INTRODUCTION
In Romania, two CANDU 600 (CANada Deuterium Uranium) reactors are currently in operation at the Cernavoda site, and two more CANDU units are foreseen to be commenced in the future. Due to its long half-life (T1/2=5730 yr), high mobility in groundwater system, easy incorporation into humans via the food-chain, and high inventory in radioactive waste generated from Cernavoda Nuclear Power Plant (NPP) operation, 14C is a radionuclide of concern for radioactive waste management, both in the predisposal activities as well as for final disposal.
14C is generated mainly by neutron activation of stable nitrogen—14N, oxygen—17O and, to a lesser extent, stable carbon isotope—13C. In nuclear reactors, 14C is produced in the fuel, from core structural materials, and in reactor coolant, due to the presence of the stable parent isotopes that are activated by the neutrons generated from nuclear fission reactions occurring in the fuel (Yim and Caron Reference Yim and Caron2006).
Since CANDU reactors use heavy water (D2O) both as moderator and as coolant, more 14C is generated in this type of reactors due to the large amount of D2O having higher isotopic abundance in 17O compared to the light water (0.055% in D2O and 0.037% in H2O), but also to the higher average thermal neutron flux in CANDU reactors (1014 ncm–2s–1compared to 1013 ncm2 s–1 in light water reactors).
From the total 14C generated in a CANDU reactor, more than 95% is generated in moderator system (MOD), around 1.3% in the primary heat transport system (PHTS), 3.37% in the fuel, and 0.13% in the annulus gas (IAEA 2002). Most of the 14C generated in MOD and PHTS is retained in the ion exchange resins (IERs) used to purify the heavy water from these circuits. When the criteria thresholds are reached in a circuit, spent ion exchange resins (SIERs) are discharged in form of slurry (under a flow of light water) and transferred to the storage vaults designed to store the SIERs generated during the life of Cernavoda NPP. At Cernavoda NPP, the SIERs are stored in different concrete vaults depending on their origin: SIERs originating from non-fuel contact systems (systems that are not coming in contact with the fuel, i.e. MOD, liquid zone control, etc.) are stored separately to the SIERs generated in fuel contact systems (i.e. PHTS, spent fuel cooling pool, etc.).
The chemical form under which 14C is fixed within or adsorbed to the IERs is highly related to the chemical condition of the reactor. The heavy water in CANDU MOD system has low temperature and pressure (~60°C and essentially atmospheric pressure) and it is highly purified; the only chemicals added to the system are those used as neutron poisons for excess reactivity control or to guarantee reactor shut-down (typically, gadolinium nitrate and/or boric acid). The pH of the heavy water in MOD is kept slightly acidic and it is not purposely deaerated; if necessary oxygen may be added to ensure the recombination of deuterium generated by radiolysis (Cook and Lister Reference Cook and Lister2014). Due to the above described chemical conditions prevailing in the MOD system of the CANDU reactor, the 14C, generated mainly by neutron activation of 17O, would be predominantly as 14CO2 and carbonate species dissolved in D2O (IAEA 2002). These species are easily fixed on the IERs (around 91.43 % of the 14C generated in MOD is retained on the IERs) and only a limited amount of 14C is released to the atmosphere through the ventilation system of the moderator cover gas system (IAEA 2002). Consequently, the main radioactive waste stream containing 14C consists of SIERs generated in MOD purification system. Even for the MOD SIERs, it is recognized that the 14C content is highly dependent on the column operating history. For example, the resins used for normal moderator purification usually contain high levels of 14C, while the resins used for moderator reactivity control contain low levels of 14C.
Unlike the MOD, the heavy water in the PHTS system has an alkaline pH (~10.5) and the water is deaerated to maintain the dissolved oxygen concentrations below 0.01 mg/kg (Cook and Lister Reference Cook and Lister2014). Under these reducing conditions, in the PHTS less oxidized 14C species could be generated, i.e. end-product 14CH4. But as mentioned above, in PHTS only a small fraction of 14C is generated.
The different release pathways for 14C in gaseous or dissolved forms and the lack of knowledge of its speciation in both phases results in high uncertainty in its potential contribution to the total dose that could be associated with a radionuclide release from a radioactive waste disposal facility. The travel time of 14C from such a facility to the biosphere is affected by both the physical form of 14C (e.g. due to the potential migration of gaseous 14C with bulk gas) and the chemical speciation (organic and inorganic 14C species have different sorption in the geosphere). For these reasons, not only the total 14C inventory in SIERs but also its speciation are important for a safety assessment of disposal facility containing SIERs.
