Book contents
- Textbook of Neural Repair and Rehabilitation
- Textbook of Neural Repair and Rehabilitation
- Copyright page
- Contents
- Contributor affiliations
- Preface
- Introduction toNeural Repair and Rehabilitation
- Section 1 Neural plasticity: cellular and molecular mechanisms of neural plasticity
- Section 2 Functional plasticity in the central nervous system
- Section 3 Plasticity after injury to the central nervous system
- Section 4 Neural repair: basic cellular and molecular processes
- Section 5 Determinants of regeneration in the injured nervous system
- Chapter 23 Non-mammalian models of nerve regeneration
- Chapter 24 Myelin-associated axon growth inhibitors
- Chapter 25 Inhibitors of axonal regeneration
- Chapter 26 Glial development and axon regeneration
- Chapter 27 Effects ofthe glial scar and extracellular matrix molecules on axon regeneration
- Chapter 28 Therole of the inflammatory response in central nervous system injury and regeneration
- Chapter 29 Neurotrophin repair of spinal cord damage
- Chapter 30 Intraneuronal determinants of axon regeneration
- Section 6 Promotion of regeneration in the injured nervous system
- Section 7 Translational research: application to human neural injury
- Index
Chapter 23 - Non-mammalian models of nerve regeneration
from Section 5 - Determinants of regeneration in the injured nervous system
Published online by Cambridge University Press: 05 May 2014
- Textbook of Neural Repair and Rehabilitation
- Textbook of Neural Repair and Rehabilitation
- Copyright page
- Contents
- Contributor affiliations
- Preface
- Introduction toNeural Repair and Rehabilitation
- Section 1 Neural plasticity: cellular and molecular mechanisms of neural plasticity
- Section 2 Functional plasticity in the central nervous system
- Section 3 Plasticity after injury to the central nervous system
- Section 4 Neural repair: basic cellular and molecular processes
- Section 5 Determinants of regeneration in the injured nervous system
- Chapter 23 Non-mammalian models of nerve regeneration
- Chapter 24 Myelin-associated axon growth inhibitors
- Chapter 25 Inhibitors of axonal regeneration
- Chapter 26 Glial development and axon regeneration
- Chapter 27 Effects ofthe glial scar and extracellular matrix molecules on axon regeneration
- Chapter 28 Therole of the inflammatory response in central nervous system injury and regeneration
- Chapter 29 Neurotrophin repair of spinal cord damage
- Chapter 30 Intraneuronal determinants of axon regeneration
- Section 6 Promotion of regeneration in the injured nervous system
- Section 7 Translational research: application to human neural injury
- Index
Summary
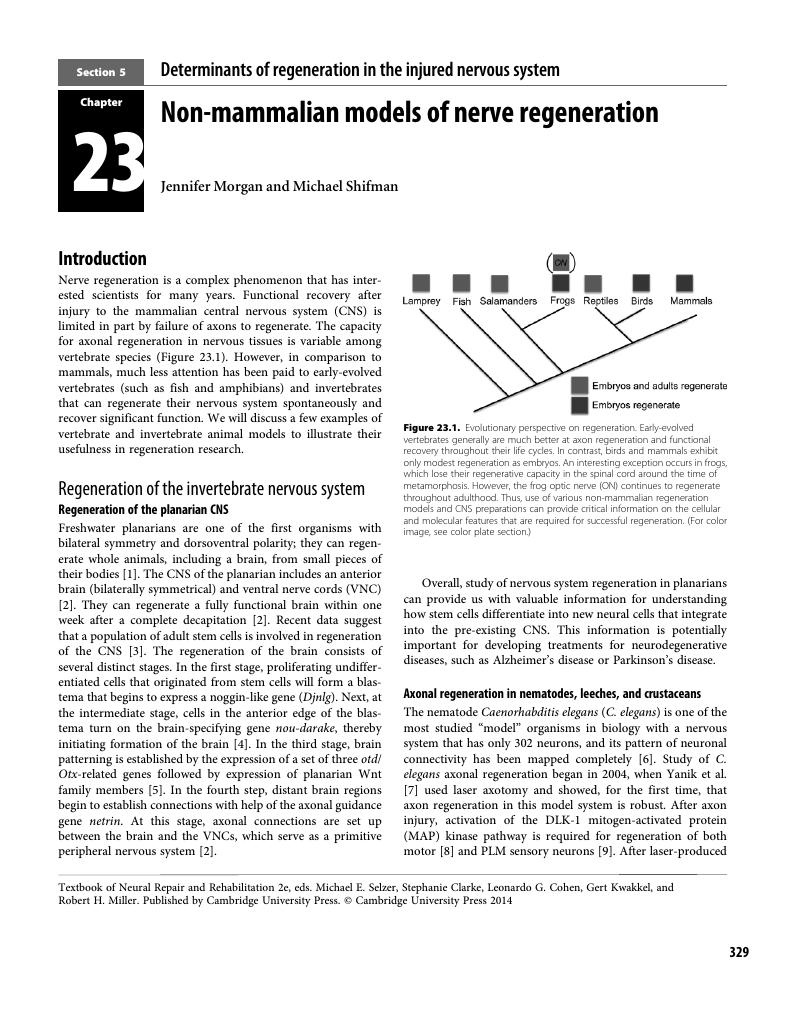
- Type
- Chapter
- Information
- Textbook of Neural Repair and Rehabilitation , pp. 329 - 338Publisher: Cambridge University PressPrint publication year: 2014
- 4
- Cited by