Introduction
Invasive meningococcal disease (IMD) has high morbidity and mortality [Reference Borrow1, Reference Olbrich2], with the incidence of IMD varying globally, from 0.01 to 0.02 cases per 100 000 in Mexico to 2–3.6 cases per 100 000 in Morocco [Reference Acevedo3]. While antibiotic treatment can be used for IMD, there is often rapid disease progression despite appropriate medical care. Mortality due to IMD remains high, with a fatality rate of 10–15%, and a high morbidity rate persists with severe complications and lifelong sequelae [Reference Pace and Pollard4]. With early diagnosis and rapid, effective, treatment challenging, prevention of disease plays an important role in reducing IMD.
In both Europe and the USA incidence of IMD is highest in infants <1 year old, followed by those 1–4 years old and those 15–24 or 16–23 years old [5, 6]. The majority of IMD is caused by six serogroups: A, B, C, W, X and Y. The relative contribution to the disease of each serogroup varies geographically [Reference Borrow1, Reference Acevedo3, Reference Vespa Presa7, 8], and over time, with decreases seen in IMD caused by serogroup C due to vaccination, and more recent increases in cases due to serogroups W and Y in parts of Europe and South America without routine vaccination for these serogroups [Reference Borrow1, Reference Acevedo3, Reference Tsang9–Reference Martin13].
Routine paediatric vaccination schedules for infants, toddlers, children and adolescents vary between countries [Reference Acevedo3, Reference Presa14], with many childhood vaccination schedules combining several vaccinations in a single visit to reduce the number of visits to a healthcare provider and increase compliance. Consequently, it is important to assess the immunogenicity and safety when vaccines are administered together, particularly as previous evidence has suggested that there may be an interaction between the vaccine protein conjugates and other vaccine antigens, which may alter the immunogenicity or safety of coadministered vaccines [Reference Findlow and Borrow15, Reference Bröker16].
Licensed protein-conjugated meningococcal vaccines fall into two groups, monovalent vaccines against single serogroups, such as vaccines against serogroups A and C, or those against multiple serogroups such as the quadrivalent vaccines against serogroups A, C, W and Y. MenACYW-TT is a tetanus toxoid conjugated vaccine against meningococcal serogroups A, C, W and Y and has been shown to be immunogenic and well-tolerated when administered as a single dose in studies conducted in toddlers, children, adolescents and adults (including those >65 years) [Reference Anez17–Reference van der Vliet23]. Coadministration of MenACYW-TT with tetanus, diphtheria, acellular pertussis (Tdap) and human papillomavirus (HPV4) vaccines in meningococcal vaccine-naïve adolescents demonstrated comparable safety and immunogenicity profiles of the MenACYW-TT and coadministered vaccines [Reference Chang24]. This current study assessed the immunogenicity and safety of MenACYW-TT when coadministered with routine paediatric vaccines in healthy meningococcal vaccine-naïve toddlers aged 12–23 months.
Methods
Study design and participants
This was a phase III, open-label, randomised, active-controlled, multi-centre study in healthy meningococcal vaccine-naïve toddlers aged 12–23 months to evaluate the immunogenicity and safety of MenACYW-TT when coadministered with routinely used paediatric vaccines in South Korea, Thailand, Mexico and the Russian Federation, according to the local immunisation programmes (NCT03205371). The choice of the countries was driven by the country-specific immunisation recommendations, [25] schedules and the published vaccination coverage rates. In South Korea and Thailand, MenACYW-TT was coadministered with a measles, mumps and rubella (MMR) vaccine and a varicella (V) vaccine. In Mexico, MenACYW-TT was coadministered with a 6-in-1 combination vaccine against diphtheria, tetanus, pertussis, polio, hepatitis B and Haemophilus influenzae type b (DTaP-IPV-HepB-Hib). In Russia, MenACYW-TT was coadministered with a pneumococcal conjugate vaccine (PCV13). This study was conducted between 7 November 2016 and 19 July 2018.
Participants were eligible for inclusion if they had received all recommended standard-of-care vaccines according to their age and a signed informed consent form was completed by their parent/guardian. Exclusion criteria included: receipt of any vaccine in the 4 weeks prior to the first visit, planned receipt of a vaccine during the study except for influenza vaccine, inclusion in another trial in the 4 weeks preceding the first visit, previous receipt of any meningococcal vaccine, history of meningococcal infection, high risk of meningococcal infection and personal history of Guillain−Barre syndrome (GBS) or Arthus-like reaction. Additionally, participants were excluded from the PCV13 groups from Russia if they had received the third dose of PCV13 (participants should have only received two PCV13 doses in the first year of life); known history of seizures or encephalopathy occurring within 7 days of vaccination with a pertussis-containing vaccine, or previous vaccination with DTaP-IPV-HB-Hib or DTaP containing vaccine at 12–23 months in Mexico; or known history of seizures, or previous vaccination with MMR or varicella at or before 12–23 months in South Korea or Thailand.
