1. Introduction
The transition between the terminal Ediacaran and early Cambrian at ca. 542–517 Ma recorded an unprecedented biological evolution event on Earth, namely, the ‘Cambrian explosion’, resulting in the rapid diversification of metazoans during this period (Marshall, Reference Marshall2006; Erwin et al. Reference Erwin, Laflamme, Tweedt, Sperling, Pisani and Peterson2011). The Cambrian explosion encompassed a series of episodic radiations. For example, the extinction of Ediacaran fauna occurred near the Ediacaran–Cambrian (E-C) boundary (Laflamme et al. Reference Laflamme, Darroch, Tweedt, Peterson and Erwin2013) and small shelly fauna (SSF), which are represented by the Meishucun Biota and Tommotiid, flourished in the Meishucun stage from ca. 539–521 Ma (Li et al. Reference Li, Steiner, Zhu, Yang, Wang and Erdtmann2007; Zhuravlev & Wood, Reference Zhuravlev and Wood2018). Afterwards, small shelly biota disappeared in the late Meishucun stage; then, Cambrian biota, represented by the Chengjiang biota of the Qiongzhusi stage, increased rapidly at ca. 521–515 Ma (Chen, Reference Chen2009; Fu et al. Reference Fu, Tong, Dai, Liu, Yang, Zhang, Cui, Li, Yun and Wu2019).
Investigation of the chemical environment of the ocean is an important basis for understanding the cause of the radiation of paleontological species, and among them, the reconstruction of redox conditions in the paleo-ocean is a key point. At present, there exist three hypotheses regarding the coevolutionary relationship between oxygenation events and the explosion of Cambrian life: the radiation of Cambrian life caused by a globally oxygenated atmosphere–marine system (Canfield et al. Reference Canfield, Poulton, Knoll, Narbonne, Ross, Goldberg and Strauss2008; Fu et al. Reference Fu, Tong, Dai, Liu, Yang, Zhang, Cui, Li, Yun and Wu2019), complete oceanic oxidation induced by Cambrian fauna and phytoplankton-induced evolution (Lenton et al. Reference Lenton, Boyle, Poulton, Shields-Zhou and Butterfield2014; Xiao, Reference Xiao2012) and no causal relationship (Mills & Canfield, Reference Mills and Canfield2014). In past studies, redox-sensitive elements (e.g., U, V, Mo) and Fe species have been extensively used to show the redox structures in local bottom waters (Algeo & Tribovillard, Reference Algeo and Tribovillard2009; Chang et al. Reference Chang, Chu, Feng, Huang and Chen2018; Li et al. Reference Li, Zhang, Jin, Cheng, Wang, Huang and Algeo2020); however, there are also limitations in applying these parameters (Algeo & Li, Reference Algeo and Li2020; Liu et al. Reference Liu, Magnall, Gleeson, Bowyer, Poulton and Zhang2020). For example, the validity of Fe speciation has been questioned (Clarkson et al. Reference Clarkson, Poulton, Guilbaud and Wood2014), especially in the context of total Fe contents <0.5% (Fan et al. Reference Fan, Wen, Han, Zhu, Feng and Chang2018). As a limiting nutrient for organisms, nitrogen has remarkably different biogeochemical cycling processes and fractionation effects under different redox conditions. Since the biogeochemical process of nitrogen is sensitive to biological activities in the photic zone, the nitrogen isotope information retained in sedimentary organic matter can more effectively reveal the redox conditions of water and the position of the chemocline, as well as paleoproductivity levels (Canfield et al. Reference Canfield, Glazer and Falkowski2010; Devol, Reference Devol2015; Zhu et al. Reference Zhu, Wang, Li, Zhao, Yan, Li and Zhou2021; Wu et al. Reference Wu, Tian, Jia, Li, Li, Zhou and Xie2022). Thus, the application of nitrogen isotopes to determine the marine redox environment has distinct advantages over other common parameters, such as redox-sensitive elements and Fe species. At present, nitrogen isotope studies are increasingly being used in studies of redox conditions in South China; however, the majority of these studies focus on the lower Yangtze Block, which comprises massive shallow-water carbonate facies and basinal facies deposits (Xiaotan in Yunnan, Dingtai in Guizhou, Daotuo in Guizhou, Yinjiang in Guizhou and Yuanjia in Hunan) (Cremonese et al. Reference Cremonese, Shields-Zhou, Struck, Ling, Och, Chen and Li2013; Wei et al. Reference Wei, Ling, Li, Wei, Wang, Chen, Zhu, Zhang and Yan2017; Chen et al. Reference Chen, Diamond, Stüeken, Cai, Gill, Zhang, Bates, Chu, Ding and Lyons2019; Wu et al. Reference Wu, Tian, Jia, Li, Li, Zhou and Xie2022). The middle Yangtze Block sedimentary rocks that record a pattern of the nitrogen cycle within the E-C transition are still less investigated, particularly in the transitional facies; thus, nitrogen isotope studies in this region enable us to more clearly depict the redox structure of the early Cambrian Yangtze Ocean.
To reveal the biogeochemical processes related to the nitrogen cycle process and further unravel the links between environmental changes and early metazoan evolution, we conducted time series measurements of carbon isotopes of the carbonate samples (δ13Ccarb), organic carbon isotopes (δ13Corg) and bulk nitrogen isotopes (δ15N) from a deep borehole in the middle Yangtze Block.
