14 results
Chapter 20 - Land and Water: Linkages to Bioenergy
-
-
- Book:
- Global Energy Assessment
- Published online:
- 05 September 2012
- Print publication:
- 27 August 2012, pp 1459-1526
-
- Chapter
- Export citation
References
-
- Book:
- A Systems Analysis of the Global Boreal Forest
- Published online:
- 12 January 2010
- Print publication:
- 05 March 1992, pp 471-544
-
- Chapter
- Export citation
Index
-
- Book:
- A Systems Analysis of the Global Boreal Forest
- Published online:
- 12 January 2010
- Print publication:
- 05 March 1992, pp 545-565
-
- Chapter
- Export citation
16 - The biological component of the simulation model for boreal forest dynamics
-
-
- Book:
- A Systems Analysis of the Global Boreal Forest
- Published online:
- 12 January 2010
- Print publication:
- 05 March 1992, pp 428-445
-
- Chapter
- Export citation
Introduction
-
-
- Book:
- A Systems Analysis of the Global Boreal Forest
- Published online:
- 12 January 2010
- Print publication:
- 05 March 1992, pp 308-312
-
- Chapter
- Export citation
Contents
-
- Book:
- A Systems Analysis of the Global Boreal Forest
- Published online:
- 12 January 2010
- Print publication:
- 05 March 1992, pp vii-viii
-
- Chapter
- Export citation
1 - Introduction
-
-
- Book:
- A Systems Analysis of the Global Boreal Forest
- Published online:
- 12 January 2010
- Print publication:
- 05 March 1992, pp 1-8
-
- Chapter
- Export citation
Part 1 - Processes in boreal forests
-
- Book:
- A Systems Analysis of the Global Boreal Forest
- Published online:
- 12 January 2010
- Print publication:
- 05 March 1992, pp -
-
- Chapter
- Export citation
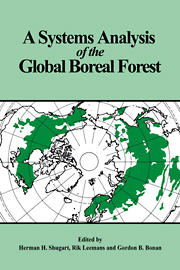
A Systems Analysis of the Global Boreal Forest
-
- Published online:
- 12 January 2010
- Print publication:
- 05 March 1992
Part 3 - Computer models for synthesis of pattern and process in the boreal forest
-
- Book:
- A Systems Analysis of the Global Boreal Forest
- Published online:
- 12 January 2010
- Print publication:
- 05 March 1992, pp -
-
- Chapter
- Export citation
Part 2 - Patterns in space and time in boreal forests
-
- Book:
- A Systems Analysis of the Global Boreal Forest
- Published online:
- 12 January 2010
- Print publication:
- 05 March 1992, pp -
-
- Chapter
- Export citation
List of contributors
-
- Book:
- A Systems Analysis of the Global Boreal Forest
- Published online:
- 12 January 2010
- Print publication:
- 05 March 1992, pp ix-xii
-
- Chapter
- Export citation
Frontmatter
-
- Book:
- A Systems Analysis of the Global Boreal Forest
- Published online:
- 12 January 2010
- Print publication:
- 05 March 1992, pp i-vi
-
- Chapter
- Export citation
18 - Concluding comments
-
-
- Book:
- A Systems Analysis of the Global Boreal Forest
- Published online:
- 12 January 2010
- Print publication:
- 05 March 1992, pp 466-470
-
- Chapter
- Export citation