Book contents
- Frontmatter
- Contents
- List of Figures
- List of Tables
- List of Contributors
- Introduction
- PART I ADVANCES IN GPCR PROTEIN RESEARCH
- 1 The evolution of the repertoire and structure of G protein-coupled receptors
- 2 Functional studies of isolated GPCR-G protein complexes in the membrane bilayer of lipoprotein particles
- PART II OLIGOMERIZATION OF GPCRS
- PART III GPCR SIGNALING FEATURES
- PART IV LIGAND PHARMACOLOGY OF GPCRS
- PART V PHYSIOLOGICAL FUNCTIONS AND DRUG TARGETING OF GPCRS
- Index
- References
2 - Functional studies of isolated GPCR-G protein complexes in the membrane bilayer of lipoprotein particles
from PART I - ADVANCES IN GPCR PROTEIN RESEARCH
Published online by Cambridge University Press: 05 June 2012
- Frontmatter
- Contents
- List of Figures
- List of Tables
- List of Contributors
- Introduction
- PART I ADVANCES IN GPCR PROTEIN RESEARCH
- 1 The evolution of the repertoire and structure of G protein-coupled receptors
- 2 Functional studies of isolated GPCR-G protein complexes in the membrane bilayer of lipoprotein particles
- PART II OLIGOMERIZATION OF GPCRS
- PART III GPCR SIGNALING FEATURES
- PART IV LIGAND PHARMACOLOGY OF GPCRS
- PART V PHYSIOLOGICAL FUNCTIONS AND DRUG TARGETING OF GPCRS
- Index
- References
Summary
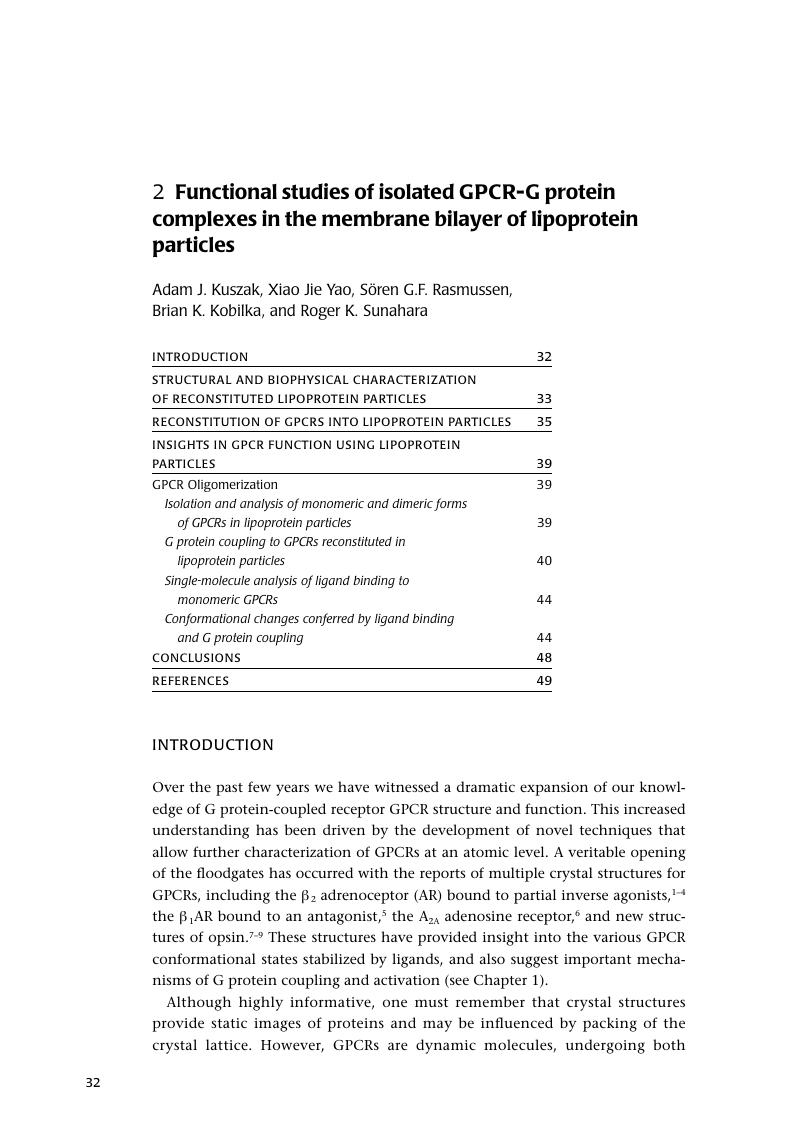
- Type
- Chapter
- Information
- G Protein-Coupled ReceptorsStructure, Signaling, and Physiology, pp. 32 - 52Publisher: Cambridge University PressPrint publication year: 2010