In South Korea, Thailand and Mexico, healthy, meningococcal vaccine-naïve toddlers aged 12–23 months were randomised 2:1:1 to receive either MenACYW-TT and coadministered vaccine(s), MenACYW-TT alone or coadministered vaccine(s) alone. In Russia, to ensure compliance with the local immunisation recommendations for PCV13 vaccines, healthy meningococcal vaccine-naïve toddlers aged 12–14 months and 16–23 months were assigned to the MenACYW-TT alone group with a balanced proportion between the age groups; healthy toddlers aged 15–23 months who had not received the third dose of PCV13 were randomised in a 2:1 ratio to MenACYW-TT and PCV13 or PCV13 alone. Due to the nature of the study design, the study was open-label; however, the laboratory technicians responsible for the serological testing remained blinded to the participants' allocation throughout the study.
The MenACYW-TT vaccine (Sanofi Pasteur, Swiftwater, PA, USA) was provided as a 0.5 ml dose containing 10 μg of each serogroup (A, C, W and Y) and ~55 μg of tetanus toxoid protein carrier. The coadministered vaccines were M-M-R® II (Merck & Co, Whitehouse Station, NJ, USA), VARIVAX® (Merck, Sharp & Dohme, Haarlem, the Netherlands), Hexaxim® (Sanofi Pasteur, Marcy l'Etoile, France) and Prevenar 13® (Pfizer Ireland Pharmaceuticals, Ireland). These co-administered vaccines are licensed globally in multiple countries, occasionally with different brand names.
Participants provided blood samples for immunogenicity assessment at baseline (pre-vaccination) and Day 30 (30–44 days) after vaccination. Upon completion of the study, participants received the remainder of the recommended vaccines for their age as per the respective National Immunisation Programmes for each country. Additionally, after completion of all study-related vaccinations and visits, the study participants who had been randomised to the concomitant vaccines only group were offered the locally licensed quadrivalent meningococcal conjugate vaccines at the respective study clinics. This was done to ensure that these subjects also have an equal opportunity to receive protection against IMD.
The conduct of this trial was compliant with the standards established by the Declaration of Helsinki and the International Conference on Harmonization (ICH) guidelines for good clinical practice (GCP) as well as with all local and/or national regulations and directives. The study protocol was reviewed and approved by Independent Ethics Committees or Institutional Review Boards at each study site.
Immunogenicity
Functional antibodies to the meningococcal antigens were measured in the blood samples at baseline and at Day 30 by serum bactericidal antibody assay using human complement (hSBA) or baby rabbit complement (rSBA) and were conducted at Global Clinical Immunology (GCI; Sanofi Pasteur, Swiftwater, PA, USA) or Public Health England (PHE, Health Protection Agency, Manchester UK), respectively, as described previously [Reference Maslanka26, Reference Pina27]. Polio, hepatitis B and Hib (anti-PRP) were assessed by GCI (Sanofi Pasteur) as described previously [Reference Tregnaghi, Zambrano and Santos-Lima28]; anti-diphtheria, tetanus, pertussis and pneumococcal antibodies were assessed by GCI (Sanofi Pasteur) (described in Supplementary Methods); anti-measles, mumps, rubella and varicella antibody levels were assessed by PPD Laboratories (Richmond, VA, USA), as described previously [Reference Shehab, Brunell and Cobb29–Reference Provost33].
The primary endpoints of this study were the determination of immune response against all four meningococcal serogroups as measured by hSBA before vaccination and at Day 30 after vaccination, in terms of the proportion of participants with hSBA titres ≥1:8 (seroprotection), the geometric mean titres (GMTs) of antibodies and the proportion of participants with hSBA seroresponse at Day 30 (post-vaccination titres ≥1:16 in those with pre-vaccination titres <1:8, or post-vaccination titres ≥4-fold greater than the pre-vaccination titre in participants with pre-vaccination titres ≥1:8). Secondary endpoints were assessment of immune responses to the antigens of the coadministered vaccines before vaccination and at Day 30 after vaccination, in terms of GMTs or geometric mean antibody concentrations (GMCs), and the proportion of participants with seroprotective levels at Day 30 (detailed in Supplementary Methods). Additionally, antibody titres against meningococcal serogroups A, C, W and Y measured by rSBA before and 30 days (up to 44 days) after vaccination with MenACYW conjugate vaccine in a subset of participants (100 participants randomly assigned from each of the MenACYW-TT and coadministered vaccine(s) groups (South Korea, Mexico and Russia only) and 50 randomly assigned participants from each of the MenACYW-TT alone groups (South Korea, Mexico and Russia only)) were also determined.
Baselines titres (D0) were evaluated for antibodies to tetanus, pertussis, measles, mumps, rubella, varicella antigens and all serotypes contained in the pneumococcal vaccine in the respective study groups. Since established correlates of protection exist for diphtheria, polio, hepatitis B and Hib, D0 antibodies were not evaluated for these four antigens. Post-vaccination (D30) titres were evaluated for all antigens in the paediatric vaccines administered concomitantly in the study across all countries.
