1. Introduction
The Central Mediterranean is a very complicated yet interesting geological puzzle. At the regional scale, the development of this basin and surrounding mountain chains is related to the interaction between the African and European plates since Late Cretaceous time (e.g. Biju-Duval et al. Reference Biju-Duval, Letouzey, Montadert, Courrier, Mugniot, Sancho, Burk and Drake1974; Laubscher & Bernoulli, Reference Laubscher, Bernoulli, Nairn, Kanes and Stehli1977; Vai & Martini, Reference Vai and Martini2001). Nonetheless, the single features are difficult to frame into a ‘simple’ collisional geodynamic context.
Scattered fragments of an ancient collisional chain that once extended from Gibraltar to the Alps have been recognized in the islands of Sardinia and Corsica (e.g. Alvarez, Reference Alvarez1976, Reference Alvarez1991), separated from the current French–Spanish coast in early Miocene time (Burdigalian–Langhian; Speranza et al. Reference Speranza, Villa, Sagnotti, Florindo, Cosentino, Cipollari and Mattei2002) by the opening of the Liguro-Provençal Basin. In particular, on the eastern coast of Sardinia (Golfo di Orosei area), remnants of a W-verging (in present-day coordinates) fold-and-thrust belt have been found (Arragoni et al. Reference Arragoni, Maggi, Cianfarra and Salvini2016), resulting from the deformation of the European sedimentary wedge and its basement in Cenozoic time. Despite their noteworthy geological significance, these small relics of the collisional belt cannot account for the entire European palaeo-sedimentary wedge; an important part of this palaeogeographic domain is apparently missing.
Furthermore, most collisional chains from around the world are characterized by double vergence, for example: the Rocky Mountains (Bally, Gordy & Stewart, Reference Bally, Gordy and Stewart1966; Fitz-Diaz, Hudleston & Tolson, Reference Fitz-Diaz, Hudleston, Tolson, Poblet and Lisle2011); the Alps (Schmid et al. Reference Schmid, Pfiffner, Froitzheim, Schönborn and Kissling1996; Lardeaux et al. Reference Lardeaux, Schwartz, Tricart, Paul, Guillot, Béthoux and Masson2006; Lammerer et al. Reference Lammerer, Gebrande, Lüschen, Veselá, Siegesmund, Fügenschuh and Froitzheim2008); and the Himalaya (Butler, Reference Butler1986; Acton et al. Reference Acton, Priestley, Mitra and Gaur2010). As evidenced by Arragoni et al. (Reference Arragoni, Maggi, Cianfarra and Salvini2016), the Eastern Sardinia fold-and-thrust belt shows a westwards vergence related to the westwards subduction (in present-day coordinates) of the African Plate beneath the European Plate, and the opposite verging side may be heavily hidden within the Apennines by the successive post-Oligocene Tyrrhenian Sea opening and Apennine chain formation.
The Eastern Sardinia mountain belt has been deeply eroded since Eocene time. The Mesozoic sedimentary cover was completely removed in many places, leaving the Palaeozoic basement exposed together with the deeper flat-over-flat sectors of the regional thrusts (sole thrusts). This erosional phase implies the production of a huge amount of sediments (both carbonate and silicoclastic) and raises some questions: which are the Cenozoic sedimentary units that record this event? Where are these units located now?
The opening of the Liguro–Provençal and Tyrrhenian Sea basins, which disrupted and translated the former Alpine collisional chain in Neogene time, determined the formation of the Apennines. Here we will use the term ‘Central-Southern Apennines’ to indicate the portion of the Apennines between the Ancona–Anzio line (Parotto & Praturlon, Reference Parotto and Praturlon1975; reinterpreted as structural element the Olevano–Antrodoco line by Salvini & Vittori, Reference Salvini and Vittori1982) and the Calabria–Peloritani block.
This oblique chain (with respect to the present-day orientation of the zone of convergence between Africa and Europe) derives from the deformation of the Meso-Cenozoic sedimentary units (mainly carbonates) detached from their basement (e.g. Vai & Martini, Reference Vai and Martini2001). According to the most accepted interpretations (e.g. Carmignani et al. Reference Carmignani, Decandia, Disperati, Fantozzi, Kligfield, Lazzarotto, Liotta, Meccheri, Vai and Martini2001 a; Barchi et al. Reference Barchi, Landuzzi, Minelli, Pialli, Vai and Martini2001; Molli, Reference Molli, Siegesmund, Fügenschuh and Froitzheim2008; Rossetti et al. Reference Rossetti, Glodny, Theye and Maggi2015) the Northern Apennines resulted from the deformation of the African margin; a sequence of palaeogeographic domains can be reconstructed, from the ancient continental passive margin represented by the Umbria–Marche and Tuscany successions, to the Tethys oceanic domain represented by the ophiolite-bearing Liguride units. These oceanic units are tectonically superposed on the whole African palaeo-passive margin units.
In the last decades, a number of high-quality studies have been performed in the Central-Southern Apennines with the development of several models (e.g. Scandone, Reference Scandone1967, Reference Scandone1975; Ogniben, Reference Ogniben1969, Reference Ogniben1972; D'Argenio, Pescatore & Scandone, Reference D'Argenio, Pescatore and Scandone1973; Pescatore & Ortolani, Reference Pescatore and Ortolani1973; Mostardini & Merlini, Reference Mostardini and Merlini1986; Sgrosso, Reference Sgrosso1986; Patacca et al. Reference Patacca, Sartori and Scandone1990; Vai & Martini, Reference Vai and Martini2001; Vezzani, Festa & Ghisetti, Reference Vezzani, Festa and Ghisetti2010). However, due to the complex geological evolution of this area, the geodynamic significance and attribution of deformed units is still debated and a complete sequence of palaeogeographic domains is more difficult to reconstruct. In this area the Liguride units occur both (mostly) under and over the Meso–Cenozoic sedimentary cover (e.g. Majella Mountains: SGN, 1970; Volsci Range and Latina Valley: Angelucci, Devoto & Farinacci, Reference Angelucci, Devoto and Farinacci1963; Angelucci & Devoto, Reference Angelucci and Devoto1966; SGN, 1967; Cilento: Monaco & Tortorici, Reference Monaco and Tortorici1995; Catalano et al. Reference Catalano, Monaco, Tortorici, Paltrinieri and Steel2004).
A crucial open question in the Central-Southern Apennines is the presence of widespread conglomeratic units containing igneous and metamorphic basement clasts whose origin and significance are still controversial. These include many different scattered sedimentary units cropping out from the Filettino area (NE of Rome, Latium) to the Cilento region (SE of Naples, Campania). The presence of basement clasts in these conglomerates is a striking feature already noted and discussed by the first researchers who studied the geology of the Central-Southern Apennines (e.g. Viola, Reference Viola1898; Sacco, Reference Sacco1910; Beneo, Reference Beneo1943). In the existing geological maps of the region they are usually reported as ‘puddinghe, talora con elementi di rocce intrusive (graniti, porfidi) ed altre rocce estranee alla regione’ (‘clast-supported conglomerates, sometimes with clasts of intrusive rocks – granites, porphyries – and other exotic rocks’; SGN, 1939), ‘puddinghe poligeniche’ (‘polygenic clast-supported conglomerates’; SGN, 1963), ‘Conglomerati a matrice prevalente, con elementi di rocce cristalline e calcaree’ (‘matrix-supported conglomerates, with clasts of crystalline and calcareous rocks’; SGN, 1969) or ‘Conglomerati stratificati, a ciottoli grossolani di natura prevalentemente estranea alla serie locale’ (‘well-bedded conglomerates, with coarse-grained clasts of prevailingly exotic origin’; SGN, 1975).
Despite the apparently local significance of these deposits, their correct interpretation could bear dramatic consequences for the geological interpretation of the whole Central Mediterranean area. As already discussed the sedimentary cover of the Central-Southern Apennines lacks its basement and outcrops of metamorphic or igneous lithologies are absent, except in the Calabro–Peloritani arc (Bonardi et al. Reference Bonardi, Cavazza, Perrone, Rossi, Vai and Martini2001) and in very small outcrops of basement rocks (e.g. the Zannone Island, De Rita et al. Reference De Rita, Funiciello, Pantosti, Salvini, Sposato and Velonà1986; Pantosti & Velonà, Reference Pantosti and Velonà1986) and metamorphosed limestones, probably related to hydrothermal alteration due to the presence of regional-scale faults (e.g. the Mondragone yellow marble, Di Girolamo et al. Reference Di Girolamo, Sgrosso, De Gennaro and Giurazzi2000). The source area for these exotic clasts therefore has to be searched for in places where the basement was extensively exposed at the time of deposition, and also subjected to the typical peneplanation processes active in the various geodynamic environments after the tectonic activity (Cianfarra & Salvini, Reference Cianfarra and Salvini2014; Maggi, Cianfarra & Salvini, Reference Maggi, Cianfarra and Salvini2016; Cianfarra & Maggi, Reference Cianfarra and Maggi2017). These include the Calabro–Peloritani arc and the Sardinia–Corsica block (Maggi et al. Reference Maggi, Rossetti, Corfu, Theye, Andersen and Faccenna2012, Reference Maggi, Rossetti, Ranalli and Theye2014; Rossetti et al. Reference Rossetti, Glodny, Theye and Maggi2015), which were physically connected to the Central-Southern Apennines before the opening of the Tyrrhenian Sea (Langhian: Kastens & Mascle, Reference Kastens and Mascle1990; Sartori et al. Reference Sartori, Torelli, Zitellini, Carrara, Magaldi and Mussoni2004).
The recognition of specific source areas for these conglomerates allows the geological evolution of the Central-Southern Apennines to be linked to that of Sardinia, hence providing new clues for the reconstruction of the Meso-Cenozoic European sedimentary wedge.
In this work we analyse the sedimentary features of four different conglomeratic units cropping out from Filettino to Cilento. The petrographic and geochemical features of igneous clasts sampled in selected outcrops are described and compared with data taken from the existing literature about Sardinia and Calabria.
The geodynamic implications of our results are discussed in order to add new constraints for unravelling the complex Tertiary geological evolution of the Central-Southern Apennines and the surrounding areas.
