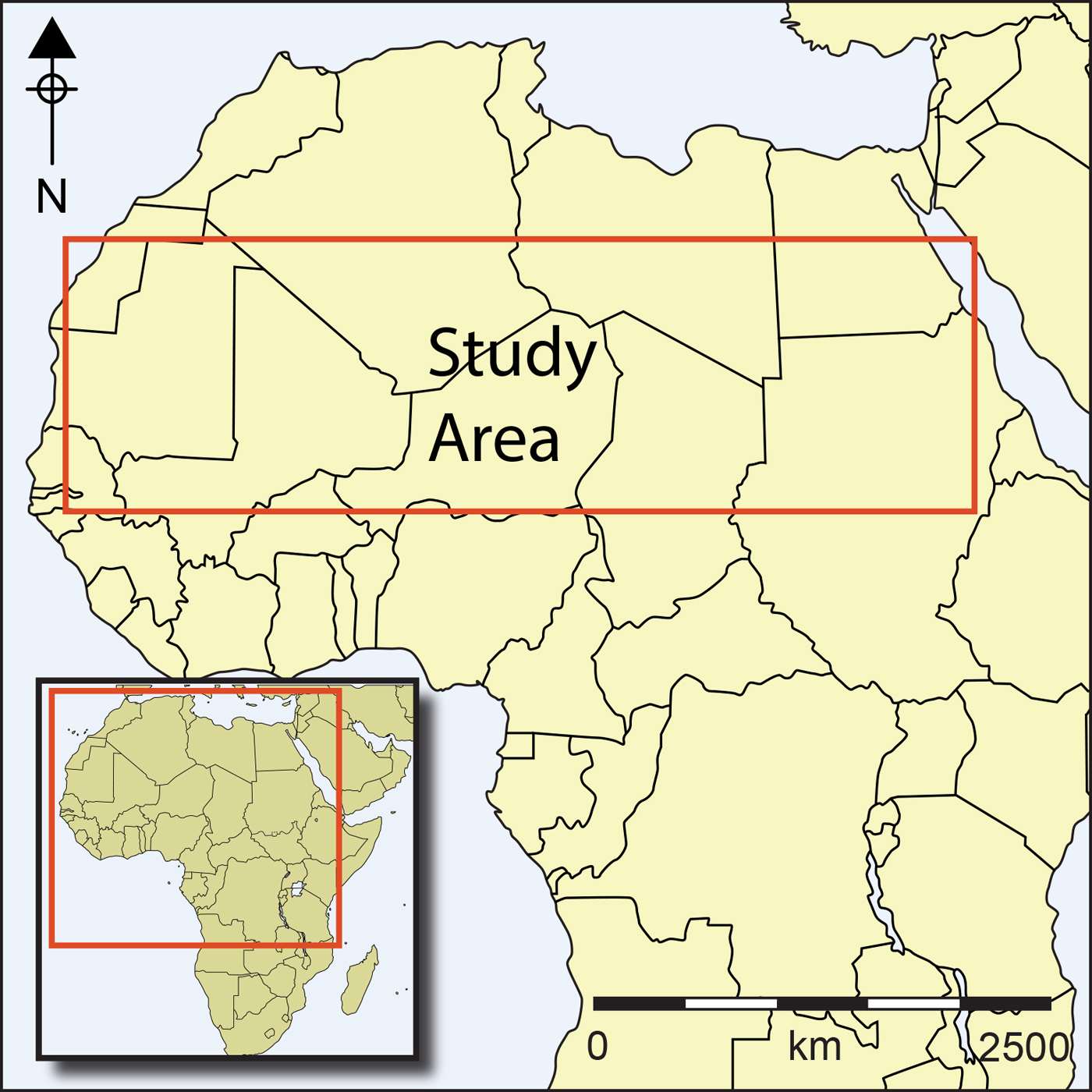
Introduction
Today, drylands cover approximately 40 per cent of the world's land area and host around 40 per cent of the world's population. The United Nations Environment Programme defines drylands as tropical and temperate areas with an aridity index of less than 0.65, including hyper-arid drylands or deserts with an aridity index below 0.05 (Safariel & Adeel Reference Safriel, Adeel, Hassan, Sholes and Ash2005: 626). These areas are especially sensitive to climate change, shifts in precipitation patterns and increases in extreme climatic and meteorological events (Huang et al. Reference Huang2017). Nonetheless, drylands have been inhabited for thousands of years, and are today home to societies that display a wide array of adaptive behaviours. Such practices, evidenced by traditional ecological knowledge, are key to the resilience of these communities.