2. Geological setting
The South China Craton was located at approximately 30°N during the early Cambrian (Fig. 1a) and is composed of the Yangtze Block and the Cathaysia Block (Fig. 1b). As a result of the Neoproterozoic rifting event at approximately 820 Ma, the Yangtze Block progressively converted from a single rift basin to a continental margin basin spreading from northwest to southeast during the early Cambrian (Wang & Li, Reference Wang and Li2003); this conversion led to the development of three different depositional patterns, namely, shallow-water platform facies, transition facies and slope-basin facies (Jiang et al. Reference Jiang, Wang, Shi, Xiao, Zhang and Dong2012; Gao et al. Reference Gao, Liu, Jia, Young, Wang, Wang, Zhang and Wang2016) (Fig. 1b). In terms of sedimentary sequences during the E-C transition, the shallow-water facies was dominated by carbonate deposits (e.g., the Dengying and Zhujiajing formations), and the mid- and deep-water slope basin facies were predominantly characterized by siliciclastic deposits (e.g., the Liuchapo and Laopu formations) (Och et al. Reference Och, Cremonese, Shields-Zhou, Poulton, Struck, Ling, Li, Chen, Manning and Thirlwall2016). During Cambrian Stages 2–3, a series of black shales were extensively formed throughout the Yangtze Block under the context of global sea level rise (e.g., Shuijingtuo and Qiongzhusi formations) (Jin et al. Reference Jin, Li, Algeo, Wu, Cheng, Zhang and Shi2020; Chen et al. Reference Chen, Zhang, Jiang, Chen, Zhang, Zhang, Wei, Lu, Chen and Lin2022).

Figure 1. (a) Early Cambrian paleogeography (∼530 Ma; adapted from Li et al. Reference Li, Bogdanova, Collins, Davidson, De Waele, Ernst, Fitzsimons, Fuck, Gladkochub, Jacobs, Karlstrom, Lu, Natapov, Pease, Pisarevsky, Thrane and Vernikovsky2008); (b) paleogeographic reconstruction of the South China Craton during the earliest Cambrian, with the position of the YD-4 borehole presented in the red box (modified from Cremonese et al. Reference Cremonese, Shields-Zhou, Struck, Ling, Och, Chen and Li2013); (c) geological map of the study area (modified from Chen et al. Reference Chen, We, Zhang, Li, Li, Liu and Luo2018).
In this study, samples were obtained from the YD-4 core on the southern edge of the Huangling Uplift in the middle Yangtze Block and were formed in deep shelf palaeogeographical conditions (Chen et al. Reference Chen, We, Zhang, Li, Li, Liu and Luo2018; Zhang et al. Reference Zhang, Xu, Zhou, Ren, Guo and Wang2019). Lithostratigraphically, the E-C transition in the study area includes the Dengying, Yanjiahe and Shuijingtuo formations. Among them, the Dengying Formation is mainly composed of dolomite. The Yanjiahe Formation unconformably overlies the Dengying Formation and is composed of dolomite, calcareous shale, limestone and chert. The Shuijingtuo Formation is in conformable contact with the underlying Yanjiahe Formation and mainly consists of organic-rich shale, argillaceous limestone and limestone lenses.
3. Materials and methods
In this study, 47 samples were taken from the YD-4 borehole (1283–1373 m, coordinates: 11°20′55.5″, 30°18′41.0″) that penetrates the Dengying Formation, Yanjiahe Formation and the lower part of the Shuijingtuo Formation. We measured samples for δ13Ccarb and oxygen isotopes of carbonate (δ18O), δ13Corg, δ15N, as well as the carbonate weight percent, total organic carbon (TOC) and total nitrogen (TN) abundances at the Laboratory for Coastal Ocean Variation and Disaster Prediction of Guangdong Ocean University, China. Concentrations of trace elements were analysed at the Central South Mineral Resources Supervision and Testing Center of the Ministry of Natural Resources (Wuhan, China).
Sample powders (∼1 mg) were subjected to a 24-hour reaction with 100% phosphoric acid to measure the δ13Ccarb and δ18O of carbonates using a Multicarb inlet device in-line with a 253 plus isotope ratio mass spectrometer (Spötl, Reference Spötl2011). The CO2 analyte gas was separated using gas chromatography, and H2O was eliminated using a Nafion trap before being admitted into the mass spectrometer equipped with a continuous flow interface. The international standard NBS-19 was utilized and calibrated to the Vienna Pee Dee Belemnite (V-PDB) scale. The analytical uncertainties for most samples were less than ±0.05‰ for δ13Ccarb and δ18O based on standard analyses and ±0.1‰ based on the reproducibility of replicate analyses.
The fine-grained powders were acidified by 3 M HCl to thoroughly eliminate the carbonate component, and the percentages of calcite were then determined by utilizing these residues. The carbonate-free residues were dried overnight in an oven. The content and isotopic values of organic carbon and TN were analysed via combustion to CO2 and N2 using an EA Isolink series elemental analyser interfaced with a 253 plus isotope ratio mass spectrometer (IRMS). A proper amount of the residues (1∼25 mg) was transferred into a tin capsule and further introduced into a quartz combustion column at 1040 °C. Subsequently, CO2 and N2 entered the IRMS via He carrier gas. The N2 and CO2 isotopic ratios are reported in delta notation in per mil (‰) relative to atmospheric N2 and Pee Dee Belemnite (PDB), respectively. Two carbon isotope standards (urea: δ13C = −28.25‰ ± 0.24‰) were inserted for each set of 10 samples, and the tested carbon isotopes of the standards generated a precision within 0.2‰. The uncertainties were generally better than 0.4‰ based on the reproducibility of replicate carbon isotopic analyses of samples. Two nitrogen isotopic standards (International standard IVA33802153: δ15N = +5.78 ± 0.1‰) were inserted for each set of 10 samples, and the tested nitrogen isotopes of the standards generated a precision within 0.2‰. Uncertainties based on the reproducibility of replicate nitrogen isotopic analyses were less than 0.3‰. The TOC and TN abundances were calculated by multiplying the percentages of carbon and nitrogen with the percentage of residue after acidification.
Trace element abundances were tested using a Thermo Scientific Element XR inductively coupled plasma mass spectrometer (ICP–MS). A proper amount of the homogenized residues (∼40 mg) was reacted with a mixture of HNO3 + HCl + HF in a sealed Teflon cup. The Chinese national standards GSR1 (granite) and GSR3 (basalt) were employed for the purpose of uncertainty detection. The analytical precision was better than 2%.