2. Geological framework
The Central-Southern Apennines (Fig. 1) form part of the Apennine Chain, a long (about 1500 km), arcuate collisional chain connecting the Western Alps to the Maghrebian Chain of Northern Africa (Vezzani, Festa & Ghisetti, Reference Vezzani, Festa and Ghisetti2010).
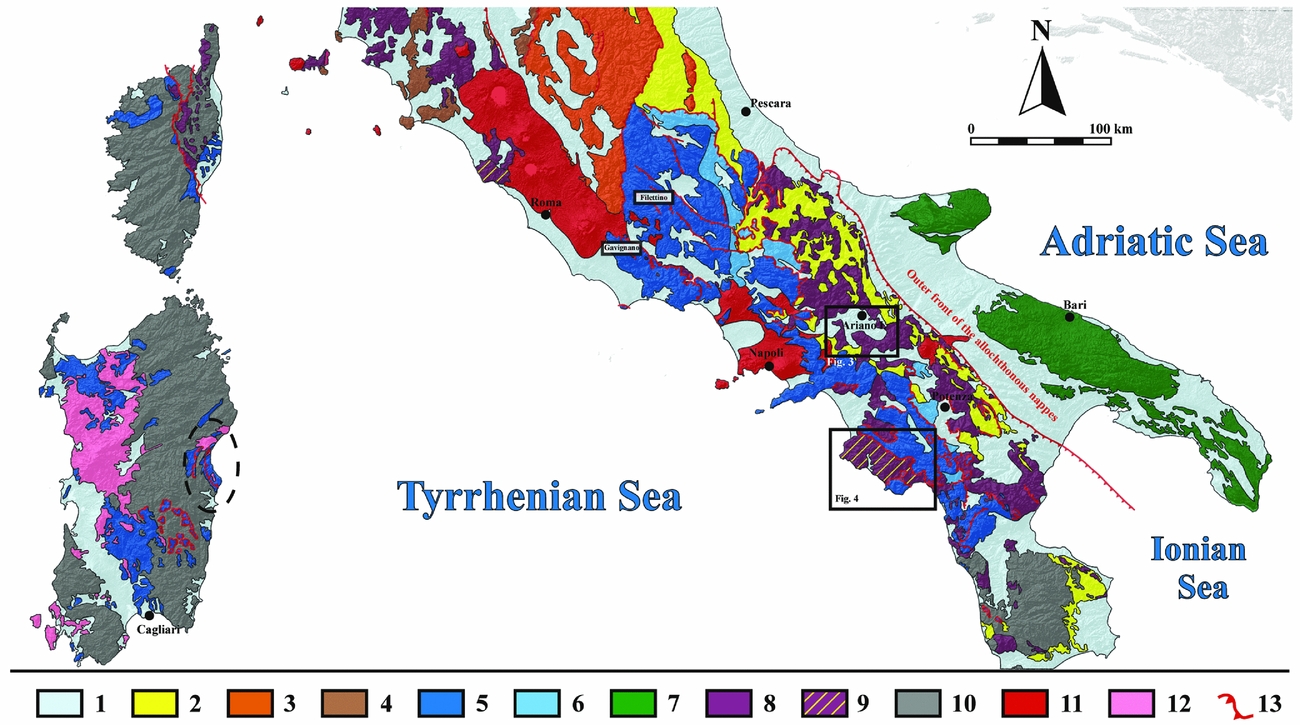
Figure 1. Simplified geological map of the Southern Apennines and Corsica and Sardinia Islands (modified from Bigi et al. Reference Bigi, Cosentino, Parotto, Sartori, Scandone, Carrozzo, Luzio, Margiotta and Quarta1992). Key to symbols: 1, Plio-Quaternary deposits (except volcanic rocks); 2, Cenozoic terrigenous units; 3, Umbria−Marche sedimentary units (Upper Triassic – Miocene); 4, Tuscany sedimentary units (Upper Triassic – Oligocene); 5, sedimentary cover of the European sedimentary wedge (mainly shallow-water limestones: Latium–Abruzzi and Campano–Lucana carbonate platforms) (Middle Triassic – Miocene); 6, Meso-Cenozoic pelagic sedimentary units of the Southern Apennines (including the Lagonegresi–Molisane units); 7, Apula carbonate platform (Upper Triassic – Miocene); 8, ocean-derived units, including ophiolites and varicoloured clays (Cretaceous–Eocene); 9, Pietraforte and Cilento sedimentary units (Upper Cretaceous – Miocene); 10, Palaeozoic basement units (Corsica, Sardinia and Calabria); 11, Cenozoic volcanic rocks (Italian Peninsula); 12, Cenozoic volcanic rocks (Sardinia); and 13, main thrusts. The black ellipse indicates the source area of the exotic-clast-bearing conglomerates of the Southern Apennines.
The Apenninic chain still preserves tectonostratigraphic imprints of superposed events of rifting, drifting, subduction and collision related to the interaction between the African and European plates since Triassic time (e.g. Cavazza, Roure & Ziegler, Reference Cavazza, Roure, Ziegler, Cavazza, Roure, Spakman, Stampfli and Ziegler2004; Dilek, Reference Dilek, Dilek and Pavlides2006; Patacca & Scandone, Reference Patacca, Scandone, Mazzotti, Patacca and Scandone2007). The roughly N–S convergence between the two plates started in Late Cretaceous time (Alpine orogeny: Trümpy, Reference Trümpy, De Jong and Scholten1973; Castellarin & Transalp Working Group, Reference Castellarin, Crescenti, D'Offizi, Merlino and Sacchi2004) and determined the closure of the Tethys Ocean and the development of the Alpine collisional chain extended from Gibraltar to the present-day Alps (Alvarez Reference Alvarez1976, Reference Alvarez1991), whose relics are well preserved in Eastern Sardinia (Arragoni et al. Reference Arragoni, Maggi, Cianfarra and Salvini2016) and Corsica (Maggi et al. Reference Maggi, Rossetti, Corfu, Theye, Andersen and Faccenna2012, Reference Maggi, Rossetti, Ranalli and Theye2014; Rossetti et al. Reference Rossetti, Glodny, Theye and Maggi2015). The opening of the Tyrrhenian Sea since Langhian time (Kastens & Mascle, Reference Kastens and Mascle1990; Sartori et al. Reference Sartori, Torelli, Zitellini, Carrara, Magaldi and Mussoni2004) determined the eastwards translation of part of the deformed Meso-Cenozoic sedimentary covers of the chain. These were detached from their basement to form the Apenninic orogen. This event produced the NE-directed migration of a compressional front (Boccaletti et al. Reference Boccaletti, Ciaranfi, Cosentino, Deiana, Gelati, Lentini, Masari, Moratti, Pescatore, Ricci Lucchi and Tortorici1990; Cipollari & Cosentino, Reference Cipollari and Cosentino1995; Cosentino, Cipollari & Pipponzi, Reference Cosentino, Cipollari and Pipponzi2003), currently located in the Adriatic Sea (Favali et al. Reference Favali, Funiciello, Mattietti, Mele and Salvini1993; D'Agostino et al. Reference D'Agostino, Avallone, Cheloni, D'Anastasio, Mantenuto and Selvaggi2008). The compressional deformation was accompanied by extension on the eastern side of the Tyrrhenian Sea and the facing Apennines (e.g. Carmignani et al. Reference Carmignani, Decandia, Fantozzi, Lazzarotto, Liotta and Meccheri1994; Vai & Martini, Reference Vai and Martini2001; Brogi et al. Reference Brogi, Lazzarotto and Liotta2005; Arragoni, Martini & Sandrelli, Reference Arragoni, Martini and Sandrelli2012; Martini et al. Reference Martini, Arragoni, Aldinucci, Foresi, Bambini and Sandrelli2013).
In the Southern Apennines the tectonically superposed units include the Liguride units (Cretaceous–Eocene: Ogniben, Reference Ogniben1969; Knott, Reference Knott1987) together with the ‘varicoloured clays’ (Angelucci, Devoto & Farinacci, Reference Angelucci, Devoto and Farinacci1963; Angelucci & Devoto, Reference Angelucci and Devoto1966) whose present-day structural position (e.g. Monaco & Tortorici, Reference Monaco and Tortorici1995; Catalano et al. Reference Catalano, Monaco, Tortorici, Paltrinieri and Steel2004; Tortorici, Catalano & Monaco, Reference Tortorici, Catalano and Monaco2009) does not unequivocally clarify their palaeogeographic position. This tectonic pattern determined the formation of several tectonically controlled basins in which terrigenous sequences accumulated since Cenozoic time (e.g. Martini, Sagri & Colella, Reference Martini, Sagri, Colella, Vai and Martini2001; Patacca & Scandone, Reference Patacca, Scandone, Vai and Martini2001). The studied conglomerates were deposited prior or contemporary to these sequences.
3. Exotic-clast-bearing conglomerates in the Central-Southern Apennines
The Tertiary terrigenous units of the Central-Southern Apennines include a great variety of deposits representing a wide range of ages and sedimentary environments (Vezzani, Festa & Ghisetti, Reference Vezzani, Festa and Ghisetti2010).
Despite the substantial lack of basement outcrops along this segment of the chain (from the Ancona–Anzio line down to the Calabrian Arc), several of these terrigenous units contain crystalline and metamorphic clasts of internal (i.e. westwards in present-day coordinates) provenance (Civitelli & Corda, Reference Civitelli and Corda1982; Sartori, Mascle & Amaudric du Chaffaut, Reference Sartori, Mascle, Amaudric du Chaffaut, Kastens, Mascle and Auroux1987) whose geodynamic significance is still puzzling.
In this work we investigate four main sedimentary units scattered along the whole chain from north to south, namely: Filettino (the northernmost outcrop, 70 km east of Rome); Gavignano and surroundings (50 km SE of Rome); Ariano Irpino (80 km NNE of Naples); and Cilento (the southernmost and largest unit, 100 km SE of Naples). In the following sections these sedimentary units are referred to by the name of the main outcrop locality.