In this article, we present a review of rain-fed agriculture in the absence of water-harvesting structures in the arid and hyper-arid lands of North Africa; farming in the Sahara is a surprisingly common practice even though it is omitted from most maps of current and past land use. On the basis of historical records and our field observations, we argue that this type of rain-fed agriculture in arid and hyper-arid lands is an overlooked practice that holds huge potential for designing effective strategies to cope with current climate-driven desertification. Further, we suggest that rain-fed cultivation without water-harvesting techniques might have played a so-far unrecognised role in the development of food production in arid areas in the past, even where rainfall is normally considered too scarce for crop cultivation.
In Old World contexts, considerable research has been directed to the study of the origin of agriculture, focusing especially on the Near East—one of the cradles of domestication—and crops such as wheat and barley that provide the foundation for many modern agricultural systems. It is clear, however, that past human groups also experimented with a diverse assemblage of crops, some of which (e.g. millets) are well adapted to areas with water constraints. Recent work by Winchell et al. (Reference Winchell, Brass, Manzo, Beldados, Perna, Murphy, Stevens and Fuller2018) has traced the domestication trajectories of African crops, including sorghum (Sorghum bicolor (L.) Moench) and pearl millet (Pennisetum glaucum (L.) R.Br). Past cultivation practices related to these crops, including how water was managed, however, are still under study.
In this article, we first provide an overview of rain-fed cultivation of cereals in the arid and hyper-arid lands of North Africa, based upon the study of ethnographies from the nineteenth and twentieth centuries, as well as a few more recent observations. We then present the results from Al Khiday, Sudan, where a multidisciplinary pilot investigation on rain-fed crops was carried out in 2015–2016. This case study has driven the development of a larger research project (RAINDROPS), featuring an experimental methodology aimed at identifying crop-water availability and management directly from archaeobotanical remains.
A review of rain-fed cultivation in arid and hyper-arid North Africa
In arid lands, irrigation, a permanent water source, or some form of rainwater-harvesting is usually deemed necessary to support cultivation. There are modern examples, however, that demonstrate the presence, even in hyper-arid areas, of successful rain-fed cultivation systems. For the purposes of this article, we employ the following definitions:
a) Irrigated cultivation: water is provided to crops at regular intervals throughout the growing season by human intervention.
b) Décrue cultivation: water is provided by natural inundation, typically from major river systems (floodplain cultivation).
c) Rain-fed cultivation: water is provided by rainfall alone (directly or as run-off); cultivation occurs far from any permanent water sources (e.g. rivers, wells, lakes or cisterns) and without any water harvesting.
This last type, rain-fed cultivation in arid and hyper-arid environments, has received comparatively little research attention as it is often considered to play only a minor role in human livelihoods. Although the harvest yielded by this type of cultivation is lower compared to irrigated or décrue cultivation, its benefits as an adaptive strategy in drylands can be important. Indeed, rain-fed crops, such as millets and sorghum, may offer a considerable contribution to a predominantly pastoral economy, as they provide carbohydrates and a high percentage of daily protein and minerals (Gulia et al. Reference Gulia, Wilson, Carter, Singh, Janick and Whipkey2007). Such crops are currently identified as ‘future smart foods’ (Li & Siddique Reference Li and Siddique2018), as they have the potential to offer better options than traditional crops, such as wheat/barley or corn, for mitigating the effects of environmental and, especially, climate change, and to supply affordable and sustainable dietary diversity.
North Africa is dominated by the Sahara, which is often considered to be agriculturally ‘inactive’ (Rockström & Falkenmark Reference Rockström and Falkenmark2015). Indeed, land-use models and maps of North Africa generally characterise the Sahara and its margins as a huge empty area that stretches from the northern edge of the Sahel up to the Mediterranean coast and the Atlas Mountains (Rockström & Falkenmark Reference Rockström and Falkenmark2015). Similarly, modern technical reports and studies on dryland food production tend to regard the Sahara as being devoid of cultivation (Portmann et al. Reference Portmann, Siebert and Döll2010).