The information on 14C content and speciation in CANDU SIERs is scarce, and available data are those from the measurements carried out on SIERs generated from CANDU reactors in operation in Canada and South Korea. Based on available data for CANDU reactors from Canada, the specific activity of 14C in the SIERs originating from the MOD is ranging between 0.05 and 213 Ci/m3, with an average value of 71 Ci/m3, while in the SIERs from PHTS the 14C specific activity is between 0.24 and 6.7 Ci/m3, with an average of 3.34 Ci/m3 (Miller et al. Reference Miller, Erdebil and Solaimani1997). Results obtained by Park et al. (Reference Park, Kim, Han and Jee2008) on SIERs from CANDU reactors from Korea, and by Moir et al. (Reference Moir, Ross, Chen, Tarr, Bialas, Boivin, Doern, Hoffman and Ross1994) on two moderator SIERs from the CANDU reactor Bruce A in Canada, show that more than 92% of the 14C activity in SIERs is retained in anionic resins under inorganic form (Park et al. Reference Park, Kim, Han and Jee2008). A minor fraction of 14C containing species has been measured in the cation exchange resins, after their separation from a mixed bed (Moir et al. Reference Moir, Ross, Chen, Tarr, Bialas, Boivin, Doern, Hoffman and Ross1994; Park et al. Reference Park, Kim, Han and Jee2008), probably under the form of metal carbonate (Moir et al. Reference Moir, Ross, Chen, Tarr, Bialas, Boivin, Doern, Hoffman and Ross1994).
Within the CArbon-14 Source Term (CAST) project (Scourse and Williams Reference Scourse and Williams2014), Work Package 4 was particularly dedicated to the identification of the source term of 14C within SIERs and to the speciation of the possible released 14C bearing molecules. In this paper, experimental data for inorganic and organic 14C content in a SIERs sample from Cernavoda NPP are reported. Also, to get an insight on potential 14C form and species (inorganic and organic) released from SIERs in cementitious environment characteristic to the SIERs disposal, results on 14C desorption in alkaline conditions are also presented.
MATERIALS AND METHODS
Currently, at Cernavoda NPP the SIERs are stored in three reinforced concrete vaults, lined with epoxy resin, with capacity of 200 m3 each. The SIERs are segregated as fuel contact and non-fuel contact resins and stored in separated vaults. No SIER samplings from the Cernavoda storage vaults were performed and no real measurements on Cernavoda SIERs were performed before the beginning of the CAST project. During maintenance, beads of spent resins were collected from the strainers of the purification systems and stored in drums in Cernavoda storage facility. Such a SIER sample (in form resin beads in heavy water) originating from purification systems of MOD and of PHTS from Unit 1 of Cernavoda NPP was transferred to RATEN ICN laboratory in 2015 to be used in the experimental programme developed under CAST project. To decrease the tritium content and to get a D2O content less than 10%, a volume of distilled water was added over the 100 g of SIERs and the container was put in a gas-tight vessel and transferred to RATEN ICN.
Method Used for Total 14C Measurement
For total 14C measurement in SIERs, a non-catalytic combustion by the flame oxidation method was used. By this method, the resin samples are combusted in an oxygen-enriched atmosphere with a continuous flow of oxygen using sample oxidizer Model 307 by PerkinElmer. By sample combustion in this system any hydrogen and carbon is oxidized to H2O and CO2, respectively. The H2O resulting from the combustion process is condensed in a cooled coil, washed into a counting vial where it is mixed with LSC cocktail (MonophaseS) and the resulting vial is ready for tritium counting by liquid scintillation counting (LSC).
Since the carbon dioxide readily reacts with compounds containing amines (RNH2, with R representing the organic radical, an organic substituent known as “side chain”), the Carbo-Sorb® E was chosen to absorb the CO2 released during combustion process. The adsorption reaction of carbon dioxide can be described as follows (Ahn et al. Reference Ahn, Song, Sohn, Lee, Song and Jee2013):



As shown in the above equations, the amine contained by the Carbo-Sorb E® solution (RNH2) reacts with 14C-labeled carbon dioxide to form a zwitterion, which reacts with H2O to form a stable carbonate compound (Equation 3) that is mixed with Perma-fluor® E+ directly in the counting vial to be counted by LSC for 14C measurement.