3.a. Filettino
The Filettino conglomerates form a small outcrop (about 0.5 km2) on the northern flank of Monte Viglio (Filettino, 41°55′26′′N, 13°22′32′′E). Poor exposure conditions and the small size of the outcrop have attracted only a little interest previously (Beneo, Reference Beneo1943; Devoto, Reference Devoto1967 a, b; Accordi et al. Reference Accordi, Carbone, La Monica, Praturlon, Sirna and Zalaffi1969), which resulted in uncertain interpretations of their origin and stratigraphic position. On the other hand, their precise dating is not so important for this study as the focus is the origin of the exotic clasts. Devoto (Reference Devoto1967 b) attributed these deposits to the Fosso Fioio Unit (Messinian), describing an unconformable base over the Upper Cretaceous units of the deformed Mesozoic carbonate platform. Nevertheless, this contact is not clearly exposed today. The unit is about 50 m thick and consists of thick beds (up to 1 m) of polygenic conglomerates with well-rounded clasts, mainly made of shallow-water limestones, sandstones, older conglomerates and rare yellow micritic limestones. Clasts are poorly sorted, ranging from granules to cobbles, and are often aligned parallel to bedding (Fig. 2a). The sedimentation of the Filettino conglomerates can be related to mass-wasting processes, in particular to unconfined cohesionless debris flows (Nemec & Steel Reference Nemec, Steel, Koster and Steel1984; Nemec et al. Reference Nemec, Steel, Porebski, Spinnangr, Koster and Steel1984; see also Lowe Reference Lowe1982).
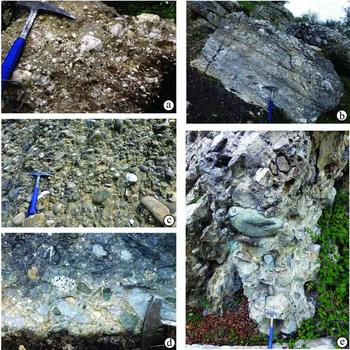
Figure 2. Main features of the studied conglomeratic units. (a) Filettino conglomerates; (b) Gavignano conglomerates; (c) Ariano Irpino conglomerates; (d) Cilento conglomerates; and (e) Cilento conglomerates, top of Monte Gelbison.
A ‘Lago Mare’ environment (Orszag-Sperber, Reference Orszag-Sperber2006) was proposed by Cosentino & Cipollari (Reference Cosentino and Cipollari2012) for similar deposits cropping out in the surrounding areas, thus implying a Miocene age.
3.b. Gavignano
The Gavignano-Gorga Unit (Alberti et al. Reference Alberti, Bergomi, Catenacci, Centamore, Cestari, Chiocchini, Chiocchini, Manganelli, Molinari-Paganelli, Panseri-Crescenzi, Salvati and Tilia-Zuccari1975) is exposed in scattered outcrops around the Gavignano village (50 km SE of Rome; 41°42′N, 13°3′E) and, to a lesser extent, close to the Gorga village (41°39′21′′N, 13°6′40′′E).
Alberti et al. (Reference Alberti, Bergomi, Catenacci, Centamore, Cestari, Chiocchini, Chiocchini, Manganelli, Molinari-Paganelli, Panseri-Crescenzi, Salvati and Tilia-Zuccari1975) recognized three lithofacies in this unit, which from base to top are as follows.
-
− Lower conglomeratic lithofacies. This is made of massive or crudely bedded clast-supported conglomerates with a sandy matrix and carbonate cement (Fig. 2b). The clasts are generally well rounded and poorly sorted, and display rare imbrications. They are composed of Cretaceous and Miocene limestones from the local succession with subordinate exotic Pietraforte-like sandstones. This unit unconformably overlies Upper Cretaceous carbonate-platform limestones and presents a maximum thickness of about 20 m.
-
− Chaotic lithofacies. This sharply overlies the previous lithofacies and consists of very poorly sorted (from granules to boulders), angular to sub-angular clasts set in a muddy sandstone matrix with carbonate cement. The clasts are mainly from exotic lithologies: green marlstones, turbidite sandstones, ‘Pietra-paesina’ pelagic marly limestones, marly limestones with black and white chert, micritic limestones and subordinate igneous acidic rocks, mainly granites with pink feldspars and rhyolites with porphyritic texture. Due to its outcrop conditions and chaotic nature, the thickness of this unit cannot be precisely evaluated.
-
− Upper conglomeratic lithofacies. This comprises massive or thick-bedded clast-supported conglomerates with a sandy matrix and carbonate cement. The clasts are poorly sorted and consist of varied exotic lithologies: Eocene micritic limestones with planktonic foraminifera (not reported from the underlying carbonate-platform succession); reddish chert; alveolinid grainstones; and rare microgranular granites. The total thickness of this lithofacies does not exceed 20 m.
Additionally, the three lithofacies contain scattered clasts composed of older conglomerates.
The age of these deposits is uncertain although, according to the resedimented faunas, they should be younger than Late Miocene (Alberti et al. Reference Alberti, Bergomi, Catenacci, Centamore, Cestari, Chiocchini, Chiocchini, Manganelli, Molinari-Paganelli, Panseri-Crescenzi, Salvati and Tilia-Zuccari1975). Regarding the palaeo-environmental interpretation, the whole unit can be interpreted as alluvial-fan deposits (Nemec & Steel, Reference Nemec and Steel1988; Reading, Reference Reading1996). In particular, the lower and upper conglomeratic units can be related to channelized debris flows, while the chaotic unit was probably deposited from unconfined debris flows (Nemec & Steel, Reference Nemec, Steel, Koster and Steel1984). This probably reflects an alternation of progradational and retrogradational fan phases, leading to the vertical stacking of proximal channelized reaches and distal lobes.
3.c. Ariano Irpino
This area presents many different terrigenous units (Fig. 3) related to the Mio-Pliocene evolution of the Central-Southern Apennines and recording the eastwards migration of the fold-and-thrust belt. As in the previous cases, the nature of the successions and the outcrop scarcity preclude precise regional correlations (Amore et al. Reference Amore, Bonardi, Ciampo, De Capoa, Perrone and Sgorsso1988 b) that are anyway beyond the purpose of the present study.

Figure 3. Simplified geological map of Ariano Irpino−Trevico area (modified from SGN, 1963). Key to symbols: 1, Recent alluvial deposits; 2, Pliocene sandstones and clays; 3, Pliocene conglomerates (Trevico member of the Baronia Formation); 4, Miocene sandstones; 5, Miocene clays; 6, Frigento Formation (Miocene): marls and silty clays with alternations of marly limestones and quartz-arenites; 7, varicoloured clays (uncertain age); 8, Cretaceous units; 9, main faults; and 10, location of the collected samples.
The Pliocene deposits include several terrigenous units (SGN, 1963) resting on a mainly Miocene substrate including terrigenous, carbonate and evaporitic (gypsum) deposits (Jacobacci & Martelli, Reference Jacobacci and Martelli1967). The area presents an undifferentiated assemblage of varicoloured clays of uncertain age containing Triassic and Cretaceous olistoliths and broken terrigenous and calcareous units, among which jaspers and pelagic limestones stand out.
In the first regional synthesis for these units, Ippolito et al. (Reference Ippolito, D'Argenio, Pescatore and Scandone1973) grouped all the terrigenous formations deposited after Middle Pliocene time in the Ariano Unit. D'Argenio, Pescatore & Scandone (Reference D'Argenio, Pescatore and Scandone1975) recognized two main sedimentary cycles of early and middle Pliocene age. These were attributed by Amore et al. (Reference Amore, Basso, Ciarcia, Di Nocera, Matano, Torre, Ciampo, Di Donato, Esposito and Staiti1998) to the Ariano Group, in particular the Baronia (Zanclean–Piacenzian) and the Torrente Cervaro (middle Pliocene) formations. Each formation represents a complete shallowing and coarsening-upwards cycle, from clays to sandstones and conglomerates (Ciarcia & Torre, Reference Ciarcia and Torre1996).
Our study is focused on the Baronia Formation, in particular the Trevico Conglomerate member (BNA5 in Chiocchini, Reference Chiocchini2007; Torre, Di Nocera & Matano, Reference Torre, Di Nocera and Matano2007), cropping out close to Ariano Irpino and Trevico villages (SGN, 1963, 2007). This unit is constituted by thick-bedded or massive, polygenic, clast-supported conglomerates showing plane-parallel lamination or gently inclined cross-bedding and occasional normal grading (Fig. 2c). Clasts are well rounded and poorly sorted, ranging from granules to pebbles with rare cobbles. They mainly consist of turbiditic sandstones and limestones from the varicoloured clays and the Mesozoic carbonate platforms (Chiocchini & Chiocchini, Reference Chiocchini and Chiocchini1999). Conglomerate clasts from older conglomeratic units and rare acidic igneous (granitoids) and metamorphic clasts are also present.
The age of the Trevico Conglomerates is late Zanclean – Piacenzian (Chiocchini, Reference Chiocchini2007; Torre, Di Nocera & Matano, Reference Torre, Di Nocera and Matano2007), while the depositional setting can be related to a fluvial environment, probably dominated by gravel and subordinate sand bars (Reading, Reference Reading1996).
3.d. Cilento
The Cilento region is located on the western side of the Southern Apennines and the eastern coast of the Tyrrhenian Sea (Fig. 4). According to Martelli & Nardi (Reference Martelli and Nardi2005), the outcropping formations can be grouped in two sets: (1) Alburno–Cervati and Monte Bulgheria carbonate units (Campano–Lucana carbonate platform); and (2) ‘Internidi Units’, presenting a mainly terrigenous character.

Figure 4. Simplified geological map of the Cilento area (modified from SGN, 1969). Key to symbols: 1, Recent alluvial deposits; 2, Monte Sacro Conglomerates (Paleocene–Oligocene); 3, San Mauro Formation (Paleocene–Oligocene); 4, Pollica Formation (Upper Cretaceous – Paleocene); 5, Ascea Formation (Cretaceous); 6, Campano–Lucana carbonate platform (Monte Bulgheria and Alburno–Cervati units, Upper Triassic – Miocene); 7, main faults; and 8, location of the collected samples.
The former are constituted by Upper Triassic – lower Miocene carbonate-platform deposits (Selli, Reference Selli1957, Reference Selli1962; Cocco, Reference Cocco1971; Barattolo & Parente, Reference Barattolo, Parente, Barattolo, De Castro and Parente1991; Torrente et al. Reference Torrente, Civile, Martino and Milia2000) which tectonically overlie (Monte Bulgheria Unit, SE Cilento) and are overlain by the Internidi deposits (Alburno–Cervati Unit, NE Cilento).