The perceived dominance of pastoralism, together with the notion that hot deserts are unsuitable for growing crops, seems to have obscured the role that cultivation has played in the past. While therefore not surprising that the extent of rain-fed modern and ancient agriculture in the Sahara is poorly understood, observations published over the past two centuries indicate the practice of crop cultivation in different parts of the Sahara (Figure 1). In central Mauritania, for example, where rainfall averages 170mm/year, rain-fed crops were observed in the early 1960s, and so-called pastoral communities were reportedly growing cereals without irrigation (Toupet Reference Toupet and Bataillon1963).

Figure 1. CGIAR-CSI aridity index and the localities mentioned in the text. The aridity index takes into account the values of precipitation, temperature and/or potential evapo-transpiration. The cases of rain-fed agriculture in the Sahara fall within the ‘hyper-arid’ (Ghat, Guezendou, Tassili, Tadrart Acacus), or ‘arid’ (Al Khiday, Moudjeria, and Air) class—well beyond the threshold for the supposed suitability of rain-fed agriculture. Map produced in QGis by the authors; baseline data (Aridity Index values) obtained from https://cgiarcsi.community/data/global-aridity-and-pet-database/ (accessed 10 July 2019) (Zomer et al. Reference Zomer, Bossio, Trabucco, Yuanjie, Gupta and Virendra2007, Reference Zomer, Trabucco, Bossio, van Straaten and Verchot2008).
Millet was grown in the nineteenth century in the Ahaggar Mountains, where rainfall varies between 0 and 100mm/year (Duveyrier Reference Duveyrier1864: 372). The Tuareg peoples inhabiting the valleys of the Ahaggar used a specific word (‘tawgest’) to designate non-irrigated plots of cultivated land (Nicolaisens & Nicolaisens Reference Nicolaisen and Nicolaisen1997: 251). Similarly, in the Air massif, where average precipitation is 120mm/year, Rodd (Reference Rodd1926: 133) observed that rain-fed cereals could be grown most years. The engagement of nomadic Tuareg groups with rain-fed cultivation is further confirmed by Nicolaisen and Nicolaisen (Reference Nicolaisen and Nicolaisen1997: 250–51), who observed rain-fed wheat and millet/sorghum fields in the Tassili n'Ajjer in the early 1950s. This last report is particularly surprising, given the very low (and mostly uneven) rainfall in this area, which ranges between 0 and 40mm/year. Cultivation has also been recorded in the Tadrart Acacus massif in south-west Libya (di Lernia et al. Reference di Lernia, N'siala, Zerboni, Mol and Sternberg2012) and around the city of Ghat (Bourbon del Monte di Santa Maria Reference Bourbon del Monte Santa Maria1912), where annual rainfall is less than 20mm. In the Tibesti Mountains of south-east Libya, close to the Guezendi area, rain-fed cereal plots were observed in areas with less than 50mm/year of precipitation (Desio Reference Desio1942). These examples clearly show that the cultivation of rain-fed crops complemented pastoral and foraged resources in the Sahara in the recent past.
The Al Khiday case study
In 2015–2016, we conducted ethnoarchaeological surveys in the area west of Al Khiday in central Sudan (Figure 2), with the aim of investigating the late prehistoric and historical landscape of the region, as well as the traditional ecological knowledge of contemporary populations. Today, the area is categorised as arid by both the Koppen-Geigen and CGIAR-CSI classifications (Zomer et al. Reference Zomer, Bossio, Trabucco, Yuanjie, Gupta and Virendra2007, Reference Zomer, Trabucco, Bossio, van Straaten and Verchot2008), with an aridity index of 0.0765, a yearly average rainfall of approximately 100mm (concentrated in July, August and partially September) and widespread sandy aridisols (i.e. soils typical of arid and hot environments, characterised by low organic content and colonised by drought- and salt-tolerant vegetation). Donatella Usai has been conducting archaeological investigations in this region since 2001 (Usai Reference Usai and Honegger2018). Upon joining her project in 2015, we noted the presence of many regularly spaced, rows of tufts of vegetation, located around 30km inland from the Nile, in an area devoid of farms, wells and permanent rivers (Figure 3). The following year, systematic fieldwork led to the identification of several rain-fed fields of pearl millet, located between 15 and 30km west of the White Nile.

