Evidence exists that a more acidic diet is detrimental to bone health(Reference Welch, Bingham, Reeve and Khaw1Reference New, Macdonald, Campbell, Martin, Garton, Robins and Reid2Reference Macdonald, New, Fraser, Campbell and Reid3Reference Alexy, Remer, Manz, Neu and Schoenau4Reference Arnett5). Net acid excretion has been related to dietary acid–base balance in small intervention studies mainly designed to test the effect of changes in acid–base balance on indicators of bone health(Reference Trilok and Draper6Reference Lutz7Reference Reddy, Wang, Sakhaee, Brinkley and Pak8Reference Breslau, Brinkley, Hill and Pak9Reference Lemann, Lennon, Goodman, Litzow and Relman10Reference Buclin, Cosma, Appenzeller, Jacquet, Decosterd, Biollaz and Burckhardt11Reference Frassetto, Morris and Sebastian12Reference Remer and Manz13Reference Marangella, Di Stefano, Casalis, Berutti, D'Amelio and Isaia14). Precise measurements of net endogenous acid excretion require labour- and laboratory-intensive steady-state measurements of dietary nutrient intakes and urine and stool composition and alternative methodologies are neededReference Frassetto, Morris and Sebastian(15, Reference Remer and Manz16). One alternative is urine pH, a reflection of acid–base balance, which is readily measurable but has not been related to habitual dietary intake in general populations. If urine pH were related to habitual diet this would provide further evidence for the potential for dietary acid–base balance to affect bone health. If it were also related to fruit and vegetable intake it could have potential for monitoring changes in fruit and vegetable consumption.
The acid–base equilibrium in the body is maintained within tight limits by three mechanisms; blood and tissue buffering, excretion of CO2 by the lungs and renal excretion of H+ and regeneration of HCO3–(Reference Hainsworth17, Reference Drage and Wilkinson18). Diet has the potential to contribute to mild metabolic acidosis and affect acid–base status via the supply of acid and alkaline precursors from foods(Reference Frassetto, Morris and Sebastian15, Reference Remer and Manz16, Reference Remer19). Hepatic oxidation of the S-containing amino acids, cysteine and methionine (found in meats, fish, cereal and dairy foods), generates H+(Reference Remer and Manz16, Reference Frassetto, Todd, Morris and Sebastian20). This is balanced by carbonate present as alkaline salts in fruits and vegetables, that also supply large amounts of Mg and K in the diet(Reference Frassetto, Todd, Morris and Sebastian20). Thus dietary acid–base load is a balance between protein-containing foods such as meats, cereals and dairy foods that supply acid and fruit and vegetable foods that supply base precursors(Reference Sebastian, Frassetto, Sellmeyer, Merriam and Morris21). As the kidneys are the main route of excretion of dietary H+ ions, the acid–base load of the diet is reflected in urine pH and net acid excretion, provided kidney function is not compromised by disease(Reference Reddy, Wang, Sakhaee, Brinkley and Pak8, Reference Lemann, Lennon, Goodman, Litzow and Relman10, Reference Buclin, Cosma, Appenzeller, Jacquet, Decosterd, Biollaz and Burckhardt11, Reference Marangella, Di Stefano, Casalis, Berutti, D'Amelio and Isaia14, Reference Frassetto, Todd, Morris and Sebastian20, Reference Remer22, Reference Michaud, Troiano, Subar, Runswick, Bingham, Kipnis and Schatzkin23).
The health benefits of high fruit and vegetable intake are well recognised and extensive public health programmes exist to encourage increased consumption but, at present, individuals cannot monitor the effects of change in dietary behaviour. Since we are unaware of previous studies relating urine pH to dietary determinants of acid–base load in a large free-living population of men and women, the purpose of the present study was to investigate if there was a relationship. We particularly wanted to investigate whether urine pH was related to specific foods, particularly fruit and vegetables and meat consumption. Therefore, an established biomarker of fruit and vegetable intake, plasma vitamin C, was also compared with urine pH. Second, because dietary methods differ in their estimates of foods and nutrients and because food consumption and urine excretion vary throughout the day, we wanted to compare relationships between different methodologies used to assess the relationship between diet and urine pH.