The dominantly terrigenous Internidi Units were interpreted as occupying a palaeogeographic position more ‘internal’ (i.e. closer to the palaeo-ocean and westwards in present-day coordinates) than the Campano–Lucana carbonate platform (one of the Apenninic carbonate platforms), since they were deposited on transitional to oceanic crust (Mostardini & Merlini, Reference Mostardini and Merlini1986; Sgrosso, Reference Sgrosso1986). Due to their sedimentary features and structural position, these units were correlated to the Liguride Complex of the Northern Apennines (Ogniben, Reference Ogniben1969; Mostardini & Merlini, Reference Mostardini and Merlini1986; Bonardi et al. Reference Bonardi, Amore, Ciampo, De Capoa, Miconnet and Perrone1988 a; Bigi et al. Reference Bigi, Cosentino, Parotto, Sartori, Scandone, Carrozzo, Luzio, Margiotta and Quarta1992). More recently, Monaco & Tortorici (Reference Monaco and Tortorici1995) and Catalano et al. (Reference Catalano, Monaco, Tortorici, Paltrinieri and Steel2004) clarified the relation between the Liguride Complex and the other units cropping out in the Southern Apennines with an evolutionary model. Our study on the provenance of the exotic clasts in the Central-Southern Apennines allows to better frame this proposed evolution within the previous Alpine collisional chain.
The terrigenous deposits of the Cilento region consist of pelagic turbidites showing a clayey-calcareous and clayey-sandy composition. They are divided into three tectonic units (e.g. Martelli & Nardi, Reference Martelli and Nardi2005), which are overlain by the Cilento Group (Amore et al. Reference Amore, Bonardi, Ciampo, De Capoa, Perrone and Sgorsso1988 a) and the Monte Sacro Conglomerates (Selli, Reference Selli1962; De Pippo & Valente, Reference De Pippo and Valente1991).
The Cilento Group (previously referred to as ‘Cilento Flysch’; Ietto, Pescatore & Cocco, Reference Ietto, Pescatore and Cocco1965; Cieszkowski et al. Reference Cieszkowski, Malata, Oszczypko, Pescatore, Senatore, Ślączka and Valente1992, Reference Cieszkowski, Oszczypko, Pescatore, Ślączka, Senatore, Valente, Carannante and Tonielli1994) crops out all over the Cilento area, notably in the Monte Stella and Vallo della Lucania sectors. It includes the Pollica and San Mauro formations (Cocco, Reference Cocco1971), composed of sandy turbidites with frequent conglomerate beds. The conglomerates are generally matrix-supported, polygenic and comprise poorly sorted, moderately rounded to angular granules to cobbles formed of crystalline and metamorphic basement rocks. The matrix is composed of coarse to very coarse sand-grade particles from metamorphic and acidic igneous rocks, and subordinately from limestones and sandstones. The presence of ophiolite blocks has been referred to by Dietrich & Scandone (Reference Dietrich and Scandone1972), Di Girolamo, Morra & Perrone (Reference Di Girolamo, Morra and Perrone1991) and Mazzeo et al. (Reference Mazzeo, De Vita, Aulinas, Arienzo, Cirillo, Iovine and Sparice2016) at Monte Centaurino, east of our study area.
The Pollica and San Mauro formations have been assigned Late Cretaceous – Paleocene and Paleocene–Oligocene ages, respectively (Cocco, Reference Cocco1971) even if, based on either literature or merely relative stratigraphic/geometric positions, younger ages have been proposed (e.g. Martelli & Nardi, Reference Martelli and Nardi2005). This discrepancy may easily derive from different approaches by authors in defining the depositional ages and may reflect successive reworking or tectonic stacking.
The Monte Sacro Conglomerates, with a total thickness of about 600 m, include six lithofacies (De Pippo & Valente, Reference De Pippo and Valente1991) ranging from coarse conglomerates to clays. Clasts are made of sedimentary, mainly acidic, igneous and metamorphic rocks.
Conglomerates are generally matrix-supported, chaotic, massive and formed of very poorly sorted granules to boulders. Clasts are angular to moderately rounded, depending on their composition (Fig. 2d). Limestones clasts are usually less rounded than those of metamorphic and igneous rocks. The metamorphic fragments tend to have a rectangular shape due to the presence of pervasive foliation, while the igneous fragments are generally well rounded.
The conglomerates show a clear coarsening- and thickening-upwards trend, with very thick (up to 20 m) massive beds composed of boulders floating in a coarse sandy matrix on top of the succession (Monte Gelbison; Fig. 2e).
According to SGN (1969), the age of the Monte Sacro Conglomerates (included in the San Mauro Formation) is Oligocene–Paleocene; Martelli & Nardi (Reference Martelli and Nardi2005) however suggested a rather short depositional interval in early Tortonian time for this 600 m thick unit, based on their mere stratigraphic/geometric position. Nonetheless, this dating is still uncertain due to the nature of the deposits, the lack of biostratigraphic markers and the unclear relations with the underlying formations.
The general features of the Monte Sacro Conglomerates, dominated by alternating unconfined and channelized debris-flow deposits and showing a coarsening- and shallowing-upwards trend, suggest a fan-delta setting, probably passing upwards to an alluvial fan (Critelli & Le Pera, Reference Critelli and Le Pera1990, Reference Critelli and Le Pera1994; De Pippo & Valente, Reference De Pippo and Valente1991; Martelli & Nardi, Reference Martelli and Nardi2005).
4. Methodology
This work is based on the study of selected outcrops from the conglomeratic units (Filettino, Gavignano, Ariano Irpino and Cilento) and laboratory analyses on selected samples. In particular, 70 stations were chosen for sampling and collection of detailed outcrop photographs, photomosaics and, when possible, general stratigraphic logs. Most of the field work was performed in the Cilento area, which exhibits the best and most continuous (both laterally and vertically) conglomerate outcrops containing exotic clasts.
The average lithologic composition of each unit was estimated from fieldwork and the analysis of 21 pictures using the graphic ImageJ software (developed at the National Institutes of Health, NIH, USA). For every picture, at least 3000 points were counted. These data were integrated with those from the literature.
With the aim of producing statistically significant sampling of the existing conglomerate population, a total of 258 samples were collected: 15 from Filettino, 16 from Gavignano, 86 from Ariano Irpino and 141 from the Cilento area. The samples were randomly taken, where possible, among the exotic clasts, focusing in particular on the igneous lithologies. Clasts of sedimentary rocks as well as the conglomerate matrix were also sampled.
A total of 78 samples was selected to make thin-sections for petrographic studies: 35 of the igneous clasts and 43 of the sedimentary clasts (including the conglomerate matrix). From the total set, 23 thin-sections of the intrusive lithologies and 24 of the matrix of the conglomerates and sandstone clasts were analysed by means of a point-counter stage (1000 points per section) in order to obtain the modal composition of the main constituents of the rock. For the classification, the modal compositions were plotted on a quartz – alkali feldspar – plagioclase (QAP) diagram for the intrusive rocks (Streckeisen, Reference Streckeisen1974) and on a quartz – feldspar – lithic fragments (QFL) diagram for the sandstones (Zuffa, Reference Zuffa1980; Dickinson et al. Reference Dickinson, Beard, Brakenridge, Erjavec, Ferguson, Inman, Knepp, Lindberg and Ryberg1983).
4.a. Major- and trace-element analyses
From the total sample set, 27 representative igneous clasts were selected for major- and trace-element analyses (including rare Earth elements or REE; Table 1). Given the nature of the samples, the size of the clast represented a significant discriminating element and only the clasts larger than 64 mm (cobbles and boulders) were analysed.
Table 1. Major, minor and REE composition of the analysed samples.

g – granitoid; gc – cordierite-bearing granite; s-v – subvolcanic.
Major elements were analysed by x-ray fluorescence (XRF) in the Technical-Scientific Services of Oviedo University (Spain) using a WD-XRF spectrometer (model 2404, PANalytical) coupled with a Rh tube. Analyses were performed using glass beads of powdered rocks after fusion with lithium tetraborate. The estimated error is smaller than 1%.
Trace- and rare Earth elements were analysed by inductively coupled plasma mass spectrometry (ICP-MS) following sample decomposition with lithium metaborate at the Geochronology and Geochemistry-SGIker facility of El País Vasco university/EHU (Spain) (for further details see García de Madinabeitia, Sánchez Lorda & Gil Ibarguchi, Reference García de Madinabeitia, Sánchez Lorda and Gil Ibarguchi2008).
The results have been analysed and plotted using the GCDkit software, version 4.1 (Janoušek, Farrow & Erban, Reference Janoušek, Farrow and Erban2006).
5. Sedimentary features and petrography
5.a. Filettino
The clasts of the Filettino conglomerates are mainly composed of grey-whitish limestones (57–85%) and sandstones (15–43%). The relative proportion of these main components changes through the unit, with an upwards increase of the percentage of the carbonate clasts. Sandstones are dominantly lithic-arenites with different degrees of lithification, as shown by the even or irregular (pitted) clast surfaces. Rare (usually less than 1%) yellow micritic limestones have been found. According to Devoto (Reference Devoto1967 b), very rare igneous clasts (granitoids) are also present; nonetheless they were not found in this study.
The 15 samples were collected throughout the conglomeratic unit and comprise limestones (11 samples) and sandstones (4 samples). The former are dominantly shallow-water carbonates probably eroded from the surrounding Mesozoic carbonate formations, as also indicated by their low roundness. No igneous or metamorphic clasts were found.
The conglomerate matrix represents 21–55% of the rock, its grain size is medium to coarse sand and its composition differs from that of the clasts. It is composed of quartz (11–17%), chert (1–4%), feldspars (8–12%) and lithic fragments (34–53%); it therefore plots in the ‘litharenite’ field of the QFL diagram (Fig. 5a). The main components show the following features: quartz grains are well rounded and display a bimodal grain-size distribution (modes: 0.2–0.4 mm and 0.6–0.7 mm); feldspars are generally represented by angular grains (some of which are almost idiomorphic; Fig. 6a) with a bimodal distribution (modes: 0.2 mm and 0.4 mm); scattered coarser grains (up to 1.2 mm) are present; lithic fragments are mainly of sedimentary rocks (limestones and rare sandstones) and subordinate metamorphic and igneous fragments (mainly granitoids, when recognizable).