Safety
Participants were observed for 30 min after vaccination to assess the occurrence of any immediate unsolicited systemic adverse events (AEs). Parents/legally acceptable representatives were provided with diary cards, digital thermometers and flexible rulers to record daily body temperature, solicited injection site and systemic reactions and other unsolicited AEs up to seven days after vaccination. Unsolicited non-serious AEs were recorded up to Day 30; serious AEs (SAEs) were recorded throughout the study. Adverse reactions (ARs) were any unintended responses to the vaccination where a causal relationship was a possibility.
Statistical analysis
This was a descriptive study and, as such, there were no formal hypotheses, however the analyses were conducted following a formal Statistical Analysis Plan. Categorical data are presented as frequency counts and percentages with 95% confidence intervals (CIs). The 95% CIs of point estimates were calculated using the normal approximation for quantitative data and the exact binomial distribution (Clopper–Pearson method) for percentages. For GMTs or GMCs, 95% CIs of point estimates were calculated using normal approximation assuming they are log-normally distributed.
Descriptive statistics were presented by the group for Mexico and Russia and by the pooled group for participants included in South Korea and Thailand. All immunogenicity analyses were performed on the per-protocol analysis set (PPAS) which was comprised of all participants who received at least one dose of the study vaccine, had a valid post-vaccination blood sample and had no protocol deviations. The safety data are presented for the safety analysis set (SafAS), which comprised all participants who received at least one dose of study vaccine and had safety data available.
Results
A total of 1183 participants were enrolled in this study; 213 in South Korea, 400 in Mexico, 400 in Russia and 170 in Thailand. Participant flow through the study is shown in Supplementary Figure S1. The baseline characteristics were well balanced between the treatment groups (Table 1).
Table 1. Baseline characteristics (all randomised participants)

DTaP-IPV-HepB-Hib, diphtheria, tetanus, pertussis, polio, hepatitis B and Haemophilus influenzae type b vaccine; MMR, measles, mumps and rubella vaccine; N, number of participants; n, number of participants fulfilling the criteria listed; PCV13, pneumococcal conjugate vaccine; s.d., standard deviation; V, varicella vaccine.
a Variation in age between the countries due to randomisation protocol in each country.
MenACYW-TT concomitant with MMR and varicella vaccines (Thailand and South Korea)
In both the MenACYW-TT coadministered with MMR and V and MenACYW-TT groups the proportion of participants with seroprotection to each meningococcal serogroup was comparable between the groups at Day 30 (Fig. 1a). hSBA GMTs for each meningococcal serogroup increased from baseline to Day 30 and were comparable between the groups at Day 30 (Supplementary Table S1), as was hSBA vaccine seroresponse to each serogroup (Supplemental Fig. S2). The proportions of participants with rSBA titres ≥1:8 and ≥1:128 to each meningococcal serogroup were also comparable between the vaccine groups (Supplementary Table S2).