Methods
Approximately 25 000 men and women aged 40–79 years living in the general community participated in a baseline examination in 1993–7 in Norfolk (UK), as part of the European Prospective Investigation into Cancer and Nutrition (EPIC), a ten-country collaboration of diet and cancer and, in the EPIC-Norfolk study, other health outcomes(Reference Day, Oakes, Luben, Khaw, Bingham, Welch and Wareham24). Men and women were invited to attend a health examination where height and weight were measured by trained nurses using standardised protocols(Reference Day, Oakes, Luben, Khaw, Bingham, Welch and Wareham24). BMI was calculated as weight in kilograms divided by height in meters squared. Freshly voided, casual urine samples were collected. pH was measured using Ames multiple reagent strips (Miles Laboratories, Inc., Elkhart, IN, USA) with a range of detection between 5 and 8·5 (0·5 to 1·0 difference in units). Reliability data are unavailable for Ames multiple reagent strips. However, one study reported good reliability for pH measured using Clinitek-50 dipsticks, although their data were not presented(Reference Buys Roessingh, Drukker and Guignard25).
Smoking habit was ascertained by response to the question ‘Do you smoke cigarettes now?’ There were 22 034 men and women aged 39–78 years with complete data for urine pH and the FFQ used in these analyses.
Sub-study
In a sub-study of 363 men and women (an average of 1 year after the baseline health check) 24 h urine samples were collected and validated for completeness with p-aminobenzoic acid. Boric acid was used as preservative; a previous study showed that when used in concentrations of 0·5–2 g/l it does not affect urine pH(Reference Michaud, Troiano, Subar, Runswick, Bingham, Kipnis and Schatzkin23). pH was measured using a Jenway 3310 pH meter (Jenway, Dunmow, Essex, UK), detection range − 2 to 16 with a resolution of 0·01 pH units.
Dietary methods
All participants were asked to complete a self-administered EPIC-Norfolk FFQ, complete a 7 d food diary and a detailed health and lifestyle questionnaire(Reference Welch, Luben, Khaw and Bingham26, Reference Bingham, Welch, McTaggart, Mulligan, Runswick, Luben, Oakes, Khaw, Wareham and Day27). The FFQ was designed to estimate habitual intake over the previous year and nutrients were computed using an in-house program, the Compositional Analyses from Frequency Estimates (CAFE) program(Reference Welch, Luben, Khaw and Bingham26). The 7 d food diary included an interview about the first day and was completed for the remaining 6 d by the participant. Data were entered using the Data into Nutrients for Epidemiological Research (DINER) system and a series of checks performed to correct for data-entry errors(Reference Welch, McTaggart, Mulligan, Luben, Walker, Khaw, Day and Bingham28).
The potential renal acid load (PRAL) index was calculated using individual nutrients derived from the FFQ and 7 d food diary using the formula:
PRAL (mEq/d) = (P (mg/d) × 0·0366+ protein (g/d) × 0·4888) – (K (mg/d) × 0·0205+ Ca (mg/d) × 0·0125+ Mg (mg/d) × 0·0263)(Reference Remer and Manz16, Reference Welch, Luben, Khaw and Bingham26).
The protein:K ratio was calculated(Reference Frassetto, Todd, Morris and Sebastian20).
Intake of foods was calculated from the FFQ and 7 d food diary(Reference Welch, Luben, Khaw and Bingham26) (AA Welch, AA Mulligan, A McTaggart, KT Khaw and SA Bingham, unpublished results).