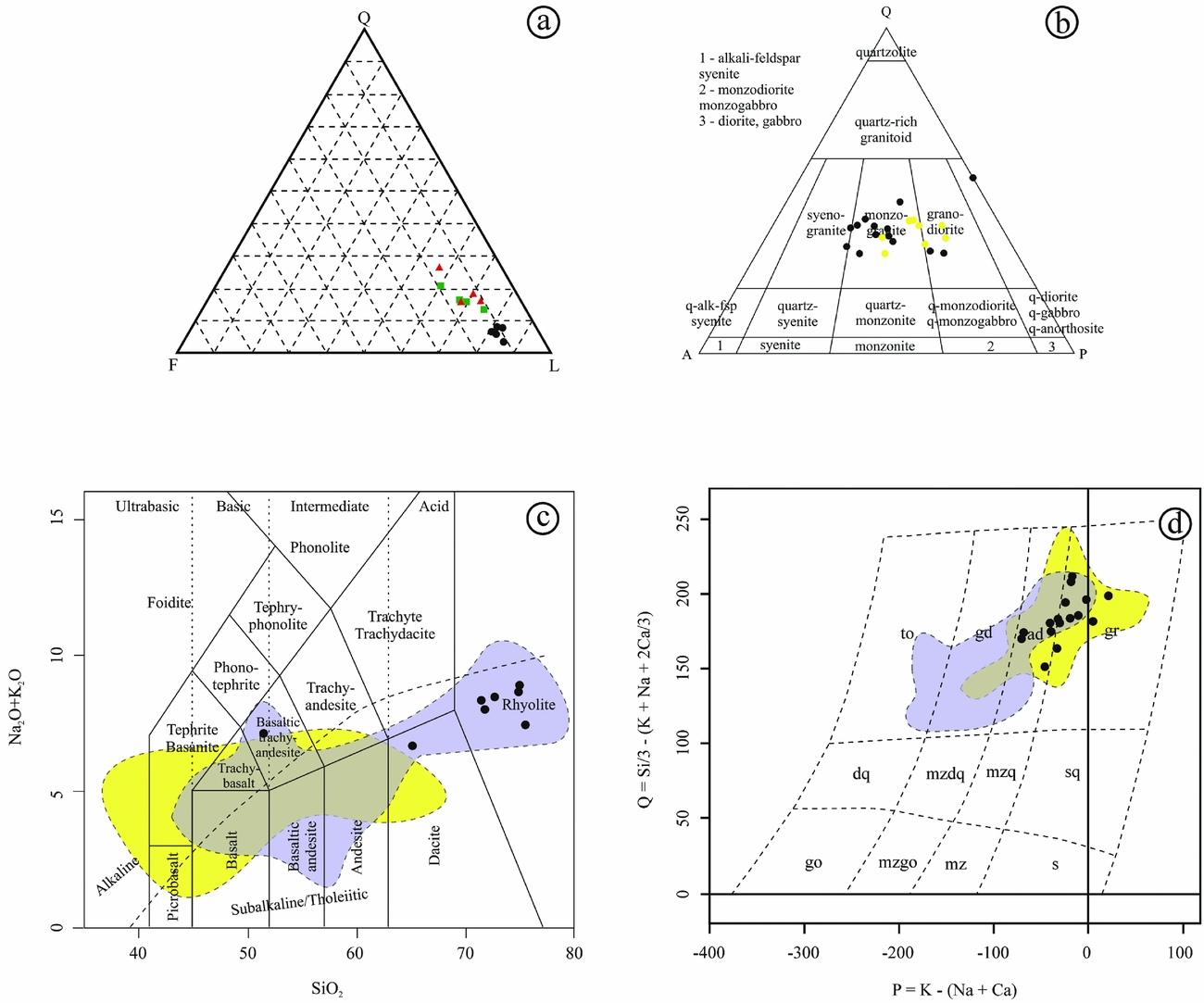
Figure 5. (a) QFL diagram of the arenaceous matrix of the conglomerates. Black dots: Cilento samples; red triangles: Filettino samples; green squares: Gavignano samples. (b) QAP diagram of the intrusive samples (Streckeisen, Reference Streckeisen1974). Yellow dots indicate cordierite-bearing granitoids. (c) TAS diagram (Le Bas et al. Reference Le Bas, Le Maitre, Streckeisen and Zanettin1986) of the subvolcanic samples; (d) P-Q diagram (Debon & LeFort, Reference Debon and LeFort1983) of the intrusive samples. The shaded areas indicate the field of existence of rocks from the two possible source areas. Yellow: Calabria; violet: Sardinia (data taken from the literature, see text).

Figure 6. Photographs of representative thin-sections from the studied conglomeratic units. (a) Filettino conglomerates, sample FIL01c. Note the presence of feldspars, quartz and peloidal/bioclastic limestones (black fragments). (b) Gavignano conglomerates, sample VOL01a. Note the presence of feldspars, quartz, shallow-water limestones and large benthic foraminifera (centre of the image). (c) Cordierite-bearing granite, sample CIL25a; crossed Nicols. (d) Porphyritic rhyodacite, sample CIL16, crossed Nicols. (e) Subvolcanic basaltic trachy-andesite, sample CIL04a, crossed Nicols. The texture is given by the plagioclase crystals. (f) Monzogranite, sample CIL11a, crossed nichols. Mineral abbreviations (Whitney & Evans, Reference Whitney and Evans2010). Afs – alkali feldspar; Qz – quartz; Pl – plagioclase; Bt – biotite; Crd – cordierite; Chl – chlorite.
5.b. Gavignano
The composition of the whole unit is dominated by calcareous fragments (90–94%) and subordinate sandstones (6–10%). In contrast to the Filettino conglomerates, no clear trends in clast composition are present.
The 16 collected samples come from the 3 different lithofacies and are composed of sedimentary rocks, limestones (5 samples) and sandstones (11 samples). No igneous or metamorphic rocks were found. The limestones are dominantly shallow-water carbonates, some with gastropods, molluscs and rare benthic foraminifera (Fig. 6b).
The matrix is very similar to that of the Filettino conglomerates. It represents 40–51% of the rock and its grain size varies between medium and coarse sand. The most striking feature of the matrix is its different composition with respect to clasts. It is made of quartz (11–16%), chert (0–2%), feldspars (9–15%) and igneous and metamorphic rock fragments (45–64%). It can therefore be classified as ‘litharenite’ in the QFL diagram (Fig. 5a). The main characteristics of the grains are: quartz grains are usually well rounded, with a bimodal grain size distribution (modes: 0.3–0.4 mm and 0.6–0.7 mm); feldspar grains are angular, nearly intact crystals, some of which are almost idiomorphic (Fig. 6b) and also show a bimodal size distribution (0.2 mm and 0.4–0.6 mm), although coarser grains (up to 1.5 mm) are present; lithic fragments are mainly of sedimentary rocks (33–57% of the matrix), in particular of limestones with subordinate sandstones; and the rest is made up of metamorphic (3.5%–10% of the matrix) and igneous (8–20% of the matrix) fragments (the latter are dominantly granitoids when recognizable).
5.c. Ariano Irpino
The 86 collected samples come from different stratigraphic levels of the Baronia Formation, in particular the Trevico Conglomerates member (BNA5 of Chiocchini, Reference Chiocchini2007 and Torre, Di Nocera & Matano, Reference Torre, Di Nocera and Matano2007) (Fig. 3). They are represented by sedimentary (65 samples, of which 14 limestones, 43 sandstones and 8 cherts/jaspers) and igneous (21 samples) rocks. The latter generally show a high degree of alteration, which varies with the average clast size and rounding. In particular, the coarser clasts are usually less rounded and weathered than the finer ones. The igneous samples are entirely represented by acidic intrusive lithotypes (granitoids), in particular monzogranites and tonalites according to the modal composition (Fig. 5b, d; Table 2).
Table 2. Modal composition of selected igneous samples.

The matrix is scarce (less than 10%) and even locally absent, resulting in an openwork conglomerate. It comprises reddish medium- to coarse-grade sand, composed of quartz, chert, feldspars and lithic fragments. The latter are mainly of sedimentary rocks (sandstones and subordinate limestones) with minor metamorphic and acidic igneous fragments. Given the scarcity of the matrix, no detailed compositional studies have been performed.
5.d. Cilento
The collected samples come from the Cilento Group (Pollica and San Mauro formations) and the Monte Sacro Conglomerates. The conglomerate clasts are composed of sedimentary, metamorphic and igneous rocks. Their relative proportion varies widely among the studied outcrops.
The number of clasts of sedimentary rocks varies from 0% to 77% and they range from granules to cobbles. They dominantly comprise grey limestones and subordinately sandstones. Limestone clasts in some cases contain shallow-water fauna (gastropods and benthic foraminifera). These have undergone selective dissolution leading to moldic pores, in some cases with remnants of the dissolved clast.
Clasts of metamorphic rocks (representing 2–52% in the studied outcrops) are mainly represented by phyllites, schists, rare gneisses and quartzites as well as by grains of metamorphic quartz. Subordinate whitish-yellow marble clasts have also been found. Clast size ranges from granules to boulders 40–50 cm in diameter and they show different degrees of rounding. Foliated lithologies (phyllites, schists) tend to yield more angular clasts than non-foliated quartzites and marbles).
Clasts of igneous rocks (representing 17–70% in the studied outcrops) are composed almost exclusively of acidic rocks, both intrusive (granitoids) and subvolcanic with a porphyritic texture (rhyolites and dacites). Clasts are usually well rounded and vary from granules to boulders 40–50 cm in size.
This composition is in agreement with data from Critelli & Le Pera (Reference Critelli and Le Pera1990) and Critelli (Reference Critelli1991).
