Book contents
- The Cambridge Handbook of Evolutionary Perspectives on Human Behavior
- The Cambridge Handbook of Evolutionary Perspectives on Human Behavior
- Copyright page
- Dedication
- Contents
- Figures
- Tables
- Contributors
- Preface
- Acknowledgments
- Part I The Comparative Approach
- Part II Sociocultural Anthropology and Evolution
- Part III Evolution and Neuroscience
- Part IV Group Living
- Part V Evolution and Cognition
- Part VI Evolution and Development
- Part VII Sexual Selection and Human Sex Differences
- Part VIII Abnormal Behavior and Evolutionary Psychopathology
- Part IX Applying Evolutionary Principles
- Part X Evolution and the Media
- Index
- References
Part III - Evolution and Neuroscience
Published online by Cambridge University Press: 02 March 2020
- The Cambridge Handbook of Evolutionary Perspectives on Human Behavior
- The Cambridge Handbook of Evolutionary Perspectives on Human Behavior
- Copyright page
- Dedication
- Contents
- Figures
- Tables
- Contributors
- Preface
- Acknowledgments
- Part I The Comparative Approach
- Part II Sociocultural Anthropology and Evolution
- Part III Evolution and Neuroscience
- Part IV Group Living
- Part V Evolution and Cognition
- Part VI Evolution and Development
- Part VII Sexual Selection and Human Sex Differences
- Part VIII Abnormal Behavior and Evolutionary Psychopathology
- Part IX Applying Evolutionary Principles
- Part X Evolution and the Media
- Index
- References
Summary
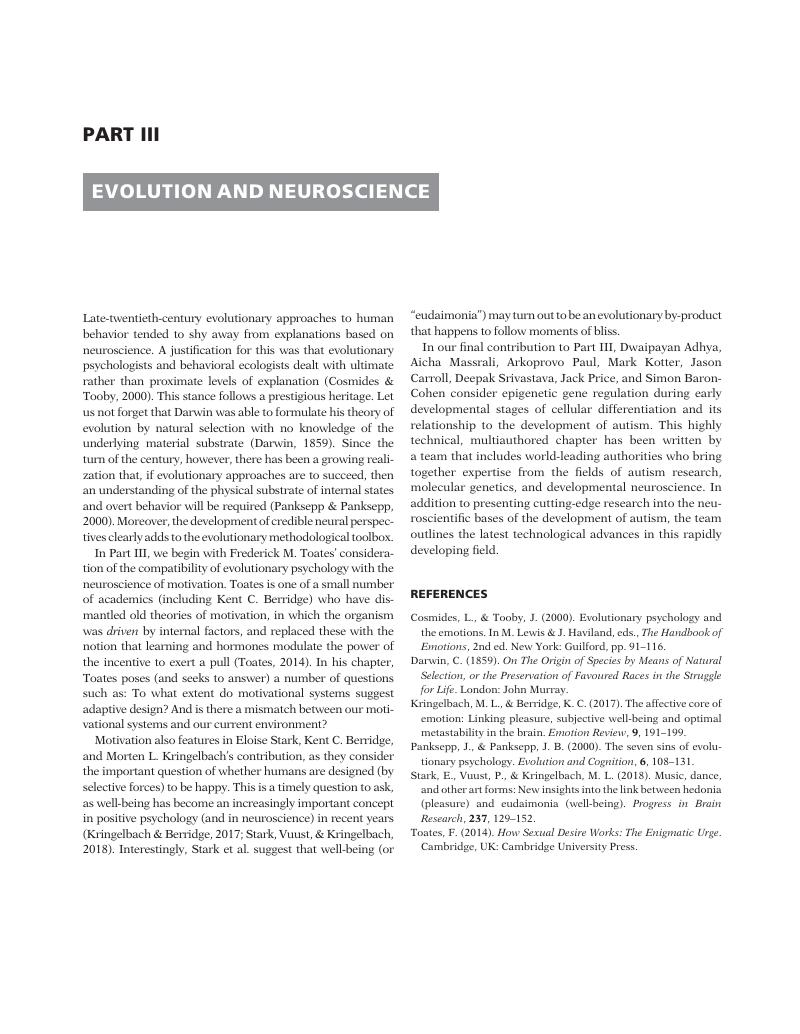
- Type
- Chapter
- Information
- Publisher: Cambridge University PressPrint publication year: 2020