Plasma vitamin C
Since plasma vitamin C is an established biomarker of fruit and vegetable consumption, and fruit and vegetables are one of the main contributors to the nutrients in the PRAL index, we investigated whether plasma vitamin C was related to urine pH. Plasma vitamin C was measured in non-fasting venous blood samples taken in citrate bottles. After overnight storage in a dark box at 4–7°C, sample bottles were centrifuged at 2100 g for 15 min at 4°C and plasma was stabilised in a standard volume of meta-phosphoric acid at − 70°C. Plasma vitamin C was estimated with a fluorimetric assay within 1 week of sampling(Reference Vuilleumier, Keller and Keck29). The CV was 5·6 % at the lower end of the range (mean 33·2 μmol/l) and 4·5 % at the upper end (102·3 μmol/l). Plasma vitamin C was available in 19 338 individuals and was regressed against urine pH and also adjusted for age, BMI and smoking habit. Individuals taking supplements were not excluded from the analyses.
Statistical analyses
pH measured in casual urine samples was grouped into categories 5·0, 6·0, 6·5, 7·0 and 7·5 and over. The correlation was calculated between casual urine pH, as a continuous variable, with pH measured in 24 h urine samples.
The interaction between pH and sex was significant (P = 0·009). Univariate regression coefficients were calculated in men and women to estimate the relationship between casual urine pH and age, BMI, physical activity, smoking habit and the dietary covariates to enable comparison between nutrients and food groups. PRAL was standardised by dividing by its standard deviation (12·4). The other dietary variables were standardised by dividing by their average portion sizes (fruit and vegetables and meats, 80 g; cereal foods and meats, 100 g).
We compared mean intake of PRAL and foods in men and women in the different categories of casual urine pH before and after adjusting for age, BMI, physical activity and smoking habit (model 1). As protein, glucose and ketones in urine, diagnosed blood pressure and diuretic medication influence urine pH the analyses were also repeated after excluding those with positive urinary ketones, glucose or protein or those with diagnosed high blood pressure or taking diuretic medication. The analyses were also repeated using the protein:K ratio.
PRAL was also divided into quintiles and the percentage of foods contributing to different categories of PRAL was calculated for men and women. The percentage contribution of individual food groups was calculated as a percentage of the total food weight provided by the food groups; fruit and vegetables, meats, cereal foods and dairy.
The relationship between fruits and vegetables and pH was further examined by adjusting for other dietary factors (meats, cereal and dairy foods) as well as the covariates age, BMI, physical activity and smoking habit.
In a further analysis to compare the relationship of urine pH with dietary intake calculated using two different dietary methods, four combinations were tested in a sub-study of 363 men and women who also had 24 h urine collections; FFQ and casual urine, FFQ and 24 h urine, 7 d diary and casual urine, 7 d diary and 24 h urine. Multiple regression models were run with pH on either PRAL (model 2) or fruit and vegetables and meats (model 3) in addition to the covariates age, BMI, physical activity and smoking habit. To enable comparison between the variables derived from the two dietary methods, variables were standardised by dividing by their standard deviation.
We also compared pH in individuals who ate meat (meat-eaters) with those who ate no meat (non-meat-eaters). Mean pH by meat-eating habit was also adjusted for age, BMI, physical activity and smoking habit.
Statistical analyses were performed with Stata® statistical software (version 8.2; StataCorp LP, College Station, TX, USA).
Results
pH measured in casual urine samples was not significantly different in men and women (Table 1). The percentage of men and women in each pH category is shown in Table 3.
Table 1 Urine pH, age, body mass index, physical activity and smoking habit in men and women in the main study and sub-study (Mean values and standard deviations)
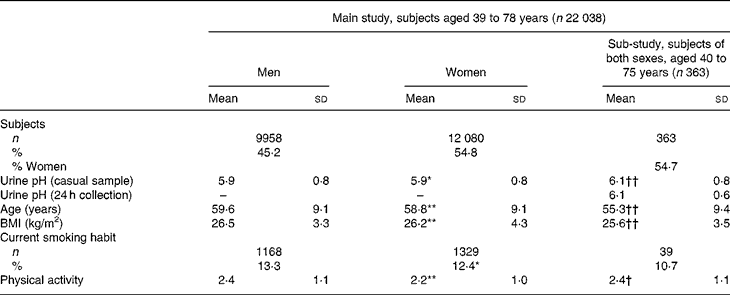
Value is significantly different from that for men: *P = 0·09, **P < 0·001 (unpaired two-sample t test for continuous variables and χ2 test for categorical variables).