4. Results
Our high-resolution chemostratigraphic results are presented in Fig. 2 and recorded in Table 1. The TOC abundances from the YD-4 core are typically low, with values in the range of 0.01 to 0.06% in the uppermost Dengying Formation and the lower part of the Yanjiahe Formation, followed by an increasing trend in the middle part of the Yanjiahe Formation (up to 2.54%) and then reaching a peak as high as 16.47% in the overlying Shuijingtuo Formation. Bulk carbonate δ13C analyses display a negative shift from 2.14‰ in the Dengying and basal Yanjiahe formations to −3.86‰ in the middle part of the Yanjiahe Formation, and then the values increase to 1.45‰ over the next 2 m. Within the basal Shuijingtuo Formation, the δ13Ccarb values reach a nadir of ca. −5.47‰. The carbonate oxygen isotopes reveal much less short-term variability in the upper part of the Dengying Formation, with an average of −6‰, and then drop to −10‰ in the middle part of the Yanjiahe Formation followed by a 2% positive excursion in the overlying layers. The δ13Corg profile shows relatively stable values of approximately −29‰ in the Dengying Formation and a smooth negative shift close to −34.4‰ in the middle part of the Yanjiahe Formation. After a recovery of values to −29.8‰ at the top of the Yanjiahe Formation, another negative signal occurs at the boundary with the Shuijingntuo Formation.

Figure 2. Depth profile showing the lithostratigraphic, isotopic and elemental information from the YD-4 borehole in the Yangtze Block.
Table 1. Chemostratigraphic data from the YD-4 core

The TN abundances increase steadily up section from approximately 10 ppm in the Denying Formation to higher values, reaching 709 ppm in the Yanjiahe Formation, and then the values continuously increase to peak values of approximately 2000 ppm in the Shuijingtuo Formation. The δ15N values increase upward from high values of approximately 3.3‰ in the uppermost Dengying Formation to a low value of 0.3‰ in the middle part of the Shuijingtuo Formation; then, the values increase to 4.0‰ over the next 2 m before decreasing monotonically to −1‰ in the upper part of the Yanjiahe and the overlying Shuijingtuo Formation. The C/N values span a range of 5.4 to 141.7, with peaks corresponding to the middle part of the Yanjiahe Formation and the Shuijingtuo shale.
5. Discussion
5.a. Diagenesis
To estimate the diagenetic effect of meteoric water-derived fluids on the study areas, geochemical criteria are applied. The δ18O of carbonate is considered an effective proxy for diagenesis because high oxygen concentrations in diagenetic fluid can significantly affect δ18O (Knauth & Kennedy, Reference Knauth and Kennedy2009; Derry, Reference Derry2010). Typically, δ18O values <−10‰ may present an altered δ13Ccarb composition (e.g., Kah, Reference Kah, Grotzinger and James2000). Our data show that the δ18O values range from −5.2‰ to −10.8‰, with an average of −7.5‰, implying that the δ13Ccarb composition from the YD-4 core probably preserves the primary signal. The noncoincidental observations between δ13Ccarb and δ18O (r = 0.20, n = 31, 0.1 < p < 0.25) in the studied samples may also represent a primary cause rather than diagenesis (Fig. 3a). Indeed, a slight correlation between δ13Ccarb and δ13Corg in the samples (r = 0.30, n = 31, 0.025 < p < 0.05, Fig. 3b) supports this view due to the different diagenetic effects on organic and inorganic carbon (Knoll et al. Reference Knoll, Hayes, Kaufman, Swett and Lambert1986). In addition, as meteoric or burial diagenesis normally raises Mn concentrations and lowers Sr concentrations, Mn/Sr values can also be considered valid proxies for evaluating diagenesis (Veizer, Reference Veizer1983). In carbonate samples, a Mn/Sr ratio lower than 10 is regarded as indicative of the original δ13Ccarb composition (Kaufman & Knoll, Reference Kaufman and Knoll1995). The Mn/Sr values in this study range from 0.1 to 2.9, with an average of 1.3 (Table 1), suggesting weak diagenetic alteration. This result is also in line with the lack of a correlation between δ13Ccarb and the Mn/Sr ratio (r = 0.24, n = 31, 0.05 < r < 0.1, Fig. 3c).

Figure 3. Crossplots of δ13Ccarb vs. δ18O (a), δ13Corg vs. δ13Ccarb (b), δ13Ccarb vs. Mn/Sr (c), TOC vs. TN (d), δ15N vs. Corg/Nbulk (e) and δ13Corg vs. δ15N (f) from the YD-4 borehole. The data are presented in Table 1.
Postdepositional influence can also result in the alteration of nitrogen abundance and possible δ15N. We found that the TOC and TN abundances in this study are positively correlated (r = 0.84, n = 46, p < 0.0005, Fig. 3d), indicating a great contribution of organic molecules to TN samples. In the early diagenetic stage, preferential degradation of 14N-enriched materials (proteins and nucleic acids, etc.) could lead to a slight decrease in the C/N ratio and an increase in the isotopic value of organic nitrogen (Lehmann et al. Reference Lehmann, Bernasconi, Barbieri and McKenzie2002). In addition, if the samples have been influenced by thermal alteration associated with metamorphism, a selective removal of isotopically light N and C and a positive correlation between δ15N and δ13Corg would be expected (Busigny et al. Reference Busigny, Cartigny, Philippot, Ader and Javoy2003; Rivera et al. Reference Rivera, Puckette and Quan2015). However, no clear correlations between δ15N values, C/N ratios and δ13Corg values are observed in this study (Fig. 3e and 3f), indicating that the δ15N composition of the YD-4 core samples was not remarkably influenced by burial fluids.