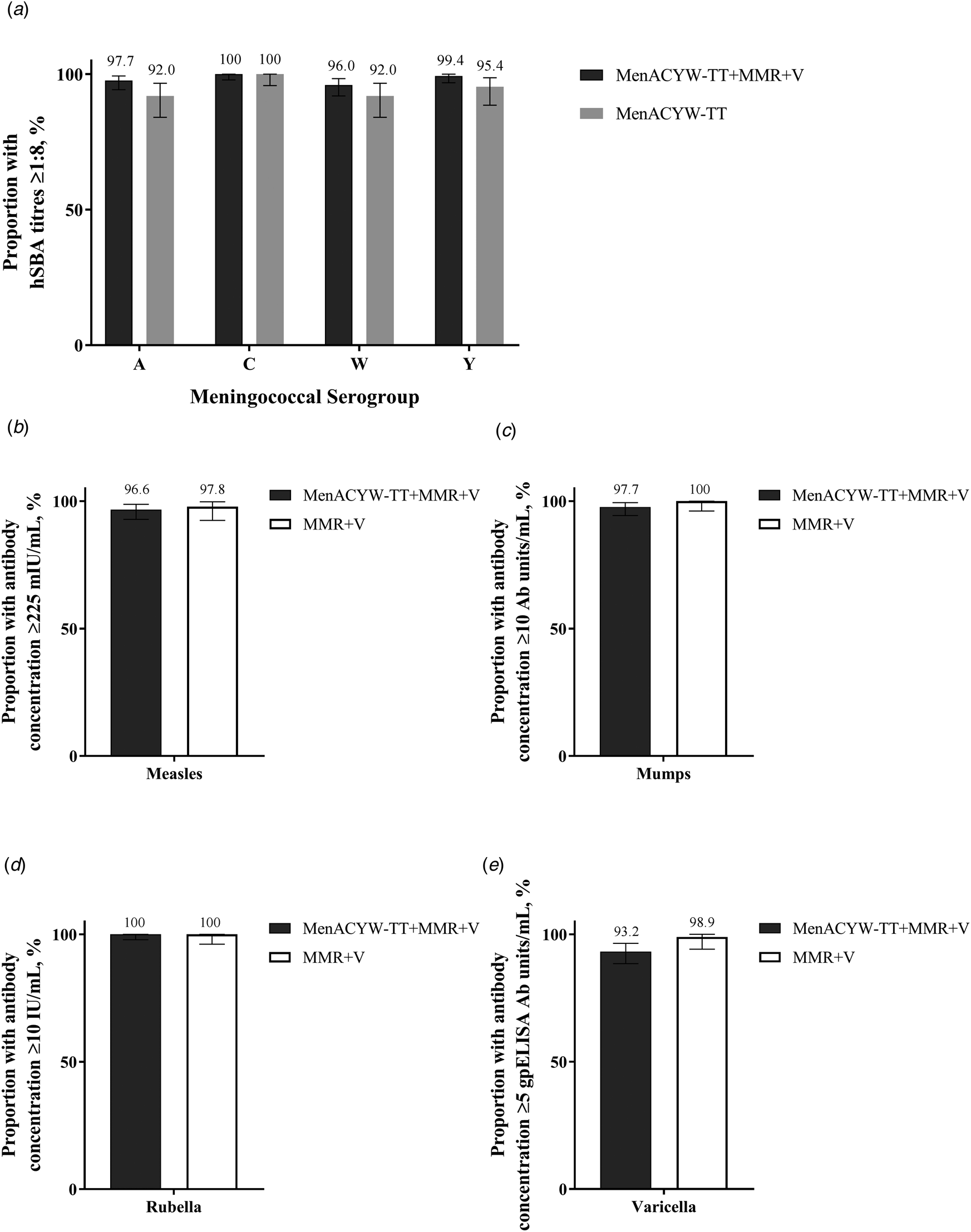
Fig. 1. Proportion of participants with (a) hSBA meningococcal titres ≥1:8, (b) anti-measles antibody concentrations ≥225 mIU/ml, (c) anti-mumps antibody concentrations ≥10 mumps antibody units/ml, (d) anti-rubella concentrations ≥10 IU/ml and (e) anti-varicella antibody concentrations ≥5 glycoprotein ELISA antibody units/ml at Day 30 in participants randomised to MenACYW-TT + MMR + V, MenACYW-TT and MMR + V (PPAS). ELISA, enzyme-linked immunosorbent assay; hSBA, serum bactericidal antibody assay using human complement; MMR, measles, mumps and rubella vaccine; PPAS, per-protocol analysis set; V, varicella vaccine.
At baseline, the GMCs for anti-measles, mumps, rubella and varicella antibodies were comparable between the MenACYW-TT coadministered with MMR and V and the MMR and V groups, and by Day 30 the GMCs increased in both groups and were comparable (Supplementary Table S3). The response rates for the MMR and V vaccine antigens at Day 30 were high and comparable between the vaccine groups (Fig. 1b–e).
An overview of the safety data is shown in Table 2. The frequencies of participants who reported at least one solicited reaction were 68.3% (129/189) in the MenACYW-TT coadministered with MMR and V group, 76.6% (72/94) in the MenACYW-TT group and 63.2% (60/95) in the MMR and V group. Most solicited reactions were Grade 1 or Grade 2 in intensity. The most commonly reported solicited injection site reaction after vaccination with MenACYW-TT, MMR or V was injection site tenderness (at the MenACYW-TT site: 30.2% (57/189) in the MenACYW-TT coadministered with MMR and V group and 34.0% (32/94) in the MenACYW-TT group; at the MMR site: 20.1% (38/189) in the MenACYW-TT coadministered with MMR and V group and 29.5% (28/95) in the MMR and V group; and at the V site: 21.2% (40/189) in the MenACYW-TT coadministered with MMR and V group and 26.3% (25/95) in the MMR and V group). The most common solicited systemic reaction was irritability (23.8% (45/189) in the MenACYW-TT coadministered with MMR and V group, 24.5% (23/94) in the MenACYW-TT group and 26.3% (25/95) in the MMR and V group).
Table 2. Safety overview in participants randomised to MenACYW-TT + MMR + V and MenACYW-TT (SafAS)

AE, adverse event; AR, adverse reaction; CI, confidence interval; M, number of participants with data available for the relevant endpoint; MMR, measles, mumps and rubella vaccine; n, number of participants fulfilling the relevant endpoint; SAE, serious adverse event; SafAS, safety analysis set; V, varicella vaccine.
There were no immediate AEs and no participant discontinued due to an AE or AR. Thirteen participants experienced at least one SAE during the study period (6/189 (3.2%) in MenACYW-TT coadministered with MMR and V, 5/94 (5.3%) in MenACYW-TT, and 2/95 (2.1%) in MMR and V groups); none were considered to be related to vaccination by the investigator or sponsor, and none led to study discontinuation. No deaths were reported.