Mean value is significantly different from that for the main cohort: † P = 0·007, †† P < 0·001.
Individuals in the sub-study were younger and had a lower BMI than the main cohort (P < 0·001). For the casual urine samples they also had a significantly more alkaline urine pH (P < 0·001). The correlation between pH measured in the casual and 24 h urine samples in the sub-study was 0·22 (P < 0·001).
Age, BMI and plasma vitamin C were significantly related to urine pH, as was smoking habit, in women only (Table 2). PRAL, fruits and vegetables and meats were also significantly related to urine pH (Table 2).
Table 2 Univariate regression between urine pH measured in casual urines and age, body mass index, physical activity and smoking habit, plasma vitamin C and dietary variables from the European Prospective Investigation into Cancer and Nutrition (EPIC)-Norfolk food-frequency questionnaire in 22 034 men and women aged 39 to 78 years (Coefficients and 95 % confidence intervals)

PRAL, potential renal acid load.
* PRAL was divided by standard deviation 12·4 mEq/d.
† One portion of fruit and vegetables is 80 g, one portion of meat is 80 g, one portion of dairy products is 100 g, one portion of cereals and cereal products is 100 g.
Men had a more acidic PRAL, a lower intake of fruit and vegetables and a higher intake of meats, cereals and dairy foods than women (P < 0·001 for PRAL and all food groups) (Table 3).
Table 3 Mean intake of potential renal acid load (PRAL) and selected food groups calculated by the European Prospective Investigation into Cancer and Nutrition (EPIC)-Norfolk food-frequency questionnaire, stratified by urine pH in casual urines for 22 034 men and women aged 39 to 78 years (Mean values and standard deviations)

Fruits and vegetables: all fruits and vegetables excluding potatoes and fruit juices. Meats: meat and meat products. Cereal foods: breads, breakfast cereals, cakes and buns, sweet and savoury biscuits, pasta, rice. Dairy: milk and dairy products including yogurts and cheese.
* Mean value was significantly from that for women (P < 0·001).
† P for trend calculated using ANOVA.
‡ Adjusted for age, BMI, smoking habit and physical activity (model 1). Mean values with their standard errors.
There was a continuous relationship between an increasingly alkaline urine pH and a more alkaline diet as shown by (FFQ) PRAL, which was significant both before and after adjustment and also after exclusion for urinary protein, glucose and ketones and those with diagnosed high blood pressure and diuretic medication (data not shown) (P < 0·001) (Table 3). The difference in PRAL between the pH categories 5·0 and 7·5 and over was 4·2 mEq/d in both men and women. The analysis with protein:K ratio was not different from those with PRAL (data not shown). For all categories of pH, PRAL was more negative for women, i.e. more alkaline than for men. Consumption of fruit and vegetables and meats was also significantly related to urine pH even after adjustment for age, BMI, physical activity and smoking habit. There was a difference after adjustment for age, BMI, physical activity and smoking habit in intake of fruit and vegetables between pH 5·0 and pH 7·5 (measured in the casual urine sample) and over in men of 50 g and of 68 g in women. For meat there was a difference of 5 g in men and 6 g in women. When fruit and vegetable consumption was adjusted for age, BMI, physical activity and smoking status, as well as other dietary covariates (meats, cereal and dairy foods), the gradient between intake of fruit and vegetables and categories of pH remained and the difference between categories pH 5·0 and pH 7·5 and over was 53 g in men and 67 g in women (the highest and lowest categories of pH) (Fig. 1). Although cereal and dairy foods contribute to the dietary acid load, their intake was not significantly related to urine pH with the exception of cereal foods (after adjustment) in men (Table 3). Men with the most alkaline urine pH had an intake of cereal foods that was 11 g lower than those with the most acid pH.