At the end of the combustion process, two separate samples, one for 14C measurement and one for 3H measurement, are trapped at ambient temperature minimizing the cross-contamination. 14C and 3H measurements were achieved using a Tri-Carb® analyzer Model 3110 TR. The major problem encountered in LSC measurements is chemiluminescence and photoluminescence (phenomena characteristic of an excitation of the cocktail either during sample preparation or storage). The standard method of avoiding this problem is to use a cocktail which is known to be resistant to chemiluminescence as well as to allow the chemiluminescence to decay in the dark before counting. For these reasons, even the LSC cocktails used are resistant to chemiluminescence, all samples were kept few hours in dark before counting.
Method Used for 14C Speciation Measurement
As 14C is a pure β-emitter (with a fairly low energy compared with the β energy emissions of the other radionuclides) it belongs to the category of difficult-to-measure radionuclides. For 14C measurement by LSC, the 14C has to be separated and purified by other potential interfering radionuclides (such as 3H, 129I, 137Cs etc.) to get accurate results.
The analytical method used for inorganic and organic 14C measurement in SIERs samples consists of a sequential extraction of inorganic and organic 14C using acid stripping and wet oxidation, adapted after the method developed by Magnusson et al. (Reference Magnusson, Stenström and Aronsson2008) for 14C measurement in spent ion exchange resins and process waters.
The experimental setup used for release and separation of inorganic and organic 14C is schematically presented in Figure 1. It consists of a reaction vessel, a separatory funnel, a nitrogen supply and a vacuum pump, two gas washing lines with a catalytic furnace between them. An Erlenmayer flask (300 mL) with a three-hole rubber stopper (two for gas and separatory funnel inlets and one for gas outlet) was used as reaction vessel and a tap-water cooling loop, made of copper tubing that fits the outer side of the Erlenmeyer flask ensured the vapor condensation. The reaction vessel was placed on a heater with magnetic stirring.

Figure 1 Experimental setup for separation of inorganic and organic 14C.
To ensure that no gases escape from the system, all dissolution and wet oxidation experiments were carried out at 0.2 bar below atmospheric pressure, and the carrier gas (N2) was introduced into the system with a flow rate between 60 and 80 mL/min (controlled by a flow meter).
Since SIERs could contain, besides 14C, also tritium, iodine, and other beta emitters that interfere with 14C measurement by LSC, the gas washing lines consist of iodine traps, placed both before and after the catalytic furnace, with silver nitrate (AgNO3), slightly acidic trap with sulphuric acid (5% H2SO4) for tritium and other potential interfering radionuclides and four alkaline traps (two placed before the catalytic furnace and two after it) with 2M sodium hydroxide (NaOH).
The possible inorganic 14C species in equilibrium are described by the following equilibria (Guillaumont et al. Reference Guillaumont, Fanghänel, Fuger, Grenthe, Neck, Palmer and Rand2003):



Carbonates CO3 2–, bicarbonates HCO3 –, and dissolved CO2(aq) equilibria can be easily shifted by weak acids towards gaseous carbon dioxide. The inorganic 14C is released during an acid stripping step resulting mainly in the release of 14CO2 and the gases are carried by the carrier gas through the first gas washing line (Figure 1). If any 14C is released as 14CO or other organic molecules, the flow passes through the scrubbing bottles of the first gas washing line, and further through the catalytic furnace, where 14CO and other 14C-bearing organic molecules are oxidized to 14CO2, and are subsequently absorbed in the scrubbing bottles of the second gas washing line. After the acid stripping step is accomplished, the first gas washing line is isolated from the system by means of three-way valves placed before the first scrubbing bottle and the fourth one.
Since organic compounds have higher bounding energies between atoms, they are decomposed by strong oxidants such as potassium persulphate (K2S2O8). The presence of a catalyzer such as silver nitrate (AgNO3) enhances the decomposition of the organic compounds and also the iodine precipitation as AgI. During the wet oxidation step the temperature of the solution in the reaction vessel is slightly increased to 90°C.