MenACYW-TT concomitant with DTaP-IPV-hepB-Hib vaccine (Mexico)
For both MenACYW-TT coadministered with DTaP-IPV-HepB-Hib and MenACYW-TT groups, the proportion of participants with seroprotection to each meningococcal serogroup increased from baseline and was comparable between the groups at Day 30 (Fig. 2a). hSBA GMTs increased from baseline to Day 30 and were comparable between the groups (Supplementary Table S4), as was the proportion with hSBA seroresponse at Day 30 (Supplementary Fig. S3). The proportion of participants with rSBA titres ≥1:8 and ≥1:128 at Day 30 was 100% for both study groups for serogroups A, C and W, and >96% for Y (Supplementary Table S5).

Fig. 2. Proportion of participants with (a) hSBA meningococcal titres ≥1:8, (b) anti-tetanus antibody concentrations ≥0.1 and 1.0 IU/ml, (c) anti-diphtheria antibody concentrations ≥0.1 and 1.0 IU/ml, (d) anti-pertussis PT and FHA vaccine response*, (e) anti-polio 1, 2 and 3 antibody titres ≥1:8, (f) anti-Hep B antibody concentrations ≥10 and 100 mIU/ml, and (g) anti-PRP antibody concentrations ≥0.15 and 1.0 μg/ml at Day 30 in participants randomised to MenACYW-TT + DTaP-IPV-HepB-Hib, MenACYW-TT and DTaP-IPV-HepB-Hib (PPAS). DTaP-IPV-HepB-Hib, diphtheria, tetanus, pertussis, polio, hepatitis B and Haemophilus influenzae type b vaccine; FHA, filamentous haemagglutinin; hSBA, serum bactericidal antibody assay using human complement; PPAS, per-protocol analysis set; PRP, polyribosyl-ribitol phosphate; PT, pertussis toxoid.
At baseline, the GMCs for anti-tetanus and pertussis vaccine components were comparable between the MenACYW-TT coadministered with DTaP-IPV-HepB-Hib and DTaP-IPV-HepB-Hib groups and, by Day 30, the GMCs had increased for both tetanus and pertussis antigens and were comparable; Day 30 GMCs for anti-diphtheria, Hep B and PRP antibodies and GMTs for anti-polio antibodies were comparable between the vaccine groups (Supplementary Table S6). The proportions of participants with seroprotection to tetanus, diphtheria, polio, Hep B and PRP were ≥98% for both vaccine groups at Day 30, and ≥88% for the vaccine response to pertussis components (Fig. 2b–g).
An overview of the safety data is shown in Table 3. The frequencies of participants who reported at least one solicited reaction were 69.6% (133/191) in the MenACYW-TT coadministered with DTaP-IPV-HepB-Hib group, 58.2% (57/98) in the MenACYW-TT group and 78.9% (75/95) in the DTaP-IPV-HepB-Hib group. Most solicited reactions were Grades 1 and 2 in intensity. The most commonly reported solicited injection site reaction after vaccination with MenACYW-TT or DTaP-IPV-HepB-Hib was injection site tenderness (at the MenACYW-TT site: 35.6% (68/191) in the MenACYW-TT coadministered with DTaP-IPV-HepB-Hib group and 27.6% (27/98) in the MenACYW-TT group; and at the DTaP-IPV-HepB-Hib site: 41.9% (80/191) in the MenACYW-TT wcoadministered with DTaP-IPV-HepB-Hib group and 56.8% (54/95) in the DTaP-IPV-HepB-Hib group). The most commonly reported solicited systemic reaction was irritability (33.5% (64/191) in the MenACYW-TT coadministered with DTaP-IPV-HepB-Hib group, 34.7% (34/98) in the MenACYW-TT group and 34.7% (33/95) in the DTaP-IPV-HepB-Hib group).
Table 3. Safety overview in participants randomised to MenACYW-TT + DTaP-IPV-HepB-Hib, MenACYW-TT and DTaP-IPV-HepB-Hib (SafAS)

AE, adverse event; AR, adverse reaction; CI, confidence interval; DTaP-IPV-HepB-Hib, diphtheria, tetanus, pertussis, polio, hepatitis B and Haemophilus influenzae type b vaccine; M, number of participants with data available for the relevant endpoint; n, number of participants fulfilling the relevant endpoint; SAE, serious adverse event; SafAS, safety analysis set.
There were no immediate AEs and no participants discontinued due to an AR, one participant discontinued due to an AE in the MenACYW-TT group. One participant experienced at least one SAE during the study period in the DTaP-IPV-HepB-Hib group which was not considered related to the vaccine by the investigator or sponsor, and did not lead to study discontinuation. No deaths were reported in any study group.
MenACYW-TT concomitant with PCV13 vaccine (Russia)
For both MenACYW-TT coadministered with PCV13 and MenACYW-TT groups, the proportion of participants with seroprotection to each meningococcal serogroup was comparable between the groups at Day 30 (Fig. 3a). hSBA GMTs for each meningococcal serogroup increased from baseline to Day 30 and were comparable between the groups for serogroups C, W and Y, with higher titres to serogroup A in the MenACYW-TT group than the MenACYW-TT coadministered with PCV13 group (Supplementary Table S7). The hSBA vaccine seroresponse at Day 30 was also comparable between the groups (Supplementary Fig. S4). rSBA titres ≥1:8 and ≥1:128 to each meningococcal serogroup were comparable between the vaccine groups at Day 30 (Supplementary Table S8).