5.b. Regional stratigraphic correlation
Due to discrete fossil and chronological data, carbon isotope curves are the most reliable tool for correlations of data in the E-C transition. Although the comparisons of δ13C stratigraphic curves demonstrate spatial heterogeneity in the Yangtze Block (Okada et al. Reference Okada, Sawaki, Komiya, Hirata, Takahata, Sano, Han and Maruyama2014; Chen et al. Reference Chen, Diamond, Stüeken, Cai, Gill, Zhang, Bates, Chu, Ding and Lyons2019), which was probably associated with the poor communication between the shallow water and the outer sea or with the missing Cambrian strata, a broad correlation has been shown in previous cases (e.g., Yuan et al. Reference Yuan, Cai, Wang, Xiang, Jia and Chen2014; Jin et al. Reference Jin, Li, Algeo, Planavsky, Cui, Yang, Zhao, Zhang and Xie2016; Wang et al. Reference Wang, Ling, Struck, Zhu, Zhu, He, Yang, Gamper and Shields2018). To trace carbon isotope anomalies that are closely related to the evolution of Cambrian fauna in the cores in this study and to evaluate the possible link between environmental evolution and biological events during the early Cambrian, the correlations of the stratigraphic framework in typical sections of the Yangtze Block as well as larger regions should be considered.
Many well-preserved outcrops that contain the E-C boundary have been observed in the Yangtze Block. Ishikawa et al. (Reference Ishikawa, Ueno, Komiya, Sawaki, Han, Shu, Li, Maruyama and Yoshida2008) conducted a high-precision carbon isotope chemostratigraphic study in the Yanjiahe section, which is situated in the Three Gorges area. The general features of the carbon isotope evolution curves obtained from our samples are generally comparable with those from the Yanjiahe section (Fig. 4). There are at least two negative carbonate carbon isotope anomalies (N1 and N2) and one positive anomaly (P1) that can be identified in the study section. The first negative δ13Ccarb excursion of ∼5‰ (N1) occurred in the lower part of the Yanjiahe Formation at the beginning of the Cambrian, and this negative shift can also be correlated with that in the contemporaneous successions in the Xiaotan section (Fig. 4), as well as the Meishucun (Brasier et al. Reference Brasier, Magaritz, Corfield, Huilin, Xiche, Lin, Zhiwen, Hamdi, Tinggui and Fraser1990) and Laolin (Shen & Schidlowski, Reference Shen and Schidlowski2000) sections. However, the minimum of ∼−4‰ in our drill core study is moderately higher than that from other sections. This discrepancy might result from multiple reasons, including the sampling resolution and/or diagenetic effect. In addition, SSF assemblages are irreplaceable for correlations of the pretrilobitic Cambrian (Terreneuvian). Previous works have evaluated the SSF associations of the Yanjiahe Formation in several typical sections of Yanjiahe village in the Three Gorges area. The Yanjiahe Formation, in turn, contains the A. trisulcatus–P. anabarica assemblage zone, the P. antiqua assemblage zone and the A. yanjiaheensis assemblage zone (Chen, Reference Chen1984; Steiner et al. Reference Steiner, Li, Qian, Zhu and Erdtmann2007; Guo et al. Reference Guo, Li and Li2014), implying that the Yanjiahe Formation is early to mid-Meishucunian in age. However, SSF associations of the Yanjiahe Formation were reported from other outcrops in the Three Gorges area, rather than from the present study core. Microscopic observations of YD-4 core samples showed only a few SSF assemblages (Cao et al., Reference Cao, Wang, Dong, Xiao, Hu, Chen, Wei, Chen, Song and Wu2023), which failed to establish a continuous evolutionary association of SSF assemblages. Due to certain limitations in studying biostratigraphy based on core samples, we admit that there is uncertainty regarding the age of the lower part of the study section. Instead, we can only pay more attention from the perspectives of stratigraphic constraints and comparable carbon isotope stratigraphy. In the stratigraphic sequence of the YD-4 core, the dolorudite of the Yanjiahe Formation conformably contacts the underlying dolomite of the Dengying Formation. Thus, N1 in the YD-4 core is the first positive excursion at the base of the Cambrian. Analogously, in the Xiaotan section, a conformable contact is recognized between the Dengying Formation and the overlying Zhujiaqiong Formation, and the first negative excursion at the base of the Cambrian is believed to be the Basal Cambrian Carbon Isotope Excursion (BACE, negative δ13C values, Cremonese et al. Reference Cremonese, Shields-Zhou, Struck, Ling, Och, Chen and Li2013). Thus, we speculate that the N1 event recognized in the lower part of the Yanjiahe Formation in this study core may correspond to the BACE.

Figure 4. Stratigraphic correlation framework comparing the section in this study, the Yanjiahe section (Ishikawa et al. Reference Ishikawa, Ueno, Komiya, Sawaki, Han, Shu, Li, Maruyama and Yoshida2008; Okada et al. Reference Okada, Sawaki, Komiya, Hirata, Takahata, Sano, Han and Maruyama2014), the Xiaotan section (Cremonese et al. Reference Cremonese, Shields-Zhou, Struck, Ling, Och, Chen and Li2013) and the Morocco section (Maloof et al. Reference Maloof, Ramezani, Bowring, Fike, Porter and Mazouad2010).
Globally, many profiles also have the characteristic δ13C excursion that began in the terminal Ediacaran and reached the lowest point at the basal E-C boundary, including in Morocco (Maloof et al. Reference Maloof, Ramezani, Bowring, Fike, Porter and Mazouad2010, see Fig. 4), the Mackenzie Mountains in NE Canada (Narbonne et al. Reference Narbonne, Kaufman and Knoll1994), the Anabar Platform in NE Siberia (Kaufman et al. Reference Kaufman, Knoll, Semikhatov, Grotzinger, Jacobsen and Adams1996) and SW Mongolia (Brasier et al. Reference Brasier, Shields, Kuleshov and Zhegallo1996). These layers all document the first occurrence of the SSF assemblage, and thus, this negative δ13Ccarb excursion event is also considered a global marker of the E-C boundary. Note that the tuffaceous bed in Oman provided a U–Pb age of 542 ± 0.3 Ma (Amthor et al. Reference Amthor, Grotzinger, Schröder, Bowring, Ramezani, Martin and Matter2003), which is consistent with the lowest δ13Ccarb value, further confirming the global carbon cycling response at the E-C boundary.