The mechanism of 14C-labeled organic compounds decomposition is based on the OH(*) radicals and can be expressed by the following equations (Ahn et al. Reference Ahn, Song, Sohn, Lee, Song and Jee2013):



The 14C released during wet oxidation step of the analytical procedure (both as CO2, but also as CO or CH4) is carried by the carrier gas through a catalytic furnace where it is oxidized to CO2, which is afterwards absorbed in the scrubbing bottles of the second gas washing line (Figure 1).
The 14C activity in the alkaline traps, as well as the3H activity in solutions sampled from the acid scrubbing bottles, was measured by LSC. Hionic Fluor liquid scintillation cocktail was used for 14C measurement by LCS, and Ultima Gold AB liquid scintillation cocktail for tritium measurement. The ratio between sample and scintillation cocktail was 1:10. All samples were kept in darkness overnight before their counting to allow for the chemiluminescence decay.
Aliquots from the reaction vessel solution, as well as from all scrubbing bottles were sampled for gamma measurements using an HPGe ORTEC detector and digiDart analyzer for spectra acquisition and Gamma Vision software for radionuclides quantification. The spectrometer was calibrated in energy and efficiency using a standard liquid source containing 60Co, 137Cs, 241Am, 152Eu in 20 mL glass vials and the same geometry was used for all gamma measurements. Depending on the radioactivity content, each sample was counted for more than 4 hours.
14C Desorption in Alkaline Conditions
Investigations of the SIERs’ behavior in alkaline solution (NaOH 0.1 M, with pH ~ 13), as a proxy to cementitious conditions, were carried out by means of desorption tests on two subsamples from Cernavoda SIERs. Two parallel tests (Test #1 and Test #2) were performed with 0.4 g of SIERs in contact with 40 mL of NaOH 0.1 M (liquid to solid ratio of 0.01 g.mL–1), in borosilicate glass bottles with polyketone (PK) lids adapted to allow N2 introduction in the desorption vessels and outgas washing through the two gas washing lines of the experimental setup presented in Figure 1. To assess the inorganic and organic 14C release in gas phase during desorption tests, scrubbing bottles with smaller volume were used as alkaline traps: two with 3 mL of NaOH 2M (B3 and B7) and two with 15 mL NaOH 2M (B4 and B8).
Desorption tests were performed at room temperature (23 ± 3°C) in semi-dynamic conditions: 5 mL of desorption solution was sampled at each time step, and 5 mL of fresh NaOH was added. Desorption solution was sampled daily in the first 4 days, and after 9, 17, 24, 45, 95, 122, and 174 days of desorption. At the end of the desorption tests, not only 14C activity in liquid and gas phase was measured but also the residual 14C activity in the SIER sample subject to desorption test.
Before sampling desorption solution, at each sampling time, N2 gas was purged in the space above the liquid level and outgases were washed through the gas bubblers. Since at the beginning of the desorption tests it was not clear whether the 14C released in gas phase could be measured by washing outgases through alkaline bubblers, the nitrogen gas was introduced through both parallel desorption vessels in the same time. To get the amount of gaseous 14C released from one vessel, the amount measured in the alkaline solution from the gas bubblers was divided by two assuming the 14C released in gas phase was equal from the two parallel tests.
The inorganic and organic 14C measurements in desorption solutions sampled during the tests were achieved using a similar procedure as that described for 14C speciation measurement in SIERs.
RESULTS AND DISCUSSION
Total 14C Measurements
To optimize the combustion method described above for total 14C measurements, preliminary tests were carried out in controlled conditions using samples of ion exchange resin (IRN 150, similar with the mixed bed resin used in the purification circuits of Cernavoda NPP) labeled with the radionuclides of interest: 14C (inorganic form as sodium carbonate/bicarbonate, and organic form as sodium acetate), 3H, 60Co, 137Cs, 241Am, and 152Eu. By these tests, recovery yields, reproducibility of the results, and memory effect of the methods were evaluated. After these preliminary combustion tests, it was concluded that the complete resin combustion is achieved within 3 minutes for 0.15 g of resin mixed with 0.5 mL of combustade (an agent that enhance the combustion process).
