Book contents
- Frontmatter
- Dedication
- Contents
- Preface
- Acknowledgments
- 1 Introduction and Preliminaries
- 2 Molecular Orbitals/Potentials/Dynamics and Quantum Energy States
- 3 Carrier Energy Transport and Transformation Theories
- 4 Phonon Energy Storage, Transport, and Transformation Kinetics
- 5 Electron Energy Storage, Transport, and Transformation Kinetics
- 6 Fluid Particle Energy Storage, Transport, and Transformation Kinetics
- 7 Photon Energy Storage, Transport, and Transformation Kinetics
- APPENDIX A Tables of Properties and Universal Constants
- APPENDIX B Derivation of Green–Kubo Relation
- APPENDIX C Derivation of Minimum Phonon Conductivity Relations
- APPENDIX D Derivation of Phonon Boundary Resistance
- APPENDIX E Derivation of Fermi Golden Rule
- APPENDIX F Derivation of Equilibrium, Particle Probability Distribution Functions
- APPENDIX G Phonon Contributions to the Seebeck Coefficient
- APPENDIX H Monte Carlo Method for Carrier Transport
- APPENDIX I Ladder Operators
- Nomenclature
- Abbreviations
- Glossary
- References
- Index
- References
References
Published online by Cambridge University Press: 05 June 2014
- Frontmatter
- Dedication
- Contents
- Preface
- Acknowledgments
- 1 Introduction and Preliminaries
- 2 Molecular Orbitals/Potentials/Dynamics and Quantum Energy States
- 3 Carrier Energy Transport and Transformation Theories
- 4 Phonon Energy Storage, Transport, and Transformation Kinetics
- 5 Electron Energy Storage, Transport, and Transformation Kinetics
- 6 Fluid Particle Energy Storage, Transport, and Transformation Kinetics
- 7 Photon Energy Storage, Transport, and Transformation Kinetics
- APPENDIX A Tables of Properties and Universal Constants
- APPENDIX B Derivation of Green–Kubo Relation
- APPENDIX C Derivation of Minimum Phonon Conductivity Relations
- APPENDIX D Derivation of Phonon Boundary Resistance
- APPENDIX E Derivation of Fermi Golden Rule
- APPENDIX F Derivation of Equilibrium, Particle Probability Distribution Functions
- APPENDIX G Phonon Contributions to the Seebeck Coefficient
- APPENDIX H Monte Carlo Method for Carrier Transport
- APPENDIX I Ladder Operators
- Nomenclature
- Abbreviations
- Glossary
- References
- Index
- References
Summary
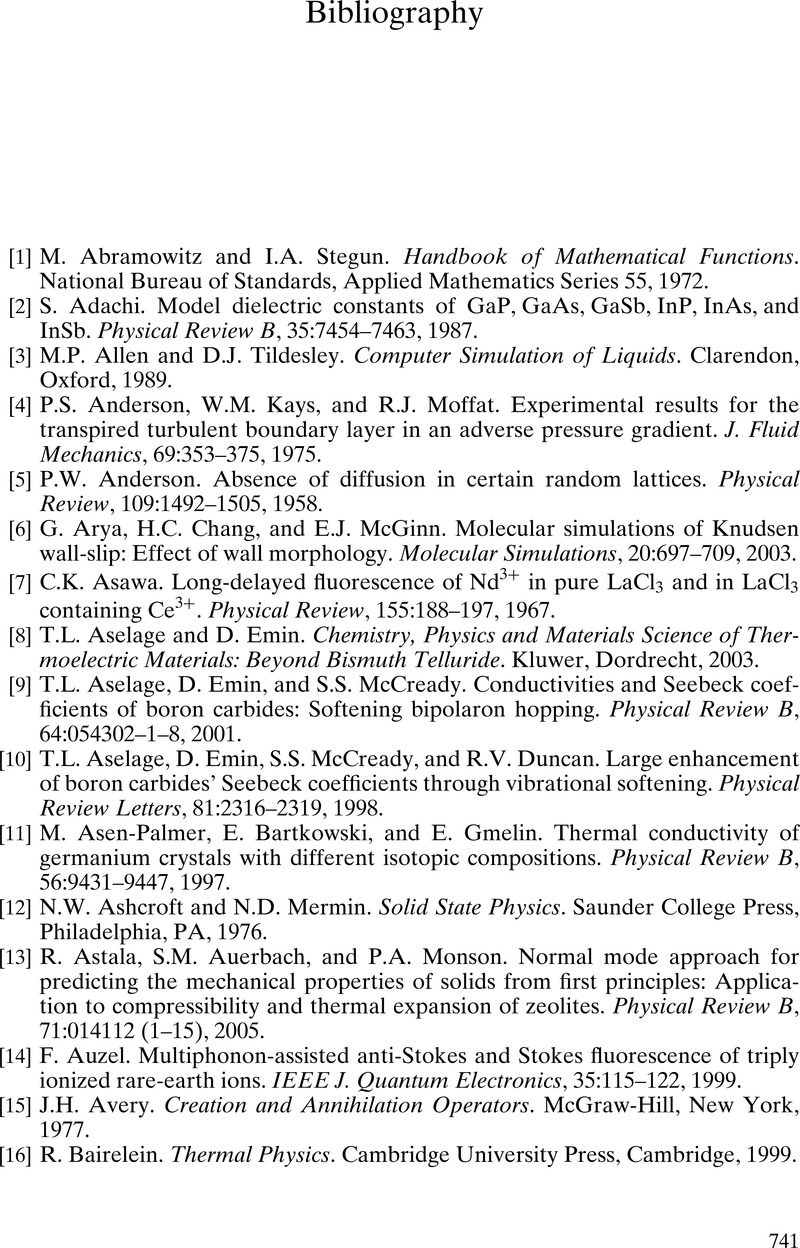
- Type
- Chapter
- Information
- Heat Transfer Physics , pp. 741 - 764Publisher: Cambridge University PressPrint publication year: 2014