Figure 2. GoogleEarth view of RFA15_01 and RFA15_02/04: a) the study area; b) RFA15_01, imagery taken in November 2014, with plough marks clearly visible; c) RFA15_02/04, imagery taken in September 2012 showing plots of cultivated lands; the crops growing form a pattern of coarsely parallel, vertical lines, clearly bounded by uncultivated land (one of the field's boundaries is indicated by the arrow) (GIS elaboration by the authors using QGIS).

Figure 3. View of recently harvested fields in the Al Khiday area in December 2016. The upper photograph shows a general view, where the margins of the fields are clearly marked in straight lines by the wild grass cover. The lower photograph shows a recently harvested plot, with tufts of millets still visible in regular rows (photographs by the authors).
Millets, and specifically pearl millet, are key crops in drylands due to their short growing season and their high productivity in arid, high-temperature conditions. As both the entire plant (before the grains mature) and the by-products from grain-processing can also be used as fodder, millets are well suited for mixed agro-pastoral systems. Pearl millet is a critical West Africa crop, and the Sahel zone south of the Sahara is an important area for its domestication (D'Andrea & Casey Reference D'Andrea and Casey2002; Winchell et al. Reference Winchell, Brass, Manzo, Beldados, Perna, Murphy, Stevens and Fuller2018). It is particularly suitable for rain-fed cultivation in hyper-arid areas, as it is well adapted to soil with low fertility and to high temperatures, and is able to grow with as little as 40mm of water per annum.
Desktop and field research
Our analysis of time-series imagery (GoogleEarth™), combined with rainfall data for the last 20 years, highlights that 70mm of rain in July/August is sufficient for pearl millet cultivation over extremely large expanses of land (up to 120ha per field system; Figure 2).
We conducted ethnographic interviews with local farmers, who revealed that the cultivated areas are divided into plots (Figure 3) owned by different families from neighbouring villages. After the late spring/early summer rains, the fields are hoed to clear wild grasses while the soil is still wet. Farmers then walk the fields along parallel lines, making holes and planting seeds approximately every metre. The sown fields are then left unattended until the harvest in September, at which time the farmers use iron sickles to cut the plants just above the crown. Grains are then threshed in the fields, and the by-products are left for the domestic livestock to graze. Although no productivity data are currently available for this type of arid or hyper-arid rain-fed agriculture, a preliminary evaluation—based on plant density and average single-plant seed production—gives a value of approximately 0.1t of millet per hectare. These figures are clearly much lower than the production in dry sub-humid and semi-arid regions of the world, where rain-fed agriculture yields vary between 0.5 and 2t/ha (Rockström & Falkenmark Reference Rockström and Falkenmark2015). In spite of the low yield, however, these farmers in central Sudan are able to produce twice the quantity of millet necessary for the families’ annual needs, leaving part of the crop to be sold at local markets after having set aside enough seed for the next planting season. What is surprising about these rain-fed fields in Sudan is that, in wetter years (with a rainfall of approximately 100mm, for example), a double cropping of millet, along with a cash crop, such as hibiscus (Hibiscus sp.) can be produced.
Phytolith analysis: preliminary results of a pilot project
In 2015, we conducted a pilot project at Al Khiday to check for the presence and preservation of phytoliths in modern deposits associated with rain-fed cultivation and to confirm the morphological ratios of phytoliths in relation to water availability, as highlighted by previous studies (Jenkins et al. Reference Jenkins, Jamjoum, Nuimat, Stafford, Nortcliff and Mithen2016). Phytoliths are silica bodies produced in plant tissues. Their inorganic nature means that they are far less affected by pre-and post-depositional issues than organic plant material, and hence, their preservation is ubiquitous (Piperno Reference Piperno2006). Two different field systems were sampled: two small ephemeral fields (RFA15_02 and RFA15_04; Figure 2) that had been cultivated the previous year, and an abandoned field (RFA15_01; Figure 2b) that had been cultivated for over 10 years, before being abandoned during the last 2–3 years. According to local informants, this latter field was mechanically ploughed but relied only on rain for water. Comparative samples were collected from inside and outside the furrows and holes used for planting (Table 1). Phytoliths were extracted and analysed according to standard laboratory protocols employed at the Laboratory for Environmental Archaeology at the Universitat Pompeu Fabra (Lancelotti et al. Reference Lancelotti, Ruiz-Pérez and García-Granero2017).