There is an upwards increase in the abundance of igneous and metamorphic versus sedimentary clasts. This is in contrast with De Pippo & Valente (Reference De Pippo and Valente1991), who reported an opposite trend. This compositional variation is accompanied by a coarsening-upwards trend, from an average clast size of about 3 cm at the base to 12–15 cm in the uppermost beds. The igneous clasts show a larger upwards increase of the average size with respect to the others, while the metamorphic clasts show no clear variations.
The 141 samples have been collected from several stratigraphic layers of the San Mauro and Monte Sacro Conglomerates formations. They comprise sedimentary (12 samples), igneous (106 samples) and metamorphic (3 samples) rocks. Finally, the matrix of the conglomerate has been studied in 18 samples. The sedimentary clasts are composed of sandstones (10 samples) and limestones (2 samples), while the igneous clasts are represented by dominantly acidic rocks which can be grouped into two subsets: intrusive (87 samples) and subvolcanic porphyritic rocks (19 samples).
Intrusive lithologies are represented by syenogranites, monzogranites and granodiorites according to their modal composition (Figs 5b, 6f; Table 2) and the P-Q diagram of Debon & LeFort (Reference Debon and LeFort1983; Fig. 5d). It is important to note the abundance of cordierite-bearing granites (8 out of 18 analysed samples: CIL05, CIL11, CIL11a, CIL24b, CIL24c, CIL25a, CIL27 and CIL28; Fig. 6c).
Three samples (CIL07a, CIL25b, CIL31c) show intense hydrothermal alteration as a result of fluid–rock interaction after solidification. In particular, the rocks were subjected to silica depletion with the formation of episyenites (Cathelineau, Reference Cathelineau1986; Recio et al. Reference Recio, Fallick, Ugidos and Stephens1997).
Subvolcanic lithologies are represented by porphyritic rocks, mainly rhyolites and dacites according to the total alkali versus silica (TAS) diagram of Le Bas et al. (Reference Le Bas, Le Maitre, Streckeisen and Zanettin1986) (Figs 5c, 6d). Only one of the analysed samples is composed of a basaltic trachy-andesite (CIL04a, Figs 5c, 6e).
The matrix of the conglomerate is very abundant, usually representing 55–86% of the rock. It is dominantly composed of lithic fragments (45–85%, mainly metamorphic and igneous acidic rock fragments), with subordinate quartz grains (usually less than 10%), feldspars (10–15%) and micas (usually less than 2%).
6. Geochemistry
The geochemical analysis of the igneous clasts of the studied Tertiary conglomeratic units from the Central-Southern Apennines represents a good test to establish the genetic relationship among the samples collected in the four localities and the known igneous complexes of Sardinia and Calabria. These have been identified as the most probable source areas due to the presence of crystalline and metamorphic basement rocks, and their palaeo-position before the opening of the Tyrrhenian Sea.
We compared the major- and minor-element (including REE) composition of the selected clasts and the igneous rocks of the Palaeozoic basement of Sardinia and Calabria. A similar methodology has been successfully applied to establish the provenance of igneous clasts contained in conglomeratic units from different localities (e.g. Spain: Rubio Ordóñez et al. Reference Rubio Ordóñez, Barba, Cuesta, Gallastegui, Suárez, Ugidos and Valladares2004; Morocco: Gigliuto et al. Reference Gigliuto, Ouazani-Touhami, Puglisi, Puglisi and Najib Zaghloul2004; Argentina: Gallastegui et al. Reference Gallastegui, González-Menéndez, Rubio Ordóñez, Cuesta and Gerdes2014).
In each diagram data from the analysed samples (black dots) are plotted together with those from Sardinia and Calabria basement rocks (coloured shaded areas). The latter geochemical data have been taken from Ferla (Reference Ferla1978), Ioppolo & Puglisi (Reference Ioppolo and Puglisi1980), D'Amico et al. (Reference D'Amico, Rottura, Maccarrone and Puglisi1981), Messina et al. (Reference Messina, Russo, Stagno and Calandra1988, Reference Messina, Russo, Perrone and Giacobbe1991), Messina, Russo & Lima (Reference Messina, Russo and Lima1993) and Fiannacca et al. (Reference Fiannacca, Cirrincione, Bonanno and Carciotto2015) in the case of the calabrian rocks and from Di Simplicio et al. (Reference Di Simplicio, Ferrara, Ghezzo, Guasparri, Pellizzer, Ricci, Rita and Sabatini1974), Del Moro et al. (Reference Del Moro, Di Simplicio, Ghezzo, Guasparri, Rita and Sabatini1975), Traversa & Vaccaro (Reference Traversa, Vaccaro, Carmignani and Sassi1992), Di Vincenzo, Andriessen & Ghezzo (Reference Di Vincenzo, Andriessen and Ghezzo1996), Cortesogno et al. (Reference Cortesogno, Cassinis, Dallagiovanna, Gaggero, Oggiano, Ronchi, Seno and Vanossi1998), Barbey et al. (Reference Barbey, Gasquet, Pin and Bourgeix2008), Cruciani et al. (Reference Cruciani, Franceschelli, Musumeci, Spano and Tiepolo2013) in the case of Sardinia.
The results have been plotted on TAS (Cox, Bell & Pankhurst, Reference Cox, Bell and Pankhurst1979; Le Bas et al. Reference Le Bas, Le Maitre, Streckeisen and Zanettin1986) and P-Q diagrams (Debon & LeFort, Reference Debon and LeFort1983; diagram based on major elements composition and equivalent to the QAP) in order to make a geochemical classification of the studied samples.
6.a. Classification
The analysed samples from the Central-Southern Apennines are made of different lithologies, as shown in the petrography section. This variation in the composition is also observed in their geochemical features, summarized in the diagrams of Figures 5c, d, 7–10. Intrusive and subvolcanic rocks have been plotted separately in order to better evidence their characteristics and test their compatibility with analogous rocks from the Palaeozoic basements.
A first approach to the classification includes the use of two different diagrams depending on the nature of the rocks: a major-element-based multicationic scheme for granitoids in a broad sense (Debon & LeFort, Reference Debon and LeFort1983); and the Nb/Y versus Zr/TiO2 diagram (Winchester & Floyd, Reference Winchester and Floyd1977) for the subvolcanic porphyritic clasts. Using these diagrams, the granitoid rocks are classified as adamellites and granites (Fig. 5d) with a peraluminous character as expected in most of granites, and particularly in cordierite-bearing granites (Clarke, Reference Clarke1995), while the subvolcanic rocks are classified in a compositional range from andesites to rhyolites (Fig. 8a). It is important to evidence the clear dominance of acidic lithotypes. Both the SiO2 versus FeOt/MgO diagram (Miyashiro, Reference Miyashiro1974) and the alkalis–iron–magnesium (AFM) diagram (Irvine & Baragar, Reference Irvine and Baragar1971) indicate that the porphyritic clasts fit well to a calc-alkaline series (Figs 8b, 8c). The Harker variation diagrams (Harker, Reference Harker1909) (Figs 9, 10) show some correlation between SiO2 and major elements (Fig. 9a, b). In particular, SiO2 correlates well with Al2O3, MgO, CaO, TiO2 and FeOt. On the contrary, plotting SiO2 versus trace elements (Fig. 10a, b) shows much more scattering and no clear correlations can be established. The meaning of these variations is beyond the scope of this work and will not be discussed.
The REE normalized patterns (Boynton, Reference Boynton and Henderson1984) (Figs 7d, 8d) have been used as a further element to better characterize the collected samples and test their compatibility with the hypothesized source areas. Both intrusive and subvolcanic rocks show similar trends with enrichment in light REEs (LREEs), a marked Eu negative anomaly and almost flat heavy REEs (HREEs). This is a common pattern for many granitic rocks (e.g. Gallastegui et al. Reference Gallastegui, González-Menéndez, Rubio Ordóñez, Cuesta and Gerdes2014; Dauphas & Pourmand, Reference Dauphas and Pourmand2015). An Ho negative anomaly can be noted for both groups; its significance and the possible explanations are beyond the scope of this work, however.

Figure 7. Geochemical features of the analysed intrusive samples: (a) P-Q diagram (Debon & LeFort, Reference Debon and LeFort1983); and (b) REE normalized patterns (Boynton, Reference Boynton and Henderson1984). The shaded areas indicate the field of existence of intrusive rocks from the two possible source areas. Yellow: Calabria; violet: Sardinia (data taken from the literature, see text).

Figure 8. Geochemical features of the analysed porphyritic samples. (a) Zr/TiO2 v. Nb/Y diagram (Winchester & Floyd, Reference Winchester and Floyd1977); (b) FeOt/MgO v. SiO2 diagram (Miyashiro, Reference Miyashiro1974); (c) AFM diagram (Irvine & Baragar, Reference Irvine and Baragar1971). (d) REE normalized patterns (Boynton, Reference Boynton and Henderson1984). The shaded areas indicate the field of existence of porphyritic rocks from the two possible source areas. Yellow: Calabria; violet: Sardinia (data taken from the literature, see text). No data are available for REE patterns of porphyritic rocks from Calabria.
Each diagram shows the possible geochemical compatibility between the igneous exotic clasts from the Central-Southern Apennines and the Calabria and Sardinia basement rocks. The analysed samples (black dots) are plotted together with the compositional fields of both source regions (shaded coloured areas). It is possible to note that no clear discriminations exist for the igneous clasts between Sardinia and Calabria, since the respective fields overlap and no distinct groups can be made (Figs 7, 9a, 10a).
