Introduction
Infestation of weeds in agronomic production systems has long been recognized as a major threat to global food security (Blackman and Templeman Reference Blackman and Templeman1938; Weber and Staniforth Reference Weber and Staniforth1957). When selecting weed management programs, growers consider several factors, such as the type of herbicide-resistant crop cultivar, weed control spectrum, selectivity, cost, environment, and fit with conservation agriculture (Buhler Reference Buhler1999; Swanton and Weise Reference Swanton and Weise1991). Conservation agriculture comprises a set of three major components: minimal mechanical soil disturbance, permanent soil organic covers using crop residues or cover crops, and crop rotation (FAO 2017). Conservation agriculture can contribute to sustainable crop production. Development and commercialization of herbicide-resistant crops, primarily glyphosate-resistant (GR) crops, ensured a simplified, flexible, and cost-effective weed management program and promoted conservation agriculture by reducing the deep tillage and maintaining the crop residues on the soil surface (Carpenter and Gianessi Reference Carpenter and Gianessi1999; Dill et al. Reference Dill, CaJacob and Padgette2008; Triplett and Dick Reference Triplett and Dick2008). However, due to the overuse of glyphosate, several recent reports have expressed concerns regarding contamination of glyphosate and its primary metabolite, aminomethylphosphonic acid, in soil, surface water, groundwater, and farm produce in the United States and Europe (Battaglin et al. Reference Battaglin, Meyer, Kuivila and Dietze2014; Bøhn et al. Reference Bøhn, Cuhra, Traavik, Sanden, Fagan and Primicerio2014; Kolpin et al. Reference Kolpin, Thurman, Lee, Meyer, Furlong and Glassmeyer2006; Silva et al. Reference Silva, Montanarella, Jones, Fernández-Ugalde, Mol, Ritsema and Geissen2018).
Multiple applications of glyphosate in a growing season in GR crops imposed intense selection pressure on weeds that resulted in the evolution of GR biotypes. Currently, 42 weed species have been reported as GR globally, among which 17 were reported in the United States (Heap Reference Heap2018b) and 6 were reported in Nebraska (Jhala Reference Jhala2018). The widespread occurrence of GR weeds in the United States compelled growers to consider applying soil-residual herbicides either as PRE or in mixture with foliar-active POST herbicides (Norsworthy et al. Reference Norsworthy, Ward, Shaw, Llewellyn, Nichols, Webster, Bradley, Frisvold, Powles, Burgos, Witt and Barrett2012; Sarangi et al. Reference Sarangi, Sandell, Kruger, Knezevic, Irmak and Jhala2017; Whitaker et al. Reference Whitaker, York, Jordan and Culpepper2010).
Since 2010, demand for non–genetically engineered (non-GE) food products has increased in the United States, with an average growth of 70% each year (Bain and Selfa Reference Bain and Selfa2017). The market for non-GE food in the United States reached US$200 billion in 2014 (Cartwright Reference Cartwright2016). China is the world’s largest producer and importer of conventional (non-GE) soybean (Muhammad Reference Muhammad2015; Wang and Houston Reference Wang and Houston2016), and a majority of the soybean (59% of total exported soybean, including both herbicide-resistant and non-GE) produced in the United States is exported to China, with an export value of US$12.4 billion in 2017 (USDA-APHIS 2018; USDA-FAS 2017). Due to the increasing anti-GE movement and mandatory label requirements in certain regions of the food industry, the market for non-GE soybean in China, Europe, and Japan is likely to expand in the near future (Babcock and Beghin Reference Babcock and Beghin1999; Cartwright Reference Cartwright2016; Davison Reference Davison2010).
The cost of soybean seed has been increasing over the years, with GE soybean seed cost being higher than that of non-GE soybean for having a technology fee. For example, in 2010 the cost of GE soybean seed was 47% higher than the cost of non-GE soybean seed (Benbrook Reference Benbrook2012). When the level of weed control and the herbicide cost are comparable between two systems, Reddy and Whiting (Reference Reddy and Whiting2000) described that the selection of a crop cultivar mostly depends on the yield potential and cost of the seed, including the technology fee. With the depressed corn and soybean prices in recent years in the United States, growers have shown interest in non-GE soybean production for its low seed cost and better after-harvest incentives (Hart and Zhang Reference Hart and Zhang2016; Jones Reference Jones2008). Additionally, the epidemic of GR weeds is rapidly lowering the value of GR corn and soybean cultivars in the Midwest. Benbrook (Reference Benbrook2016) noted that due to an increase in generic glyphosate manufacturing in the United States, glyphosate prices had been going down since 2000; in addition, the report mentioned that the application of glyphosate per unit of land was increasing, which in turn increases the selection pressure and overall production cost. In a survey of soybean growers in South Carolina, Norsworthy (Reference Norsworthy2003) reported that weed management cost in non-GE and GR soybean was similar; however, the additional cost of the technology fee increased the input cost in GR soybean system compared with non-GE soybean. Variety trials assessing the yield potential of GR and non-GE soybean in eight states in the midwestern United States showed that non-GE soybean produced 3% more yield than GR soybean in 1998 to 1999 (Carpenter Reference Carpenter2001). In a 2-yr field research study conducted in Stoneville, MS, Reddy and Whiting (Reference Reddy and Whiting2000) reported that GR and non-GE soybean showed similar yield potential.