In the YD-4 records, the δ13C values show another rapid negative excursion reaching −9‰ (N2) across the boundary of the Yanjiahe-Shuijingtuo formations. The nano-SIMS U–Pb age of the base of the Shuijingtuo Formation in the Three Gorges area was estimated to be 526.4 ± 5.4 Ma (Okada et al. Reference Okada, Sawaki, Komiya, Hirata, Takahata, Sano, Han and Maruyama2014). A previous study showed that the Shuijingtuo Formation was characterized by Hupeidiscus and Tsunyidiscus (Xue & Zhou Reference Xue and Zhou2006), and Zhu et al. (Reference Zhu, Zhang, Yang, Li, Steiner and Erdtmann2003) also recognized the Atdabanian stage-type trilobites in the upper part of the Shuijingtuo Formation (Zhu et al. Reference Zhu, Zhang, Yang, Li, Steiner and Erdtmann2003). Trilobites first occurred at the base of the Shuijingtuo Formation (Guo et al. Reference Guo, Li and Li2014), suggesting that the lower part of the Shuijingtuo Formation likely coincides with the latest Meishucunian age, which is comparable to the Tommotian age of the Siberian platform (Kouchinsky et al. Reference Kouchinsky, Bengtson, Runnegar, Skovsted, Steiner and Vendrasco2012). Moreover, such a large negative isotope excursion (∼5‰ in this study) was reported in the Xiaotan, Morocco and Oman sections (Fig. 4), suggesting a global perturbation of the seawater carbon cycle. Based on the chemo- and biostratigraphic evidence and the conformable contact between the Yanjiahe and Shuijingtuo formations in the Three Gorges area, we speculate that the N2 event recognized across the Yanjiahe and Shuijingtuo Formation boundary corresponds to the Shiyantou Carbon Isotope Excursion (SHICE, negative δ13C values) that developed during the latest Cambrian Stage 2.
A positive excursion of +6‰ in the middle part of the Yanjiahe Formation was detected in the study core (P1). High-resolution carbon isotope evidence shows that the Zhujiaqing Carbon Isotope Excursion (ZHUCE, positive δ13C values) was the last positive excursion in the continuous carbon isotope perturbations between the BACE and the SHICE during the early Cambrian period (Ren et al. Reference Ren, Zhong, Gao, Li, Cao, Wang, Dong and Yan2019). Combined with chemostratigraphic information of the Yanjiahe Formation, the P1 event in this study core can be correlated with the ZHUCE in the lower part of Cambrian Stage 2. This carbon isotope anomaly also exists in other sections in the Yangtze Block, such as the Xiaotan (Fig. 4), Meishucun (Brasier et al. Reference Brasier, Magaritz, Corfield, Huilin, Xiche, Lin, Zhiwen, Hamdi, Tinggui and Fraser1990), Maidiping (Brasier et al. Reference Brasier, Magaritz, Corfield, Huilin, Xiche, Lin, Zhiwen, Hamdi, Tinggui and Fraser1990) and Laolin (Shen & Schidlowski, Reference Shen and Schidlowski2000) sections. However, the feature of P1 in the YD-4 core is defined as a shorter duration and a lower maximum δ13C value (∼1.5‰) relative to other sections, which are probably due to an unconformity between the dolorudite and the overlying dark shale of the Yanjiahe Formation in the study area.
5.c. Evaluation of δ15N signals during the earliest Cambrian
Nitrogen cycle patterns in the modern ocean are important for revealing the nitrogen biogeochemical pathways preserved in sedimentary rocks (Fig. 5). The most significant source of biological nitrogen in the ocean comes from nitrogen fixation, which enzymatically converts atmospheric N2 into biologically available nitrogen (NH4 +) through diazotrophy (mainly cyanobacteria) (Sigman et al. Reference Sigman, Karsh, Casciotti, Steele, Thorpe and Turekian2009). The most common nitrogenase enzyme for nitrogen fixation (e.g., Mo- and Fe-based enzymes) induces only a minor isotope fractionation of approximately −1‰ (εproduct-reactan = −2‰ to 1‰) (Stüeken et al. Reference Stüeken, Kipp, Koehler and Buick2016). Direct assimilation of NO3 − and NH4 + is another way to capture biologically available nitrogen in the ocean, and incomplete assimilation of NH4 + and NO3 − can yield isotopic fractionations of ∼−4‰ to −27‰ and −5‰ to −10‰, respectively (Vo et al. Reference Vo, Inwood, Hayes and Kustu2013; Casciotti, Reference Casciotti2009). However, this scenario occurs only under extremely high nutrient levels, for instance, eutrophication conditions at high latitudes and upwelling systems in the equatorial Pacific Ocean (Sigman et al. Reference Sigman, Karsh, Casciotti, Steele, Thorpe and Turekian2009).