Table 1. Phytolith data; summary results of the phytolith analysis performed at the three sampling locations.

All the analysed samples yielded well-preserved phytoliths, with little or no evidence of chemical or mechanical damage, and several different morphotypes. Phytolith concentration is generally low and seems dependent on the intensity of use (i.e. both how long and also the different ways in which these fields were cultivated), as it is generally higher in RFA15_01 than in RFA15_02 and 04. The number of morphotypes identified, however, is virtually the same in both cases, thus indicating good representativeness of the original vegetation cover (Madella & Lancelotti Reference Madella and Lancelotti2012). Another notable aspect is the relationship between two of the main types recovered: bulliforms and trichomes. Samples from RFA15_02 and 04 yielded proportionally more bulliforms (average = 68) and fewer trichomes (average = 42) than those from RFA15_01 (Figure 4). These two morphotypes are considered indicative of environmental conditions in C4 grasses (Olsen et al. Reference Olsen, Caudle, Johnson, Baer and Maricle2013). Specifically, plants grown in mesic (more humid conditions) tend to have more bulliforms and fewer trichomes than plant grown in xeric (drier) conditions. Our results suggest that the plants from the less intensely cultivated area and farther from water sources (RFA15_02/04) grew in more humid conditions than those in the ploughed field. This may be a result of the techniques used for working the soil in preparation for sowing, with the intensive mechanical ploughing of RFA15_01 depleting the soil of moisture, whereas the soil in RFA15_02 and 04 conserved more humidity. These preliminary results confirm the viability of the methodology and encourage the further exploration of this line of research.

Figure 4. Phytolith analysis. Proportions of bulliforms (upper-left photograph) and trichomes (lower-right photograph) identified at the three sampling locations (photographs by the authors).
Exploring the past: a methodological proposal
The results of the pilot project show the potential of phytoliths as a proxy to study crop-water management practices in drylands. The methodology, however, needs to be further tested and improved in order to obtain significant results. We present a proposal on how this research should develop.
State of the art
Research on past crops in North Africa—especially for the drier areas—is still very limited. During the Early and Middle Holocene (10 000–5000 BC), patchy, savannah-like vegetation supported small communities of hunter-gatherers that exploited a range of wild millet-type grasses (Fuller Reference Fuller and Shillington2005), including attempts at opportunistic cultivation without domestication (Mercuri et al. Reference Mercuri, Fornaciari, Gallinaro, Vanin and di Lernia2018). These populations had certainly adopted livestock by 6000–5000 BC (Marshall & Hildebrand Reference Marshall and Hildebrand2002; Fuller Reference Fuller and Shillington2005). The shift from the more humid ‘Green Sahara’ (Holocene climatic optimum) to the present-day conditions occurred by the end of the Middle Holocene, and it is generally marked by relevant socio-economic transformations (Gasse Reference Gasse2000; Kuper & Kröpelin Reference Kuper and Kröpelin2006). After 3500 BC, when the climate conditions became drier, some of the nomadic pastoral groups seem to have adopted cultivation in selected Saharan locations (Cremaschi & Zerboni Reference Cremaschi and Zerboni2009). Yet, the role that rain-fed agriculture may have played in such a critical transition has not been explored, and very few data are available on the earliest agricultural experiments in the Sahara. Past studies have emphasised the contemporaneous adoption of more drought-tolerant livestock, such as the dromedary and goat, in parallel with the progressive abandonment of cattle (Clarke et al. Reference Clarke2016).
Research on rain-fed practices in the Sahara and an understanding of their chronological depth is urgently needed in order to address the role of cultivation in the resilience of human societies living in unfavourable climatic settings. Notwithstanding the importance that rain-fed agriculture could have had in the Sahara and North Africa during the Late Holocene, the practice has only occasionally formed part of the current discourse on strategies of human adaptation. This is probably due to the challenges in detecting direct evidence for rain-fed cultivation in the archaeological record. The identification of ancient fields, for example, is extremely difficult, even using techniques such as geoarchaeology or molecular footprints (Motuzaite-Matuzeviciute et al. Reference Motuzaite-Matuzeviciute, Jacob, Telizhenko and Jones2016), and fields are usually inferred from the presence of related technology, such as ploughs and terracing. Furthermore, the different types of water management are also difficult to discern, and currently there are few proxies able to identify and differentiate such practices clearly. Determining water availability directly from archaeobotanical material offers a possible solution.
