Book contents
- Frontmatter
- Contents
- Acknowledgments
- Introduction
- Part I Approaches to the origin of life
- Part II What is life? The bio-logics of cellular life
- Part III Order and organization in biological systems
- Part IV The world of vesicles
- Part V Towards the synthetic biology of minimal cells
- As a way of conclusion
- Appendix The open questions about the origin of life
- References
- Names index
- Subject index
- References
References
Published online by Cambridge University Press: 05 September 2016
- Frontmatter
- Contents
- Acknowledgments
- Introduction
- Part I Approaches to the origin of life
- Part II What is life? The bio-logics of cellular life
- Part III Order and organization in biological systems
- Part IV The world of vesicles
- Part V Towards the synthetic biology of minimal cells
- As a way of conclusion
- Appendix The open questions about the origin of life
- References
- Names index
- Subject index
- References
Summary
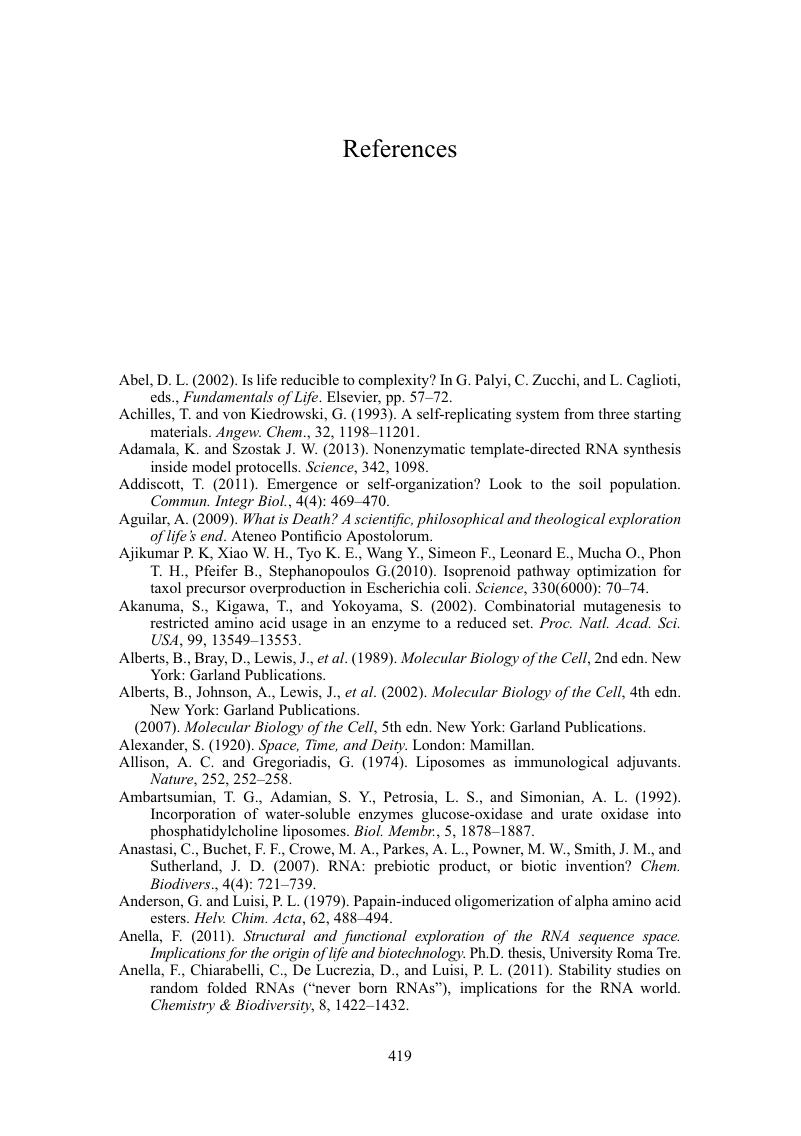
- Type
- Chapter
- Information
- The Emergence of LifeFrom Chemical Origins to Synthetic Biology, pp. 419 - 456Publisher: Cambridge University PressPrint publication year: 2016