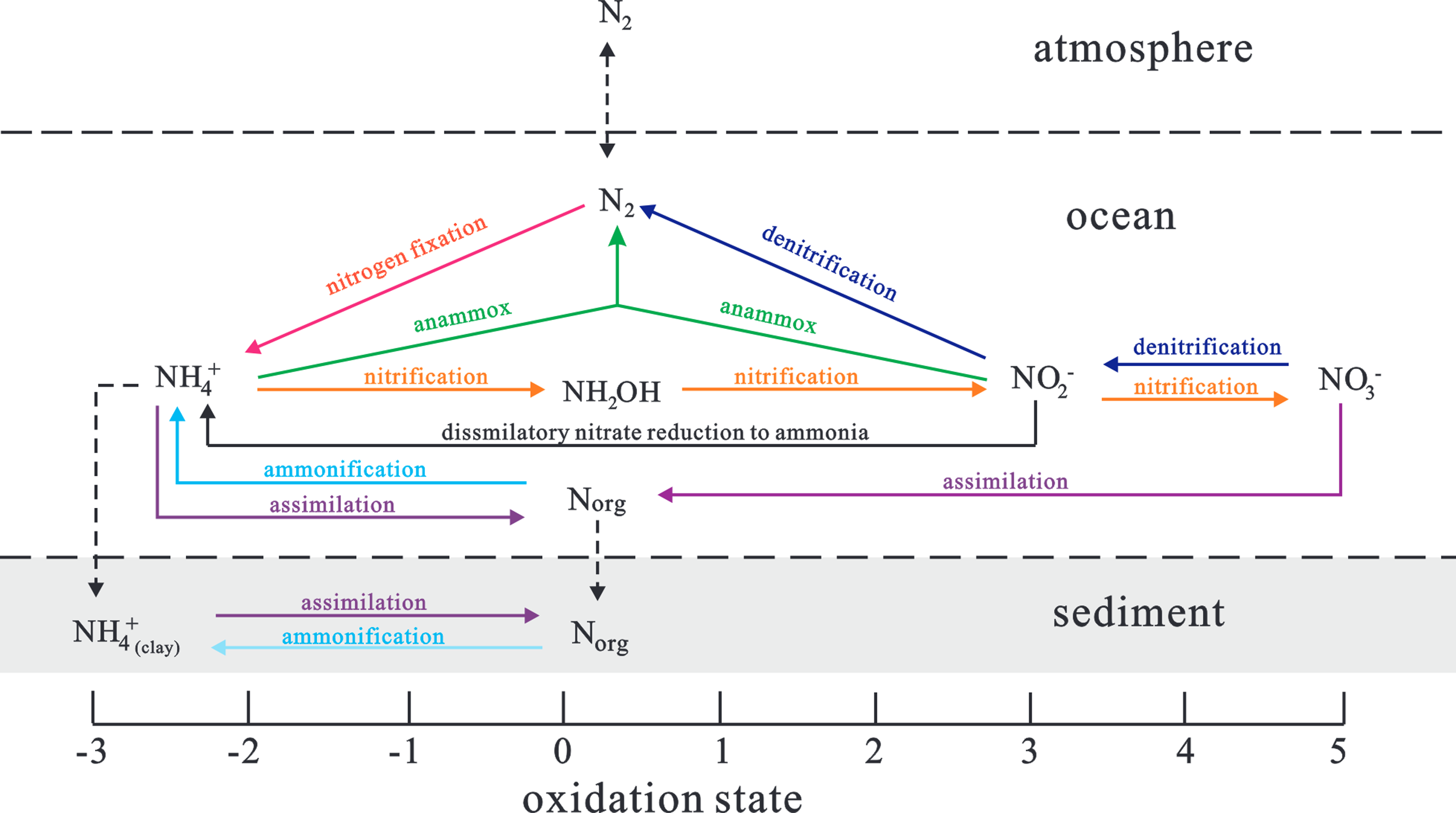
Figure 5. Application of the modern nitrogen cycle to the early Cambrian marine environment. For an analysis of dominant pathways in the lower Cambrian, see the main text. Modified from Godfrey and Falkowski (Reference Godfrey and Falkowski2009).
The removal of fixed nitrogen from seawater is mainly determined by denitrification and anaerobic ammonium oxidation (anammox), which are both active in suboxic environments and appear today primarily in oxygen minimum zones (OMZs) and oxygen-depleted pore waters (Sigman et al. Reference Sigman, Karsh, Casciotti, Steele, Thorpe and Turekian2009; Hong et al. Reference Hong, Wu, Guan, Yue and Long2019). Denitrification can normally convert NO3 − by anaerobic bacteria to N2 and lead to a residual nitrate reservoir enriched in heavier 15N with fractionation values of −20 ∼ −30‰ (Brandes & Devol, Reference Brandes and Devol2002). The isotopic fractionation of anammox can also reach up to −25‰ (Prokopenko et al. Reference Prokopenko, Hammond, Berelson, Bernhard, Stott and Douglas2006). Moreover, it was experimentally demonstrated that individual steps in nitrification can also amplify the fractionations in oxic water columns; however, net isotopic fractionation is normally not expressed via this pathway because nitrification is usually quantitatively associated with its fast rate of reaction (Lipschultz et al. Reference Lipschultz, Wofsy, Ward, Codispoti, Friedrich and Elkins1990). Thus, the primary δ15N composition is finally determined by the equilibrium between nitrogen fixation and denitrification/anammox, and this parameter also includes reliable redox clues.
Given the buildup of δ13Ccarb in the stratigraphic correlation framework, the BACE, ZHUCE and SHICE events have been recognized in the study area, where the BACE is coupled to a significant negative δ15N excursion (down to +0.3‰, Fig. 2). These values are distinctly lower than those from the modern ocean nitrate pool of ∼+5‰ (Sigman & Casciotti, Reference Sigman and Casciotti2001) and thus are suggested to be a strengthened nitrogen fixation signal. Environmentally controlled experiments have shown that only when seawater nitrate is consumed quantitatively at the chemocline can diazotrophy balance the nutrient structure of seawater by increasing nitrogen fixation (Sigman et al. Reference Sigman, Karsh, Casciotti, Steele, Thorpe and Turekian2009). Given that denitrification and anammox are anaerobic nitrogen cycling processes, the 15N-depleted isotopic signal associated with a slightly increasing TOC trend (up to 2.5%) might be independently explained by expanded marine anoxia in the earliest Cambrian Yangtze Sea. This scenario resembles the nitrogen isotope signal preserved in the Cretaceous Oceanic Anoxic Event (Higgins et al. Reference Higgins, Robinson, Husson and Pearson2012) and Late Devonian Hangenberg Event (Liu et al. Reference Liu, Qie, Algeo, Yao, Huang and Luo2016). Analogously, the SHICE across the boundary of the Yanjiahe and Shuijingtuo formations is marked by decreasing trends of δ13Ccarb and δ13Corg values accompanied by relatively long-term low δ15N values at approximately −1‰. We propose that the lighter δ15N values in the SHICE would result from an insufficient nitrate pool and intensified atmospheric nitrogen fixation, as well as partial ammonium assimilation into biomass (e.g., Liu et al. Reference Liu, Kao, Chiang, Gong, Chang, Cheng and Lan2013; Liu et al. Reference Liu, Magnall, Gleeson, Bowyer, Poulton and Zhang2020).