Previous efforts to address past water-management practices have explored charred macro-botanical remains (Jenkins et al. Reference Jenkins, Jamjoum, Nuimat, Stafford, Nortcliff and Mithen2016), have analysed weed assemblages and performed isotopic studies of δ13C. All of these analyses have demonstrated good potential for assessing water-management practices in C3 plants, such as trees, legumes and winter cereals (Styring et al. Reference Styring, Ater, Hmimsa, Fraser, Miller, Neef, Pearson and Bogaard2016). Approaches exclusively based on charred remains, however, can prove problematic in drylands, where they are often poorly preserved. Consequently, the analysis of plant microfossils, such as phytoliths, has been explored as an alternative (Madella et al. Reference Madella, Jones, Echlin, Powers-Jones and Moore2009; Jenkins et al. Reference Jenkins, Jamjoum, Nuimat, Stafford, Nortcliff and Mithen2016). Morphology—assessed through indices based on morphotype proportions—and isotopic analyses have been explored to identify different water regimes from archaeobotanical samples (McClaran & Umlauf Reference McClaran and Umlauf2000; Webb & Longstaffe Reference Webb and Longstaffe2003; Madella et al. Reference Madella, Jones, Echlin, Powers-Jones and Moore2009; Hodson Reference Hodson2016; Jenkins et al. Reference Jenkins, Jamjoum, Nuimat, Stafford, Nortcliff and Mithen2016).
RAINDROPS: an innovative approach
RAINDROPS aims to develop an innovative and reliable methodology for the identification of crop-water availability and management based on archaeobotanical remains. Highly controlled data on phytolith ratios and carbon, oxygen and silicon isotopes from macro- and micro-remains from experimental fields are being validated with ethnographic evidence, before being applied to selected key archaeological case studies in Africa and Asia.
Crops grown in experimental fields at the International Crop Research Institute for the Semi-Arid Tropics—under rigorously controlled conditions—form the basis of genotypic variation in phytolith production and isotopic fractionation for sorghum, finger millet (Eleusine coracana Gaertn.) and pearl millet landraces. To strengthen further the comparison between the experimental isotopic data and archaeological samples, the effect of charring on isotopic ratios is also being assessed.
Phytolith concentration and ratios between genetically vs environmentally controlled morphotypes analysed from experimentally grown plants are being tested to confirm that the observed correlation with plant-water availability identified in C3 plants holds true for C4 species. Simultaneously, oxygen and silicon isotopes are assessed for their potential use as proxies for evaluating crop-water availability and soil conditions. Pilot projects have anticipated the possible benefits of such an approach and have laid a strong foundation for the analysis of these oxygen and silicon isotopes in phytoliths (Chapligin et al. Reference Chapligin, Leng, Webb, Alexandre, Dodd, Ijiri and Longstaffe2011). These studies indicate that the degree of silica 18O enrichment in leaves can be related to water loss through transpiration and relative humidity, and that silicon isotopes are sensitive to environmental conditions and changes (Webb & Longstaffe Reference Webb and Longstaffe2003; Opfergelt et al. Reference Opfergelt, Cardinal, Henriet, André and Delvaux2006; Ding et al. Reference Ding, Zhou, Wan, Chen, Wang and Zhang2008; Leng et al. Reference Leng, Swann, Hodson, Tyler, Patwardhan and Sloane2009).
Ethnographic data and traditional ecological knowledge
The same set of analyses is being performed on crops grown locally using traditional methods (no chemical fertilisers and pesticides) in three study areas: Sudan, Ethiopia and Pakistan. At the same time, structured and unstructured interviews are being conducted with a number of local farmers—according to established ethnographic protocols—specifically, to assess water-management practices, as well as to collect traditional ecological knowledge on cultivation systems. These interviews target quantifiable data on cultivation methods, such as tilling, weeding, manuring and ploughing methods, as well as information on decision-making related to where and when cultivation is carried out and how these processes are affected by climate variability.