The 14C recovery obtained in these combustion tests was ranging between 93.8% and 99.9%, with an average of 97.5% and standard deviation of 1.24, proving the good reproducibility of the results. The memory effect was less than 0.04% and no gamma emitters were identified either in the counting vial for 14C measurement or in the 3H one.
Before analyzing the spent resins by combustion, a mass of SIERs sample received from Cernavoda was filtered to remove the excess of water. After filtration, the water content of the resin sample was 60.10% (calculated based on mass loss at 105°C, using a thermo balance). From this sample, 5 subsamples of around 0.15 g were prepared for total 14C measurement by combustion and other 5 subsamples were used for inorganic and organic 14C measurements by acid stripping/wet oxidation method. The values obtained for total 14C specific activity in the analyzed SIERs samples are reported in Table 1. After combustion, no other beta-gamma emitters were identified in the 14C vials.
Table 1 The total 14C content in Cernavoda SIERs.

* Wet mass.
The experimental results obtained for these samples indicate that the SIERs sample transferred from Cernavoda NPP has a 14C content of 36.5kBq/g, corresponding to around 7 Ci/m3 (assuming a bulk density of 0.71 g/mL for wet resin). This activity is lower than the reported values for MOD SIERs and more closed to the values reported for PHTS resins (Miller et al. Reference Miller, Erdebil and Solaimani1997), but as it was mentioned earlier the available SIERs sample is a mixture of resin beads collected during maintenance activities and it is not representative for the SIERs generated in MOD or PHTS. The total 14C was measured to confirm the recovery of the method used for 14C speciation measurements rather than to have representative total 14C content in SIERs generated at Cernavoda NPP.
14C Speciation Measurements
To optimize the experimental conditions and chemicals used for inorganic and organic 14C measurements, a series of acid stripping/wet oxidation tests were carried out in controlled conditions, using liquid solutions with known activities for the radionuclides of interest (14C in inorganic form as sodium carbonate/bicarbonate and organic forms as sodium acetate and lauric acid, 3H, 129I, 60Co, 137Cs, 241Am and 152Eu) as well as ion exchange resin labeled with the same radionuclides.
By these tests the 14C recovery, the reproducibility of the results and the efficiency of the acid stripping/wet oxidation method to distinguish between inorganic and organic 14C species were evaluated. The results show that by this analytical method good recovery and efficient purification are obtained:
∙ inorganic 14C recovery: between 95% and 99%, with an average value of 97% and standard deviation of 1.46;
∙ organic 14C recovery: between 94% and 98%, with an average value of 96% and standard deviation of 1.55;
∙ no other emitters were identified in the solutions sampled from alkaline gas washing bottles.
An average memory effect of less than 1% was observed (no washing step was performed between the recovery step and the memory one).
To account for the uncertainty associated to this analytical method, six identical tests were carried out using ion exchange resin (IRN 150) labeled with the above-mentioned radionuclides. The standard deviation of the results of these tests was less than 15%, proving the good reproducibility of the results. The experimental conditions chosen for the inorganic and organic 14C measurement in CANDU SIERs are presented in Table 2.
Table 2 Parameters for inorganic and organic 14C measurement in CANDU SIERs.

From the same filtered SIERs prepared for total 14C measurement, five subsamples of 1 g each were used to measure the inorganic and organic 14C content in experimental conditions summarized in Table 2. Both filtered spent resin samples and the excess water were analyzed. The experimental results obtained for inorganic and organic 14C are presented in Table 3.
Table 3 Inorganic and organic 14C content in Cernavoda SIERs.

The total 14C content in the analyzed SIERs samples are quite close to the value determined by combustion, demonstrating the good recovery of the acid stripping/wet oxidation method. The analysis of the excess water shows that it contains no inorganic 14C and only 4.42 Bq/mL of organic 14C (representing only 0.01% from the 14C on the spent resin).
Regarding the partition between mineral and organic species, the results obtained show that the 14C in the analyzed SIERs is predominantly in inorganic form and only less than 7% from the measured 14C is present in organic form. Since the main source of the analyzed SIERs is the moderator purification system, and in the CANDU moderator the major chemical form of 14C is as bicarbonate, the chemical form of 14C absorbed on the resin is also mostly bicarbonate (H14CO3 –).