The ZHUCE in this study coincides with a rapidly increasing δ15N trend (up to 4.0‰), which is closer to the mean nitrogen isotope value of nitrate in modern open-ocean environments, implying that the nitrogen cycle experienced a key fluctuation during deposition of the lowermost Yanjiahe Formation. Based on the differences in net fractionation between sedimentary denitrification (∼−1 to −3‰) and water-column denitrification, reservoir box modelling emphasized that secular variation in the δ15N of sediments was dependent on the dominant locus of denitrification (Algeo et al. Reference Algeo, Meyers, Robinson, Rowe and Jiang2014). However, there is still controversy over the interpretation of paleoclimate and paleoenvironmental models for different nitrogen isotope distributions and their corresponding dominant locus of denitrification (Junium & Arthur, Reference Junium and Arthur2007; Liu et al. Reference Liu, Altabet and Herbert2008), which may be related to differences in timescales and/or restriction of marine basins (Algeo et al. Reference Algeo, Meyers, Robinson, Rowe and Jiang2014). Accepting that nitrogen fixation and denitrification are considered two main end members that affect the nitrogen isotope pattern of nitrate, we propose that the ∼4‰ positive excursion in δ15N was likely a consequence of declined rates of denitrification and a deepening chemocline, thereby amplifying the oxic water mass associated with an elevated nitrate pool. However, the δ15N value increased to only 4.0‰, which is still slightly below the levels found in modern oceans, implying that the nitrate pool of surface water in the ZHUCE was lower than the current level.
5.d. Implication for early Cambrian biotic evolution
Several carbon isotope anomalies closely related to the evolution of early Cambrian life have been identified, implying temporal coupling between the prosperity/extinction of organisms and marine environmental changes (Zhu et al. Reference Zhu, Zhang and Yang2007; Betts et al. Reference Betts, Paterson, Jacquet, Andrew, Hall, Jago, Jagodzinski, Preiss, Crowley and Brougham2018; George et al. Reference George, Kumar and Ray2021; Topper et al. Reference Topper, Betts, Dorjnamjaa, Li, Li, Altanshagai, Enkhbaatar and Skovsted2022). For example, the diversification of Ediacaran fauna can be correlated to the Dengying Positive Carbon Isotope Excursion (DEPCE, negative δ13C values); the extinction of the Ediacaran fauna near the E-C boundary on a global scale is synchronous with the BACE; the rapid radiation of the SSF assemblages in Cambrian Stage 2 can be well correlated with the ZHUCE; the extinction of the SSFs in Cambrian Stage 2 corresponds to the SHICE and the initial explosion of arthropods (especially trilobites) corresponds to the Cambrian Arthropod Radiation Isotope Excursion (CARE, positive δ13C values) (Fig. 6).
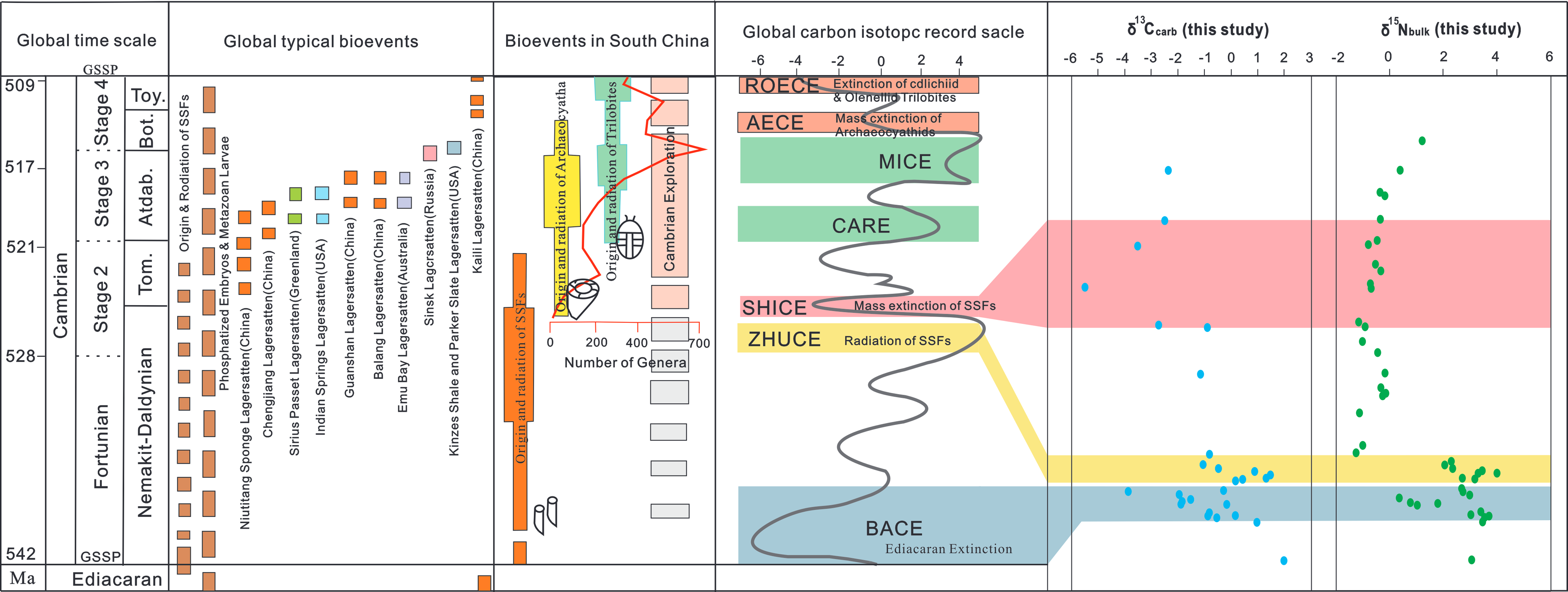
Figure 6. Compilation of evolutionary events with δ13Ccarb values during the early Cambrian (modified from Ren et al. Reference Ren, Zhong, Gao, Li, Cao, Wang, Dong and Yan2019). The diversity data of animal genera are from Li et al. (Reference Li, Steiner, Zhu, Yang, Wang and Erdtmann2007) and the δ13Ccarb curves are from Zhu et al. (Reference Zhu, Babcock and Peng2006).