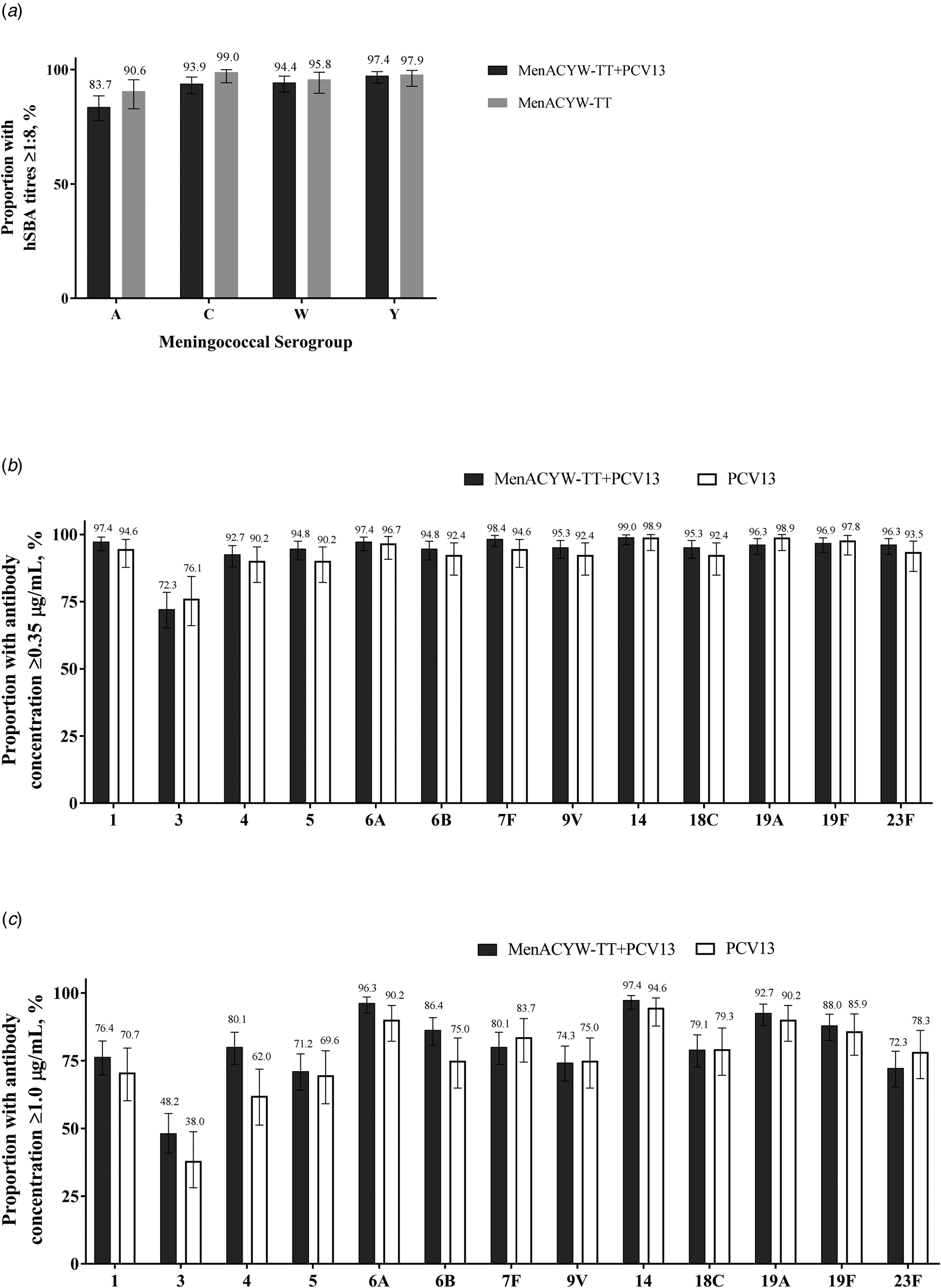
Fig. 3. Proportion of participants with (a) hSBA meningococcal titres ≥1:8, and anti-pneumococcal antibody concentrations (b) ≥0.35 μg/ml and (c) ≥1.0 μg/ml to serotypes 1, 3, 4, 5, 6A, 6B, 7F, 9V, 14, 18C, 19A, 19F and 23F at Day 30 in participants randomised to MenACYW-TT + PCV13, MenACYW-TT and PCV13 (PPAS). hSBA, serum bactericidal antibody assay using human complement; PCV13, pneumococcal conjugate vaccine; PPAS, per-protocol analysis set.
At baseline the GMCs for all the 13 PCV serotypes were comparable between the MenACYW-TT coadministered with PCV13 and PCV13 groups; by Day 30 the GMCs increased in both groups and were comparable (Supplementary Table S9). The proportion with antibody concentrations ≥0.35 μg/ml to the PCV serotypes at Day 30 was ≥90% for all components, except for serotype 3 (>72%) (Fig. 3b). The percentage of participants with anti-pneumococcal antibody concentrations ≥1.0 μg/ml ranged from 48.2% (serotype 3) to 97.4% (serotype 14) in the MenACYW-TT coadministered with PCV13 group and from 38.0% (serotype 3) to 94.6% (serotype 14) in the PCV13 group (Fig. 3c).