Fig. 1 Intake of fruit and vegetables according to pH category in men (□) and women () after adjusting for age, BMI, physical activity and smoking status, consumption of meats, cereal foods and dairy products. Values are means, with their standard errors represented by vertical bars. P for trend is significant in both men and women (P < 0·001).
The β coefficient of the relationship of standardised PRAL with pH was − 0·08 units of pH per standard deviation of PRAL (unadjusted and adjusted) and was significant before and after adjustment for covariates (P < 0·001).
In men and women who consumed no meat urine pH was more alkaline than in those who did (non-meat-eating men, pH 6·1; meat-eating men, pH 5·9 (P < 0·001); non-meat-eating women, pH 6·0; meat-eating women, pH 5·9 (P = 0·003)) and this remained significant after adjustment (men P = 0·007; women P = 0·039). Mean PRAL was − 15·78 mEq/d in non-meat-eating men and − 17·61 mEq/d in non-meat-eating women and was − 4·30 mEq/d in meat-eating men and − 6·88 mEq/d in meat-eating women.
When categorised according to quintiles of PRAL the percentages of food types differed in men and women. Women consumed a higher percentage of fruit and vegetables and dairy foods and a lower percentage of meats and cereal foods than men, with women in quintile 1 (the most alkaline) consuming 66 % of total food weight as fruit and vegetables whereas in men it was 60 % (data not shown). Consumption of dairy was 7 % in women and 6 % in men and women ate 21 % of food weight as cereal foods whereas in men this was 26 %. Women ate 6 % as meats and men 8 %.
There was a significant continuous relationship between urine pH and plasma vitamin C (Table 4), indicating that as there is a well-established relationship between fruit and vegetable intake and plasma vitamin C and the association with urine pH and fruit and vegetable intake is in the same direction, the results are not a chance finding.
Table 4 Mean plasma vitamin C (μmol/l) stratified by units of pH in 19 338 men and women aged 39 to 78 years (Mean values and standard deviations)

* Mean value was significantly from that for women (P < 0·001)·
† P for trend calculated using ANOVA.
‡ Adjusted for age, BMI, smoking habit and physical activity (model 1). Mean values with their standard errors.
When estimated by two different dietary methods (FFQ and 7 d food diary), differences between PRAL, fruits and vegetables, meats, cereal foods and dairy products were significant (Table 5). PRAL was less alkaline when estimated with the diary than with the FFQ. Intakes of meats and cereal foods were higher with the diary and of vegetables and dairy foods were much lower.
Table 5 Intake of potential renal acid load (PRAL) and food types according to dietary method in 363 men and women aged 40 to 75 years* (Mean values and standard deviations)

* Correlations between FFQ and diary data: PRAL r 0·48 (P < 0·001); fruit and vegetables r − 0·58 (P < 0·001); meats r 0·30 (P < 0·001); cereal foods r 0·42 (P < 0·001); dairy r 0·58 (P < 0·001).
The four-way comparison of estimates observed from the two dietary methods and the two methods of urine collection ranged from − 0·079 units per unit of PRAL from the FFQ and pH in casual urine to − 0·156 units using the diary and 24 h urine, a factor of 2 between the estimates (model 2; Table 6). Estimates of the relationship between PRAL and foods and urine pH were not significant with the FFQ or the diary and meat consumption and the casual urine but were significant for diet estimates from both methods and the 24 h urine. The β coefficients of foods estimated with the diary and the 24 h urine were the greatest; 0·156 pH units per g fruit and vegetables and − 0·086 units per g meats. For fruits and vegetables there was a factor of about 3·5 times between estimates from the 7 d food diary and 24 h urine and the casual urine and the FFQ; however, for meats the factor was 1·07, indicating a small difference.
Table 6 Comparison of relationship between urine pH and diet in a sub-study of 363 men and women with 24 h and casual urine collections and two dietary methods (7 d food diary and a food-frequency questionnaire) by regression of urine pH with sex, age, body mass index, physical activity and smoking habit and either potential renal acid load (PRAL) or fruit and vegetable and meat consumption* (Coefficients and standard errors)

* Variables PRAL, fruit and vegetables and meat were standardised by dividing by their standard deviations (see Table 5 for standard deviations).