The remarkable increase in dissolved oxygen levels in oceans was thought to serve as a pivotal catalyst that resulted in the innovation of primitive simple animals and their diversification (e.g., Erwin et al. Reference Erwin, Laflamme, Tweedt, Sperling, Pisani and Peterson2011; Sperling et al. Reference Sperling, Frieder, Raman, Girguis, Levin and Knoll2013). Indeed, the two negative δ15N excursions in this study occur synchronously in the BACE and SHICE, indicating the impact of the expansion of anoxic water masses on the living environment of the Ediacaran fauna and small shelly fauna. The BACE has a wide global response (Maloof et al. Reference Maloof, Ramezani, Bowring, Fike, Porter and Mazouad2010; Zhu et al. Reference Zhu, Yang, Yuan, Li, Zhang, Zhao, Ahn and Miao2019), and its initiation is coincident with sea level rise (Ishikawa et al. Reference Ishikawa, Ueno, Shu, Li, Han, Guo, Yoshida, Maruyama and Komiya2014). Iron speciation information provided evidence of anoxic and ferruginous environments in the Lower and Upper Yangtze areas during the E-C transition, such as the Diben section of Zhejiang, Silikou section of northern Guangxi and Longbizui and Yanwutan sections of western Hunan (Chang et al. Reference Chang, Chu, Feng and Huang2010; Wang et al. Reference Wang, Chen, Yan, Wei and Xiang2012; Yuan et al. Reference Yuan, Cai, Wang, Xiang, Jia and Chen2014). Given that the Rodinia breakup and Gondwana amalgamation occurred during the E-C transition, ocean circulation induced by plate movement might have led to enhanced upwelling on continental margins (Tucker, Reference Tucker1992; Wille et al. Reference Wille, Nägler, Lehmann, Schröder and Kramers2008; Ader et al. Reference Ader, Sansjofre, Halverson, Busigny, Trindade, Kunzmann and Nogueira2014; Wang et al. Reference Wang, Struck, Ling, Guo, Shields-Zhou, Zhu and Yao2015). In this case, deep-water upwelling would have enhanced nutrient fluxes and transported 13C-depleted anoxic water masses to the shallow platform area. In the ZHUCE, the δ15N value (with a maximum of 4‰) is slightly smaller than that of the modern ocean, indicating that the degree of oxidation was highly associated with the deepening chemocline, which provided a favorable O2 demand for the radiation of the small shelly fauna. The δ15N returned to 0∼−2‰ in the SHICE, which is indicative of the shallower chemocline relative to that in the BACE. The lower δ15N value in the SHICE is consistent with the largest global marine transgression during the Phanerozoic associated with strong upwelling (Goldberg et al. Reference Goldberg, Strauss, Guo and Liu2007), which would have transported the reducing deep water to the shallow-water area, thereby leading to the temporal extinction of small shelly fauna.
On the other hand, changes in the bioavailable nitrogen pool may have exerted an important influence on the regulation of eukaryotic organisms because oceanic nitrate is the preferential source of nitrogen for eukaryotic organisms (Karl et al. Reference Karl, Bidigare and Letelier2001). The variability in the oceanic nitrate pool mainly depends on the aerobic and anaerobic nitrogen cycling paths (Lehmann et al. Reference Lehmann, Bernasconi, Barbieri and McKenzie2002). Increased fixed nitrogen supply and episodic animal radiation have been proposed based on the coupling relationship between long-term δ15N variations and major diversifications in the metazoan group, as documented by body fossil and biomarker records (Wang et al. Reference Wang, Ling, Struck, Zhu, Zhu, He, Yang, Gamper and Shields2018; Wu et al. Reference Wu, Tian, Jia, Li, Li, Zhou and Xie2022). Contraction of the oceanic nitrate pool was associated with an anaerobic N cycle when the ocean became more anoxic with a shallower chemocline in the BACE and SHICE. Such nitrate limitation could have been linked to lower primary productivity and delayed radiation of eukaryotic organisms (e.g., Anbar & Knoll, Reference Anbar and Knoll2002; Koehler et al. Reference Koehler, Stüeken, Kipp, Buick and Knoll2017). The aerobic N cycle and more abundant nitrate available in the surface water are shown in the ZHUCE, which could have been beneficial to the evolution from single-celled microorganisms toward a eukaryote-dominated community (e.g., Stüeken, Reference Stüeken2013; Wang et al. Reference Wang, Ling, Struck, Zhu, Zhu, He, Yang, Gamper and Shields2018).
6. Conclusions
Time-series carbon and nitrogen isotopic data obtained from the YD-4 core samples across the E-C transition in the Yangtze Block present a sequence of significant disturbances, offering valuable environmental insights for the evolution of eukaryotes in the middle Yangtze Block. According to the comparison of the δ13Ccarb curves from several representative sections in the Yangtze Block and other time-equivalent strata in other larger regions, the BACE, ZHUCE and SHICE are well constrained in the study area. Within the BACE and SHICE in the transitional facies in the middle Yangtze Block, the δ15N value displays a rapidly negative excursion (∼0‰), suggesting enhanced microbial nitrogen fixation and partial ammonium assimilation, which were likely linked to complete denitrification associated with expanded anoxic conditions. In contrast, the positive δ15N values in the ZHUCE indicate a growing nitrate pool with a deeper chemocline. Given the linkage between the carbon isotope anomaly events and the δ15N patterns, the widespread anoxic water conditions may have been related to the extinction of Cambrian-type animals, while an expansion of oxic waters and an amplified nitrate pool in the surface waters may have promoted Cambrian biodiversity.
Acknowledgments
We are grateful for support from the China Geological Survey (DD20230006), the Natural Science Foundation of Hubei Province (2023AFB1103), Guangdong Natural Science Foundation of China (2022A1515012100), the Foreign Aid Project of the Ministry of Commerce of the People’s Republic of China ((2021) 28), South China Sea Scholar Project of Guangdong Ocean University (002029002007), Guangdong Provincial College Innovation Team Project (2019KCXTF021) and First-class Discipline Plan of Guangdong Province (080503032101, 231420003).