An overview of the safety data is shown in Table 4. The frequencies of participants who reported at least one solicited reaction were 36.5% (73/200) in the MenACYW-TT coadministered with PCV13 group, 28.0% (28/100) in the MenACYW-TT group and 17.2% (17/99) in the PCV13 group. Most solicited reactions were Grades 1 and 2 in intensity. The most commonly reported solicited injection site reaction after MenACYW-TT or PCV13 injection was injection site erythema (at the MenACYW-TT site: 21.5% (43/200) in the MenACYW-TT coadministered with PCV13 group and 17.0% (17/100) in the MenACYW-TT group; and at the PCV13 site: 24.0% (48/200) in the MenACYW-TT coadministered with PCV13 group and 8.1% (8/99) in the PCV13 group). The most commonly reported solicited systemic reaction was irritability (13.0% (26/200) in the MenACYW-TT coadministered with PCV13 group, 16.0% (16/100) in the MenACYW-TT group, and 9.1% (9/99) in the PCV13 group).
Table 4. Safety overview in participants randomised to MenACYW-TT + PCV13, MenACYW-TT and PCV13 (SafAS)

AE, adverse event; AR, adverse reaction; CI, confidence interval; M, number of participants with data available for the relevant endpoint; n, number of participants fulfilling the relevant endpoint; PCV13, pneumococcal conjugate vaccine; SAE, serious adverse event; SafAS, safety analysis set.
There were no immediate AEs and no participants discontinued due to an AE or AR and no participant experienced an SAE. No deaths were reported.
Discussion
When coadministered with common paediatric vaccines in healthy toddlers, there was no clinically relevant effect on the immunogenicity or safety of MenACYW-TT or the coadministered vaccines. This reflects the previous experience of MenACYW-TT when coadministered with Tdap and HPV4 vaccines in healthy adolescents, which similarly found comparable immunogenicity and safety profiles of MenACYW-TT and the coadministered vaccines [Reference Chang24]. The immune responses observed in the MenACYW-TT only groups from each of the four countries are comparable with the immune responses seen in another study of MenACYW-TT in toddlers (NCT02955797) [Reference van der Vliet23]. This suggests that the MenACYW-TT immune response shows no evidence of being influenced by geography or variable baseline seropositivity and the results can be extrapolated to other countries with similar vaccine schedules. Additionally, since the licensed paediatric vaccines administered in this study are broadly licensed in many countries across the world (although sometimes with varying brand names), the data generated in this study has relevance globally; not just in the four countries where the study was conducted.
It is important to assess the coadministration of paediatric vaccines as they are frequently administered together as part of childhood immunisation schedules to reduce the number of visits, and improve compliance with the full immunisation schedule. Previous evidence has suggested that there may be an interaction between the protein conjugates in vaccines and other vaccine antigens, which may alter the immunogenicity or safety of coadministered vaccines [Reference Findlow and Borrow15, Reference Bröker16]. However, coadministration of paediatric vaccines with other licensed meningococcal quadrivalent vaccines that are conjugated with either diphtheria CRM protein or tetanus toxoid proteins has been shown to have no clinically relevant effect on immunogenicity or safety [Reference Keshavan34–Reference Assaf-Casals and Dbaibo36]. This is in-line with our findings in the current study, with no clinically relevant effect observed on the immunogenicity or safety of the tetanus toxoid conjugated MenACYW-TT vaccine or the coadministered vaccines.
Vaccination with MenACYW-TT revealed no apparent safety concerns among meningococcal-vaccine naïve toddlers aged 12–23 months, when administered alone or concomitantly with the licensed vaccines MMR, V, DTaP-IPV-HB-Hib, or PCV13. The solicited reactions were mainly Grades 1 and 2, and there were no immediate AEs, and no related SAEs or SAEs leading to study discontinuation. The concomitant licensed vaccines MMR, V, DTaP-IPV-HB-Hib or PCV13 were also found to be well-tolerated when co-administered with MenACYW-TT, with comparable safety profiles. The rates of solicited injection site and systemic reactions tended to be higher when PCV13 was given with MenACYW-TT; however, those rates, including rates of Grade 3 reactions, were low.
Lower rates of AEs were reported across all vaccine groups in participants from the Russian Federation as compared to the other countries. A similar observation has been reported in a trial conducted with another quadrivalent meningococcal conjugate vaccine [Reference Javadekar37]. It is not possible to speculate the exact reason(s) for such a difference, but this observation may be influenced by the environment and the cultural differences in medical practice in the Russian Federation vs. the other countries, and as this study does not compare results across countries this disparity should not affect the conclusions drawn here.