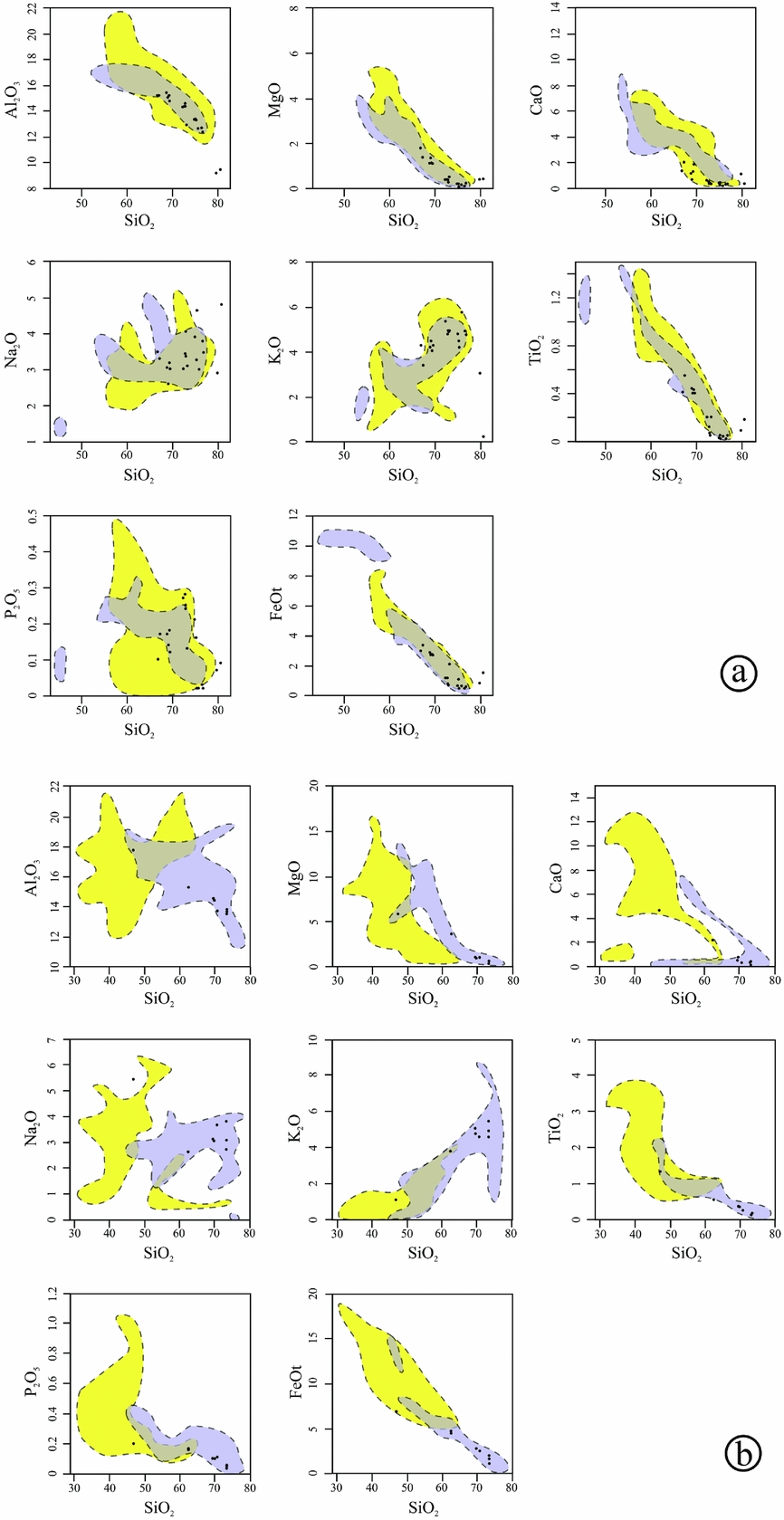
Figure 9. Harker diagrams of major elements (Al2O3, MgO, CaO, Na2O, K2O, TiO2, P2O5, FeOt) v. SiO2: (a) intrusive samples and (b) porphyritic subvolcanic samples. The shaded areas indicate the field of existence of basement rocks from the two possible source areas. Yellow: Calabria; violet: Sardinia (data taken from the literature, see text).

Figure 10. Harker diagrams of trace elements v. SiO2: (a) intrusive samples and (b) porphyritic subvolcanic samples. The shaded areas indicate the field of existence of basement rocks from the two possible source areas. Yellow: Calabria; violet: Sardinia (data taken from the literature, see text). No data are available for Ba, Cr, Ni, La and Ce composition of porphyritic rocks from Calabria.
On the contrary, a net discrimination can be made on the subvolcanic analysed samples since Sardinia and Calabria basement rocks plot in clearly separated regions of the diagrams (Figs 8, 9b, 10b). These results and their implications are extensively discussed in the following section.
7. Discussion: comparison with Sardinian and Calabrian basement rocks
The petrography and geochemistry of the exotic clasts collected in four conglomeratic units of the Central-Southern Apennines indicate that their composition is dominantly acidic, with only one sample showing a more basic composition. Despite the relatively homogeneous composition the geochemical features are quite varied, indicating provenances from magmatic bodies with different characteristics.
In order to test the compatibility of the collected samples with the Sardinia and Calabria magmatic basement units and discriminate between them, we analysed the rocks separately according to their main features; in particular, a separation was made between intrusive and subvolcanic porphyritic lithologies.
The diagrams show that no real geochemical distinction exists between the Sardinia and Calabria intrusive rocks. This is not a surprising result, since the two areas shared a common late Variscan evolution and were part of the same block before the Burdigalian drifting (Boccaletti & Guazzone, Reference Boccaletti and Guazzone1974; Alvarez, Reference Alvarez1976; Minzoni, Reference Minzoni, Ojakangas, Dickas and Green1995; Vai, Reference Vai, Vai and Martini2001). This common evolution lasted at least until Oligocene time when the opening of the Liguro–Provençal Basin separated these two blocks from the French–Spanish coast (e.g. Alvarez, Reference Alvarez1976; Speranza et al. Reference Speranza, Villa, Sagnotti, Florindo, Cosentino, Cipollari and Mattei2002; Advokaat et al. Reference Advokaat, van Hinsbergen, Maffione, Langereis, Vissers, Cherchi, Schroeder, Madani and Columbu2014). The later (Langhian: Kastens & Mascle, Reference Kastens and Mascle1990; Sartori et al. Reference Sartori, Torelli, Zitellini, Carrara, Magaldi and Mussoni2004) opening of the Tyrrhenian Sea determined the separation between Sardinia and Calabria, and the latter reached its present-day position.
Evidence of this common geological evolution also exists in the sedimentary cover of Calabria (e.g. Amodio-Morelli et al. Reference Amodio-Morelli, Bonardi, Colonna, Dietrich, Giunta, Ippolito, Liguori, Lorenzoni, Paglionico, Perrone, Piccarreta, Russo, Scandone, Zantettin-Lorenzoni and Zuppetta1976; Santantonio & Teale, Reference Santantonio, Teale, Legget and Zuffa1987; Passeri et al. Reference Passeri, Ciarapica, Reggiani and Rutledge2014) that shows analogies with East Sardinia carbonate successions (Dieni et al. Reference Dieni, Fischer, Massari, Salard-Cheboldaeff and Vozenin-Serra1983; Dieni & Massari, Reference Dieni, Massari and Cherchi1986 b; Arragoni et al. Reference Arragoni, Maggi, Cianfarra and Salvini2016).
The comparison between Sardinia (Poli, Ghezzo & Conticelli, Reference Poli, Ghezzo and Conticelli1989; Di Vincenzo, Andriessen & Ghezzo, Reference Di Vincenzo, Andriessen and Ghezzo1996) and Calabria (Atzori, Pezzino & Rottura, Reference Atzori, Pezzino and Rottura1977; D'Amico et al. Reference D'Amico, Rottura, Maccarrone and Puglisi1981; Del Moro et al. Reference Del Moro, Pardini, Maccarrone and Rottura1982; Messina et al. Reference Messina, Russo, Perrone and Giacobbe1991; Fiannacca et al. Reference Fiannacca, Williams, Cirrincione and Pezzino2008) cordierite-bearing granites yielded the same results, with no clear petrographic and geochemical discrimination between them. This again indicates the common evolution of these two crustal blocks until Oligocene time. Nonetheless the abundance of cordierite-bearing lithotypes in the collected samples (about 30% of the collected igneous clasts) indicates the relative proximity of at least one cordierite-bearing intrusive body. Similar rocks are also present in Tuscany (e.g. Taylor & Turi, Reference Taylor and Turi1976; Peccerillo & Donati, Reference Peccerillo and Donati2003) but their relatively young age and geographical position preclude them as a source of the studied conglomeratic units, in particular the Monte Sacro Conglomerates of Cilento.
Considering only the intrusive clasts, their petrographic and geochemical features do not allow any discrimination between Sardinia and Calabria as source areas. The studied samples from the Central-Southern Apennines are compatible with both basements and there is no clear distinction among them.
On the contrary, the comparison between the analysed porphyritic clasts from the Cilento area and the analogous rocks of the Sardinia basement show a good petrographic and geochemical compatibility which is not evident with the Calabria porphyritic rocks. In particular, the analysed conglomerate clasts and the Sardinia porphyritic rocks present a similar geochemical signature (Fig. 8b, 8c). Also the relations between SiO2 and major and trace elements (as shown in the Harker diagrams of Figs 9b, 10b) and the REE patterns (Fig. 8d) show this geochemical affinity (although REE data for Calabria are not available).
We did not analyse the age of the collected samples and the related magmatic bodies. They may have been emplaced in the Sardinia and Calabria basements in distinct phases from the end of the Variscan Orogeny (e.g. Lombardi, Cozzupoli & Nicoletti, Reference Lombardi, Cozzupoli and Nicoletti1974; Ferla, Reference Ferla1978; Cozzupoli et al. Reference Cozzupoli, Gerbasi, Nicoletti and Petrucciani1984; Buzzi, Gaggero & Oggiano, Reference Buzzi, Gaggero and Oggiano2008) to Eocene time (Alpine tectonic phase in Sardinia; Arragoni et al. Reference Arragoni, Maggi, Cianfarra and Salvini2016). Nonetheless, the presented geochemical affinity may suggest Sardinia as the primary source area for the porphyritic clasts of the Monte Sacro Conglomerates of Cilento. Given the similar sedimentary features (dimensions and degree of roundness and alteration), this is also valid for the analysed intrusive clasts.
