facilitate short-term estimation of PUUV seroprevalence in bank-vole populations because laboratory assays testing for the presence of PUUV-specific antibodies or genome are significantly more time consuming and require costly specialized equipment. The rapid field test allows results to be obtained within minutes. The application of the test can be based on a non-invasive sample collection without sacrificing animals, because only a small blood volume (10 µl) is needed for testing. This is not only relevant for optimizing study design in, e.g. long-term monitoring but also for improved animal ethics.
We regarded equivocal test results in the rapid field test and ELISA as positives because in future application of the rapid field test for assessing PUUV presence in bank voles for human health, producing false positives is more acceptable than false negatives.
In conclusion, the use of the rapid field test can aid health authorities in the future to quickly assess the current risk of human PUUV infection based on PUUV seroprevalence in the rodent reservoir in a timely and cost-effective manner. Monitoring strategies for rodents in Germany have recently been published by Jacob et al. [Reference Jacob30]. In addition, harmonized methods for rodent monitoring and diagnostic routines are publicly available via ‘species cards’ (http://www.aphaea.eu/cards/species/voles) and ‘diagnostic cards’ (http://www.aphaea.eu/cards/diagnosis/hantavirosis). This information combined with the use of the rapid field test is useful in providing the data necessary to predict human PUUV infection risk temporally and spatially to support early warnings and preventative action.
ACKNOWLEDGEMENTS
We thank Dörte Kaufmann for technical assistance. Animal experimentation was approved by state authorities (North Rhine-Westphalia: 8.87-51.05.20.09.210 and 84-02.04.2014.A227, Baden-Württemberg 35-9185.82/0261). This study was commissioned and funded by the Federal Environment Agency (UBA) within the Environment Research Plan of the German Federal Ministry for the Environment, Nature Conservation, Building and Nuclear Safety (BMUB) (grant no. 3713 48 401).
DECLARATION OF INTEREST
None.