The three case studies (Sudan, Ethiopia and Pakistan) were selected because of their geographical and environmental locations, which represent biophysical hotspot areas in terms of their significance in the long-term human adaptation to drylands, as well as for the presence of preliminary results—such as for Al Khiday, with the ethnoarchaeological work presented here. Furthermore, each site is located in one of the three ecological areas that broadly define the concept of drylands (United Nations Environment Programme 1997): arid to hyper-arid (Al Khiday, Sudan), dry sub-humid (Mezber, Ethiopia) and semi-arid (Harappa, Pakistan), thereby covering the geo-climatic variability of drylands. Water-management practices at these sites have been hypothesised on the basis of local geographic or geomorphological features, economy and degree of social organisation (Giosan et al. Reference Giosan2012; C. D'Andrea pers. comm.), although no test for these hypotheses has ever been provided. All of the sites are archaeologically well studied, with abundant palaeoenvironmental and palaeoclimatic evidence and long occupation sequences—factors that will allow for the study of the long-term dynamics of human-environment interaction (Terwilliger et al. Reference Terwilliger, Eshetu, Alexandre, Huang, Umer and Gebru2011; Giosan et al. Reference Giosan2012; D'Andrea et al. Reference D'Andrea, Fahmy, Perry, Richards, Darcus, Toffolo and El Shafaey2015; Williams et al. Reference Williams, Usai, Salvatori, Williams, Zerboni, Maritan and Linseele2015; Iacumin et al. Reference Iacumin, Matteo, Usai, Salvadori and Venturelli2016). Lastly, the three sites are located in areas where traditional agriculture is still practised, and where a rich source of traditional ecological knowledge exists. RAINDROPS is both recording and utilising this information as part of its data analyses.
Concluding remarks
Desertification has been internationally recognised as one of the main challenges of sustainable development, and in 1994, the United Nations Convention to Combat Desertification (UNCCD) was created. One of the aims of the UNCCD is to put science, technology and traditional knowledge at the forefront in mitigating desertification. In this perspective, environmental archaeology and ethnoarchaeology can play a fundamental role in understanding the dynamics of long-term strategies of human adaptation and resilience in drylands. Archaeologically, considerable research has been devoted to the study of the origins of agriculture—focusing especially on the Near East—and crops such as wheat and barley that provide the foundation for modern agricultural systems in the Old World. It is clear, however, that past human groups also experimented with a diverse assemblage of crops, some of which, such as millets, are well adapted to areas with water constraints. Such crops constitute one of the most important sources for future biodiverse and sustainable agriculture in many parts of the world. Furthermore, drylands agriculture is becoming highly relevant in development studies, due to the impact of desertification on food security for a great proportion of the human population. Our current work in Sudan highlights the importance of rain-fed cultivation in drylands, and our approach will provide the research community with an innovative and reliable methodology for understanding past water-management practices for C4 crops. This will extend the chronology of traditional ecological knowledge in key low-precipitation areas, contributing long-term data to explore resilience and to improve model-building for future policies.
Acknowledgements
We would like to thank all those who helped during fieldwork, particularly Mungida Khaled Magzoub and Ahmed Altayeb, as well as the Director General of the National Corporation of Antiquities and Museums (Sudan), Abdelrhman Ali Mohamed, for granting the licence. Funding is provided by the Italian Ministry for Foreign Affairs (MAE-DGSP VI). RAINDROPS has received funding from the European Union's Horizon 2020 research and innovation programme (ERC-Stg-2017) under grant agreement 759800. Additional funding for fieldwork was provided by the University of Milano. This work is also part of the PAGES LandCover6k effort and the INQUA International Focus Group HoLa (Holocene Global Landuse). Fieldwork in Al Khiday has been conducted in the area licensed to Donatella Usai of the Center for Sudanese and Sub-Saharan Studies. The licence includes permits for exporting sediments for analysis, for which clearance has also been obtained by the National Corporation for Antiquities and Museums in Khartoum. Oral consent has been obtained from the people interviewed during ethnoarchaeological surveys. No personal or sensitive data have been collected during, or stored after, these interviews. Universitat Pompeu Fabra health and safety measures have been followed during both field and laboratory work. C.L., S.B. and M.M. are members of CaSEs (UPF), an ‘Excellence Research Group’ of the Catalan Agency for Research (AGAUR SGR-1417 and SGR-0212) and an associated unit (unidad asociada) of the Institució Milà i Fontanals of the Spanish National Research Council (IMF-CSIC).