† Model 2 included sex, PRAL, age, BMI, physical activity and smoking habit.
‡ Model 3 included sex, fruits and vegetables, meats, age, BMI, physical activity and smoking habit.
Discussion
We found a measurable difference in urine pH with dietary acid–base load and with fruit and vegetables and meat intakes in this population. The more alkaline the diet (lower PRAL) the more alkaline was urine pH, even after adjustment for age, BMI, physical activity and smoking habit and also after excluding for urinary protein, glucose, ketones, diagnosed high blood pressure and diuretic medication. The difference in PRAL between the most acidic and the most alkaline urine pH was 4·2 mEq/d. Plasma vitamin C was positively and significantly related to urine pH and as plasma vitamin C is also positively related to fruit and vegetable consumption this provides further validation for pH as a marker of diet quality, particularly fruits and vegetables. Although the effects of extreme changes in acid–base status are well established, we believe the present study to be the first to measure the more subtle relationship between diet and urine pH in such a large population.
In all categories of urine pH, PRAL was more acidic in men than women, with men eating more meat and less fruit and vegetables than women. Although one explanation for the sex differences might be different percentages of men and women in each pH category, this was not the case (see Table 3). Another explanation could be reporting bias in women, but we also found a relationship between plasma vitamin C and pH with a higher vitamin C in each category in women than men. It is also possible that men, with a larger kidney and body size, have greater capacity to excrete hydrogen ions than women. Although these sex differences are so far unexplained, it would be worthwhile investigating why, for the same urine pH, women had a more alkaline diet than men.
The results of the four-way comparisons, in the sub-study, between the dietary and urine methods found non-significant relationships with casual urine and fruit and vegetables and meats from the FFQ and with meat from the 7 d food diary. There were also significant differences between methods in PRAL and food intake. The correlation coefficient between the two dietary methods for PRAL was 0·48. However, associations with the 24 h urine were significant, indicating greater measurement error with pH measured in the casual urine sample and it is likely that our findings could be twice the scale if 24 h urine samples had been collected and the 7 d food diary used for the whole study.
Despite the homeostatic mechanisms for regulation of acid–base status and the likely measurement error with casual urine pH, we found associations between dietary acid–base load, meat and fruit and vegetables. There was a difference of 0·08 units of pH per 12·4 mEq/d of PRAL or the equivalent for 0·1 unit change in pH of 15·5 mEq PRAL per d. Although these differences may seem small scale, they are similar to interventions with diet and K salts(Reference Trilok and Draper6Reference Lutz7Reference Reddy, Wang, Sakhaee, Brinkley and Pak8Reference Breslau, Brinkley, Hill and Pak9Reference Lemann, Lennon, Goodman, Litzow and Relman10Reference Buclin, Cosma, Appenzeller, Jacquet, Decosterd, Biollaz and Burckhardt11Reference Frassetto, Morris and Sebastian12Reference Remer and Manz13Reference Marangella, Di Stefano, Casalis, Berutti, D'Amelio and Isaia14). Intervention studies with increased intakes of purified protein (wheat gluten, casein, lactalbumin, egg whites), in which Ca and P were held constant, found decreases in urine pHReference Trilok and Draper6, Reference Lutz7). Studies in which protein intake was increased and vegetable intake decreased led to more acidic urine pH, with differences of 6–13 g protein required to affect pH by 0·1 unit(Reference Reddy, Wang, Sakhaee, Brinkley and Pak8, Reference Remer and Manz13). In another volunteer study with diets designed to be acidic then alkaline, urine pH changed from 6·1 to 7·3(Reference Buclin, Cosma, Appenzeller, Jacquet, Decosterd, Biollaz and Burckhardt11). PRAL was calculated from an intervention study, with increased protein and decreased fruit and vegetable intake, and a change of 12·8 mEq/d PRAL induced a 0·1 unit reduction in pH(Reference Reddy, Wang, Sakhaee, Brinkley and Pak8). Two intervention studies with potassium citrate and sodium bicarbonate, respectively, found increases in urine pH from 6·11 to 6·33 and from 5·32 to 7·34(Reference Lemann, Lennon, Goodman, Litzow and Relman10, Reference Marangella, Di Stefano, Casalis, Berutti, D'Amelio and Isaia14). Calculations from another intervention study with varying doses of KHCO3 (30–90 mEq/d) indicated that 7–11·8 mEq KHCO3 per d were required to induce a 0·1 unit increase in pH(Reference Frassetto, Morris and Sebastian12).