A further element to identify the source area is provided by the total composition and sedimentary characteristics of the studied conglomerates. The dimensions of the clasts of the Cilento conglomerates and their sedimentary features indicate a short travel distance. As a consequence, the composition of the Cilento conglomerates is directly related to the lithologies cropping out in the source area. The relative volumetric proportions of the clasts of the conglomerates seem to be compatible with the Eastern Sardinia area where an analogous suite of rocks crops out, namely: granitoids (also cordierite-bearing granites, e.g. the San Basilio intrusion: Di Vincenzo, Andriessen & Ghezzo, Reference Di Vincenzo, Andriessen and Ghezzo1996); subvolcanic acidic rocks (dacitic and rhyolitic porphyries); metamorphic units (dominantly phyllites and schists compatible with the clasts present in the Monte Sacro Conglomerates, ‘Filladi Grigie del Gennargentu’ Formation: Oggiano & Di Pisa, Reference Oggiano and Di Pisa2001); and sedimentary units, in particular Mesozoic carbonate-platform limestones (Monte Tului and Monte Bardia formations; Amadesi et al. Reference Amadesi, Cantelli, Carloni and Rabbi1960; Dieni & Massari, Reference Dieni, Massari and Cherchi1986 b).
Eastern Sardinia may therefore represent the primary source area in Cenozoic time for the conglomerates of the Cilento region. The debated age of these formations (e.g. SGN, 1969; Martelli & Nardi, Reference Martelli and Nardi2005) may relate to possible resedimentation or mere geometric relations, and does not affect the results of this study. This is in good agreement with the presence of an uplifting Cenozoic fold-and-thrust belt (Arragoni et al. Reference Arragoni, Maggi, Cianfarra and Salvini2016). The erosion of this growing orogen would provide the mixed sedimentary and metamorphic/igneous material that is currently contained in the Pollica, San Mauro and Monte Sacro Conglomerates formations (Fig. 11a). As evidenced in Section 5 ‘Sedimentary features and petrography’, there is a clear increase of the metamorphic and igneous clasts with respect to the sedimentary ones towards the top of the succession of the Monte Sacro Conglomerates. The litharenite composition of their matrix (Fig. 5a) indicates the less mature character of these deposits compared to the other studied conglomerates (Filettino, Gavignano and Ariano Irpino sedimentary units).

Figure 11. Interpretative sketch of the studied conglomeratic units. (a) Erosion of the uplifting Eastern Sardinia fold-and-thrust belt and deposition of the Cilento conglomerates containing exotic basement clasts (Cenozoic). (b) Opening of the Tyrrhenian Sea and eastwards (in present-day coordinates) translation of the Cilento units on top of deformed Meso-Cenozoic sedimentary units (Langhian). (c) Second phase of deformation involving the Meso-Cenozoic sedimentary units detached from their basement and the Cilento conglomerates. Miocene sediments accumulate unconformably on top of the previous units (upper Miocene – Pliocene). (d) Uplift of the Southern Apennines. The erosion and recycling of Cilento conglomerates and analogous deposits determine the deposition of Filettino, Gavignano and Ariano Irpino conglomerates.
All these features indicate the progressive dismantling of an uplifting orogen, in which a dominantly carbonate sedimentary cover overlies a Palaeozoic basement strongly involved in the compressional deformation. This detritus would feed a sedimentary basin located eastwards of the mountain chain (in present-day coordinates; Fig. 11a), whose succession records the inverse stratigraphy of the orogen. Palaeocurrent directions in the San Mauro and Monte Sacro Conglomerates formations show a dominant provenance from the western and southwestern sectors (in present-day coordinates; Cocco, De Magistris, & De Pippo, Reference Cocco, De Magistris and De Pippo1978; De Pippo & Valente, Reference De Pippo and Valente1991; Valente, Reference Valente1991; Ciarcia, Vitale & Lo Schiavo, Reference Ciarcia, Vitale and Lo Schiavo2011), which is in good agreement with this interpretation. A sedimentary unit with a similar geodynamic significance is represented by the Cuccuru ‘e Flores Conglomerates (Dieni & Massari, Reference Dieni and Massari1965; Dieni, Massari & Médus, Reference Dieni, Massari and Médus2008; Oggiano et al. Reference Oggiano, Funedda, Carmignani, Pasci, Dieni and Massari2011) of the Golfo di Orosei region (Eastern Sardinia). Their composition testifies the erosion and dismantling of the uplifting Cenozoic fold-and-thrust belt of Eastern Sardinia (Arragoni et al. Reference Arragoni, Maggi, Cianfarra and Salvini2016).
The age and sedimentary features of the analysed conglomeratic units indicate that the Cilento conglomerates are the oldest and that they were directly connected to the Eastern Sardinia source area. On the other hand, the features of the Ariano Irpino deposits indicate the recycling of a conglomeratic unit similar to the Cilento unit. This is evidenced by the lesser proportion of igneous and metamorphic clasts, which show a higher degree of rounding and weathering and a finer average grain size, and by the presence of conglomerate clasts. All these features suggest that the source area contained an older conglomerate unit made of basement clasts, which was subjected to erosion and cannibalization in a continental depositional setting (Fig. 11b, c, d). A significant clast input from the sedimentary units (mainly limestones and sandstones) of the Ariano Irpino substrate resulted in a lowered proportion of exotic clasts.
The Filettino and Gavignano units, in turn, would represent a different stage of reworking. In these units, the exotic basement clasts are almost absent due to their destruction by post-depositional weathering before they could be transported into the basin and the composition of the succession is dominated by sedimentary clasts, mainly derived from the surrounding Mesozoic carbonate units. Exotic material is represented by the abundant sandstone clasts and the rare pelagic-limestone clasts contained in the conglomerates. These are the product of the dismantling of pelagic sedimentary units, which cannot be clearly identified today but which should lie in a more westwards position (in present-day coordinates) with respect to the current outcrops, as suggested by the palaeocurrent indications.
The matrix of the Filettino and Gavignano conglomeratic units indicates a provenance from an area containing metamorphic and igneous rocks, as evidenced by the presence of abundant, almost euhedral feldspars and crystalline lithic fragments. These particles are interpreted to result from the breaking down of the metamorphic and igneous clasts of the gravels present in the previous basin, now under recycling. The recycling of this previous basin constitutes a mixed source, yielding the basement clasts of its gravels and Mesozoic sedimentary, mainly calcareous, clasts from its substrate. The bimodality of the quartz grains suggests a possible double provenance from crystalline rocks and pre-existing sandstones. Quartz grains deriving from sandstone recycling would be finer grained on average due to their polycyclic origin.
This allows us to conclude that the Cilento conglomerates would represent a primary deposit, directly fed by the Eastern Sardinia basement rocks. On the contrary, the Ariano Irpino, Filettino and Gavignano units represent secondary deposits, formed by the erosion and mixing between in situ sedimentary units and exotic clasts from the dismantling of pre-existing conglomerates and gravels which contained metamorphic and igneous clasts.
The geodynamic implications of these results are noteworthy since they suggest a link between the Cilento area, and subsequently the Central-Southern Apennines Meso-Cenozoic rocks, and the Cenozoic Eastern Sardinia fold-and-thrust belt.
In our study, sedimentary, petrographic and geochemical results suggest the proximity of the European continent to the Central-Southern Apennines carbonate-platform successions at least after the formation of the Alpine collisional belt, whose erosion produced the studied exotic clasts.
The successive opening of the Tyrrhenian Sea determined the fragmentation of the pre-existing Alpine chain and the separation between Sardinia, Calabria and the Central-Southern Apennines (Fig. 11b). During this second phase of deformation, the Meso-Cenozoic sedimentary cover of the Central-Southern Apennines was detached from its basement and involved in a further compressional event which determined its current structural location and final assembly (Fig. 11c, d). In this way, the studied conglomeratic deposits, directly connected and fed by the Sardinian orogen, participated in this detachment and became incorporated in the Apenninic chain as evidence of the dismantled Alpine chain.
8. Conclusions
The study of the sedimentary, petrographic and geochemical features of the exotic clasts from the conglomeratic units of the Central-Southern Apennines provides new evidence for the reconstruction of the geodynamic evolution of the Central Mediterranean area in Cenozoic time. The presence of exotic basement clasts in areas where only Meso-Cenozoic sedimentary rocks crop out is an important element to link the geological evolution of the Central-Southern Apennines to Sardinia.
Petrographic, geochemical and sedimentary analyses indicate a compatibility among these exotic clasts and a source area located approximately in present-day Eastern Sardinia. This is also confirmed by the average composition of the conglomerates, which show a suite of rocks typical of an uplifting orogen compatible with that recognized by Arragoni et al. (Reference Arragoni, Maggi, Cianfarra and Salvini2016) in the Golfo di Orosei region (Eastern Sardinia).
The sedimentary features of the four studied conglomeratic units allow a primary (i.e. directly fed by the Sardinian source area) conglomerate, represented by the Cilento Unit, and secondary conglomerates, derived from the erosion and reworking of older conglomeratic units bearing exotic basement clasts (Fig. 11), to be recognized.
The proximity of the studied conglomeratic units to the European continent evidenced by the presented results, and the lack of Meso-Cenozoic sedimentary units at the bottom of the Tyrrhenian Sea (where Mio-Quaternary sediments lie directly on the Variscan basement: DSDP, 1978; Sartori et al. Reference Sartori, Torelli, Zitellini, Carrara, Magaldi and Mussoni2004) may imply that a part of the carbonate platforms of the Central-Southern Apennines was located on the European margin before the opening of the Tyrrhenian Sea. These Meso-Cenozoic units underwent a first phase of deformation during Eocene-Oligocene time, resulting in a double-verging orogen whose relics are preserved in Eastern Sardinia (Golfo di Orosei region; Fig. 11a). The consequent uplift of this Cenozoic Alpine orogen led to strong erosion and the accumulation of sedimentary units such as the Cilento conglomerates.
The opening of the Tyrrhenian Sea since Langhian time split apart this first fold-and-thrust chain, leading to the detachment of its cover formed by the thrusted units of the European and African passive margins to form the present-day Apenninic chain.
The Cilento conglomerates were fed by the erosion of the former fold-and-thrust Alpine chain and were detached and thrusted on the eastern side of the Tyrrhenian Basin together with the underlying carbonate-platform units (Fig. 11b, c). Erosion and reworking of these conglomerates and other analogous units formed re-deposited conglomerates such as the Filettino, Gavignano and Ariano Irpino units (Fig. 11d).
These results add new robust evidence for understanding the complex geodynamic evolution of the Central Mediterranean since early Cenozoic time, and provide new clues for the reconstruction of the relations between the European and African margins with the development of the Alpine fold-and-thrust belt. The studied exotic clasts represent the relics of this chain.