Even though these results were not obtained on a representative SIER sample for the spent resins that will be disposed of (either in a surface disposal facility or in a geological one), data obtained are similar to those reported by Park et. al. (2008), who found that more than 92% of the 14C activity concentration is as inorganic 14C.
14C release under Alkaline Conditions
Desorption experiments with Cernavoda SIERs demonstrated that the highest fraction of 14C is released as dissolved species, presumably as carbonate. Equilibrium in the solution is reached within the first 2 days (Figure 2), whereas the equilibrium level of inorganic 14C released into the gas phase was instantly achieved and did not change until the end of experiment (ca. 200 days).

Figure 2 The kinetic of 14C released into the solution (left) and gas phase (right).
The experimental data obtained from the desorption tests for the 14C activity, expressed as total 14C and inorganic and organic partition, measured at each sampling time in solution and gas, are reported in Table 4. As can be seen, the 14C measured in desorption solution is mainly in inorganic form and only insignificant fraction was found to be in organic forms, at least for desorption times less than 24 days. After this time, the organic 14C measured in desorption solutions represents around 8% from the total 14C.
Table 4 The row data measured in desorption tests.

Based on these data, cumulative 14C release, as well as the release rate, were calculated and are presented in Figure 3.
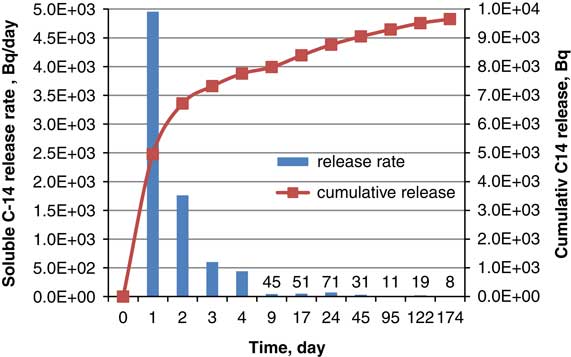
Figure 3 Differential and cumulative release of 14C in liquid phase.
From the total 14C present in the SIERs sample used for desorption test, around 7% was released as inorganic 14C in the gas phase, around 79% as dissolved species and 8% was found as residual in the SIERs sample after desorption. Around 94% of 14C content in the SIER sample was recovered at the end of desorption test. The remaining ca. 6% was not found either as dissolved species or inorganic gas species, or as residual on the SIER sample after desorption test, and could represent an unaccounted for 14C fraction of instantly released 14CO2. No organic 14C released in gas phase was measured during these tests. Gaseous releases from SIERs in cementitious conditions are caused by a transient/permanent decrease in the pH of the alkaline solution, most probably due to H+ ions released during the ion-exchange reaction of SIERs and alkaline solution (Bucur et. al. Reference Bucur, Rizzato and Shcherbina2017).
These results should nevertheless be further mitigated in view of the composition of actual cement pore water, which contains high concentration of dissolved calcium, and the possible precipitation of calcite (Berner Reference Berner1992; Pointeau et al. Reference Pointeau, Coreau and Reiller2008).
In the Cernavoda spent ion exchange resins, the major part of 14C is retained as inorganic form, in complete agreement with the literature data, and only less than 7% from the measured 14C on a SIERs sample is present inorganic form.
The total 14C content obtained by acid stripping/wet oxidation is quite close to the value determined by combustion, demonstrating the good recovery of the acid stripping/wet oxidation method. Moreover, the acid stripping/wet oxidation method allows measurement of other radionuclides in SIERs (i.e. tritium, iodine, and gamma emitters) and consequently could be used in addition to other separation techniques for a complete SIERs radiochemical characterization.
In alkaline conditions, 14C is released from SIERs both as gaseous and soluble species, predominantly as inorganic soluble species (~79%) and only a small fraction (~7%) as inorganic gaseous species. But in real conditions, SIERs will be conditioned in a suitable matrix (i.e. cement or polymer) and the percent of 14C released as soluble species shall be much lower. Furthermore, the presence of dissolved Ca in cement pore water will favor the 14C precipitation and consequently the decrease of dissolved 14C released from the disposal area.
ACKNOWLEDGMENTS
The results presented in this paper were achieved in the frame of CArbon-14 Source Term project funded by the European Union’s European Atomic Energy Community’s (EURATOM) Seventh Framework Programme FP7/2007-2013 under grant agreement no. 604779, the CAST project.