We found the urine pH of meat-eaters was significantly lower than non-meat-eaters by 0·1 units both before and after adjustment for covariates. One other study found non-significant differences between the urine pH of omnivores and vegetarians (6·26 v. 6·45, respectively)(Reference Ball and Maughan30). An intervention study which held protein, Ca, K, P and Mg constant but changed the type of protein from vegetable to animal found a decrease in pH from 6·55 to 6·17(Reference Breslau, Brinkley, Hill and Pak9).
The correlation between the casual and 24 h urine, although significant, was relatively weak. This may be due to the time difference between the data collections or due to variability in urine pH over 24 h, which would be less well captured with casual urine samples. The difference could also be due to the lower precision of the dipstick (narrower range of measurements and larger measurement intervals) or even due to errors in colour interpretation. It would be important to identify and quantify sources of differences between the two methods and to evaluate intra-individual variability in pH excretion with dipstick measures in future studies. The reliability of dipstick measures also needs to be evaluated.
The limitations of the present study are the measurement errors relating to the use of casual urine samples and dipsticks, as well as the FFQ. However, random measurement error is likely to attenuate any associations. Indeed the sub-study found stronger relationships using the more precise methodology. Nevertheless, we were able to observe significant associations between usual diet and casual urine pH, measured with dipsticks, in this large population.
Among the non-dietary factors affecting urine pH are diabetic ketoacidosis, renal failure, diuretic medication and hypertension, but we found a relationship between diet and urine pH despite these factors. Another factor is the decline in kidney function with age, which would lead to an increase in blood hydrogen ions, and the possibility that urine pH would reflect diet less well in older individuals(Reference Frassetto, Morris and Sebastian31Reference Frassetto and Sebastian32Reference Alpern and Sakhaee33). However, substantial impairment in kidney function would need to occur before effects on urine excretion were seen. Despite these influences and the greater precision with more established steady-state methodology using diet, stool and urine composition, urine pH has advantages of convenience as an indicator of the acid–base status of the body. It is easily measured with detection strips and the present study indicates that even a casual urine sample could be used to monitor population dietary differences in acid–base load, fruit and vegetables and meat consumption. Studies to evaluate the effects of dietary modification of PRAL, fruits and vegetables and meats are needed. The clinical relevance of the differences in urine pH that we found, that are associated with dietary intake, also require further investigation. However, as urine pH is a readily measurable and tangible marker of fruit and vegetable and meat intake we believe its use to enable individuals to monitor changes in their dietary habits should be investigated further.
In conclusion, dietary acid–base load was significantly related to urine pH with more alkaline urine associated with a more alkaline dietary load. There was a difference of 4·2 mEq PRAL and two-thirds to just under one portion of fruit and vegetables, in men and women respectively, between the lowest and highest categories of pH. The scale of the associations found was similar to intervention studies. Casual urine pH provides a simple tangible measure of the effects of diet in this population and its use to monitor change in diet quality needs further investigation.
Acknowledgements
A. W. provided the dietary data, performed the statistical analyses and wrote the manuscript. K. T. K. and S. A. B. are principal investigators of the EPIC-Norfolk Study. All of the authors were involved in interpreting the data and contributed to writing the manuscript. None of the authors had any conflict of interest.
EPIC-Norfolk is supported by programme grants from the Medical Research Council UK and Cancer Research UK and through additional support from the European Union, Stroke Association, British Heart Foundation, Department of Health, Food Standards Agency and the Wellcome Trust.