Section 1 - Basic Principles
Published online by Cambridge University Press: 03 December 2019
Summary
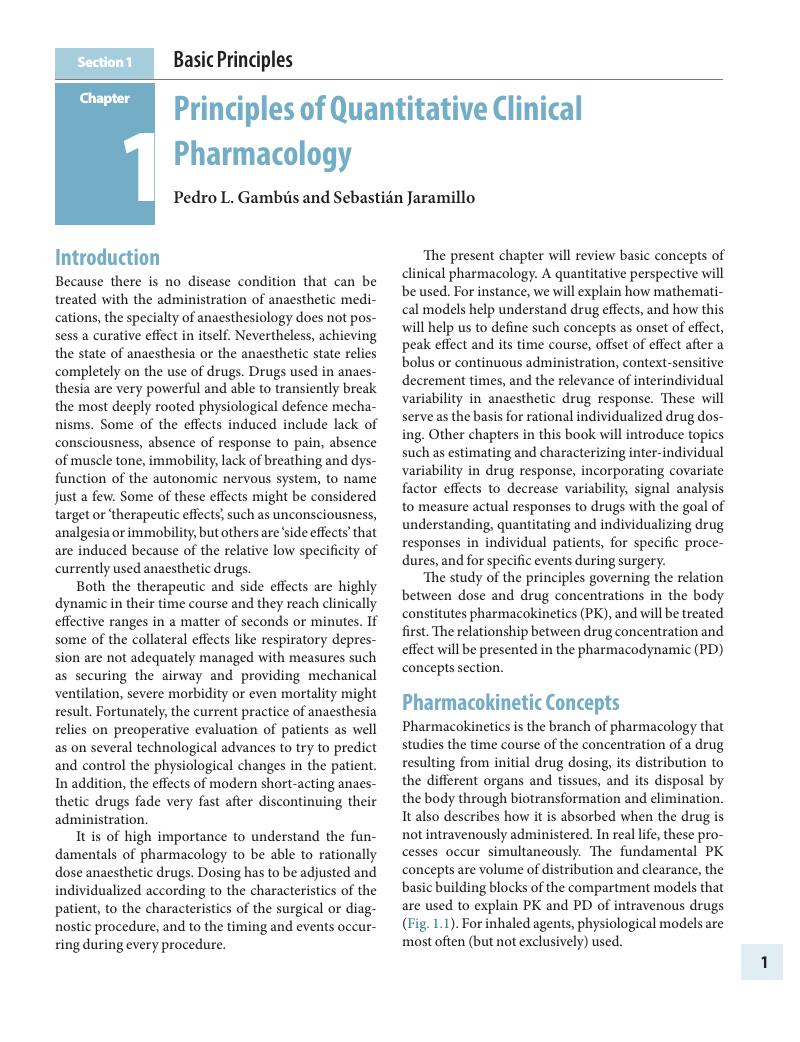
- Type
- Chapter
- Information
- Personalized AnaesthesiaTargeting Physiological Systems for Optimal Effect, pp. 1 - 102Publisher: Cambridge University PressPrint publication year: 2020