A lower hSBA GMT for serogroup A was observed when MenACYW-TT vaccine was co-administered with PCV13 vaccine compared to when MenACYW-TT vaccine was administered alone in the Russian Federation. This observation was not seen when the data were analysed using seroprotection rates (post-vaccination hSBA titres ≥1:8) which were high in both groups, or when responses were evaluated using the rSBA assay. Since the observation was limited to only hSBA GMTs, and not seen with any other endpoint (with either hSBA or rSBA), these data have minimal clinical significance. A similar observation where the rSBA GMT for serogroup A only has been reported to be lower upon co-administration of another quadrivalent meningococcal vaccine with PCV13 has been documented [Reference Cutland38].
Due to the higher risk for IMD in infants and children <5 years of age and the high morbidity and mortality of IMD, notwithstanding antimicrobial treatment, meningococcal vaccines are often included in childhood vaccination programmes [Reference Borrow1–Reference Acevedo3, Reference Booy10, Reference Presa14]. The incidence of IMD due to serogroup C has been reduced in many regions through the inclusion of monovalent C vaccines in childhood vaccination programmes [Reference Lawrence39–Reference Jafri41]. However, given the recent increases in cases of IMD due to serogroups W and Y in various regions, the inclusion of quadrivalent meningococcal vaccines into vaccination programmes is becoming increasingly common in order to try to limit IMD cases [Reference Borrow1, Reference Acevedo3, Reference Tsang9–Reference Presa14].
There are a number of limitations to this study. While this study assessed the coadministration of several different paediatric vaccines, as is relevant to different vaccination schedules, the number of participants in each group were consequently small and it was designed as a descriptive study only, and not powered to make statistical comparisons between the groups. The choice of concomitant vaccines in this study, however, will provide the opportunity for these results to be extrapolated to other countries where similar vaccines are used. Finally, due to the design of this study, the participants and the vaccinating study staff were unblinded to the vaccines administered; however, the laboratory staff conducting the immunogenicity assays were kept blinded to group allocation.
MenACYW-TT was safe and immunogenic when coadministered with MMR and varicella vaccines, DTaP-IPV-HepB-Hib vaccine, or PCV13 vaccine in healthy toddlers aged 12–23 months. This will facilitate the introduction of MenACYW-TT into paediatric vaccination schedules, as it can be co-administered with other routine childhood vaccines used in several national immunisation programmes around the world.
Supplementary material
The supplementary material for this article can be found at https://doi.org/10.1017/S0950268821000698.
Acknowledgements
The authors would like to thank the participants and their families, the investigators (S Kharit, St Petersburg, Russia; V Romanenko, Yekaterinburg, Russia; I Osipova, Barnaul, Russia; V Anokhin, Kazan, Russia; T Romanova, Samara, Russia; A Galustyan, St Petersburg, Russia; V Rodnikova, Murmansk, Russia; V Gorogin, Krasnodar, Russia; Y Kaovshirina, Tomsk, Russia; DH Kim, Seoul, Korea; S Cha, Seoul, Korea; Y-S Choi, Seoul, Korea; HM Kim, Gangwon-do, Korea; J Lee, Seoul, Korea; YK Kim, Gyeonggi-do, Korea; SM Shin, Seoul, Korea; GC Jang, Gyeonggi-do, Korea; CS Kim, Daegu, Korea; ES Song, Gwangju, Korea; JH Choi, Jeju, Korea; SH Chang, Seoul, Korea; SM Villagomez, Morelos, Mexico) and their staff and the Sanofi Pasteur study team especially Jennifer Kinsley, Amy Strickland, Alexander Goldstein, Irina Figurina, Isabelle Lacroix, Seong Bin Lee, Alexandra Quiroz and Suree Satayavisit. The authors would also like to thank the team at Public Health England, Manchester, UK, for the work done to carry out the laboratory assays used in the study, and Jean-Sébastien Persico for editorial assistance and manuscript coordination on behalf of Sanofi Pasteur. Editorial assistance with the preparation of this manuscript was provided by Nicola Truss PhD, inScience Communications, Springer Healthcare Ltd, UK, and was funded by Sanofi Pasteur.
Conflicts of interest
MSD, EJ, JO, JP, DC, DN, SB'C and MB are employees of Sanofi Pasteur, and may hold shares and/or stock options in the company. JLA-G received Sanofi-Pasteur resources to carry out this project through his institution. LN-B, K-HK, KL, C-WB, IARK OP and WJ declare no conflict of interest.
Financial support
This study was funded by Sanofi Pasteur.
Author contributions
MSD, EJ and SB were involved in the study design, LN-B, JLA-G, K-HK, KL, WJ, OP, IARK and CWB were involved in data collection, all authors were involved in data analysis and interpretation, critical review and revision of the draft, and final approval for submission.
Data
Qualified researchers may request access to patient-level data and related study documents including the clinical study report, study protocol with any amendments, blank case report form, statistical analysis plan and dataset specifications. Patient-level data will be anonymised and study documents will be redacted to protect the privacy of trial participants. Further details on Sanofi's data-sharing criteria, eligible studies and process for requesting access can be found at: https://www.clinicalstudydatarequest.com