Weed control, which is a challenge for the non-GE soybean producers, particularly in no-till or reduced-tillage production systems, has led to limited non-GE soybean acreage in the United States (Reddy Reference Reddy2003; Reddy and Whiting Reference Reddy and Whiting2000; USDA 2015). In 2017, only 6% of soybean area in the United States was planted with non-GE soybean cultivars (USDA-NASS 2017). Palmer amaranth is the most problematic weed in conservation agriculture in the United States (Chahal et al. Reference Chahal, Aulakh, Jugulam and Jhala2015; Price et al. Reference Price, Balkcom, Culpepper, Kelton, Nichols and Schomberg2011). A survey conducted by Sarangi and Jhala (Reference Sarangi and Jhala2018) ranked Palmer amaranth and velvetleaf among the six most problematic weeds in Nebraska. Palmer amaranth biotypes resistant to acetolactate synthase (ALS), hydroxyphenylpyruvate dioxygenase (HPPD), photosystem II (PSII) inhibitors, and glyphosate have been confirmed in Nebraska (Chahal et al. Reference Chahal, Varanasi, Jugulam and Jhala2017; Jhala et al. Reference Jhala, Sandell, Rana, Kruger and Knezevic2014).
Season-long interference of Palmer amaranth at a density of 3.33 and 10 plants m−1 of row reduced soybean yield by 64% and 68%, respectively (Klingaman and Oliver Reference Klingaman and Oliver1994). Similarly, Bensch et al. (Reference Bensch, Horak and Peterson2003) reported that Palmer amaranth interference at a density of 8 plants m−1 of soybean row resulted in 79% yield loss in Kansas. Soybean yield losses due to velvetleaf infestation are inconsistent: for example, Staniforth and Weber (Reference Staniforth and Weber1956) reported no significant soybean yield loss due to 10 to 20 velvetleaf plants m−2, whereas Eaton et al. (Reference Eaton, Russ and Feltner1976) found up to 66% yield loss with the season-long interference of velvetleaf at a density of 130 to 240 plants m−2.
During the last two decades, research has generated sufficient information about weed management in GR soybean; however, information on weed management in no-till non-GE soybean is limited. The objectives of this study were to (1) compare PRE-only, PRE followed by (fb) POST, and PRE fb POST with residual (POST-WR) programs for control of Palmer amaranth and velvetleaf in no-till non-GE soybean; and (2) evaluate the soybean injury, yield, gross profit margin, and benefit–cost ratio in response to different herbicide programs.
Materials and Methods
Study Site
Field experiments were conducted during the summer in 2016 and 2017 at the South Central Agricultural Laboratory of the University of Nebraska–Lincoln located near Clay Center, NE (40.57°N, 98.14°W). The experimental site was infested primarily with Palmer amaranth and velvetleaf. Distribution of common lambsquarters (Chenopodium album L.), waterhemp [Amaranthus tuberculatus (Moq.) J. D. Sauer], large crabgrass [Digitaria sanguinalis (L.) Scop.], and foxtails (Setaria spp.) was not uniform at this study site; therefore, data from these weed species were not included. No herbicide-resistant weeds apart from ALS inhibitor–resistant Palmer amaranth and waterhemp had been reported at the site. A natural seedbank of Palmer amaranth and velvetleaf was used for this study. The soil at the experiment site was a Crete silt loam (fine, montmorillonite, mesic Pachic Argiustoll) with 17% sand, 58% silt, 25% clay, 3% organic matter, and a pH of 6.5.
Treatments and Plots
The research site was under a continuous no-till corn–soybean rotation for the last 7 yr. Crop residue from the previous cropping season was retained, as the no-till system was practiced in this study. The experimental site was fertilized with 202 kg ha−1 of nitrogen in the form of anhydrous ammonia applied in the early spring with additional 11-52-0 fertilizer at 112 kg ha−1 at planting. A blanket application (preplant treatment) of paraquat (Gramoxone® SL, Syngenta Crop Protection, Greensboro, NC 24719; at 0.84 kg ai ha−1) plus a nonionic surfactant (Induce®, Helena Chemical, Collierville, TN 38017; at 0.25% v/v) was made in the spring (2 wk before soybean planting) using a tractor-mounted sprayer calibrated to deliver 140 L ha−1. A non-GE soybean cultivar (‘U11-622148 BR-16’) was planted in no-till conditions on May 12 in 2016 and April 25 in 2017 at 322,000 seeds ha−1 to a depth of 3 cm. The experimental site was under a center-pivot irrigation system. The experiment was arranged in a randomized complete block design with four replications. Three weed management programs (PRE-only, PRE fb POST, and PRE fb POST-WR) comprised 15 herbicide treatments, with a nontreated control (Table 1). The plots were 3-m wide by 9-m long, where 4 soybean rows were spaced 76.2 cm apart.
Table 1 Herbicide programs, application timing, and rates used for control of Palmer amaranth and velvetleaf in no-till non-GE soybean in Nebraska.
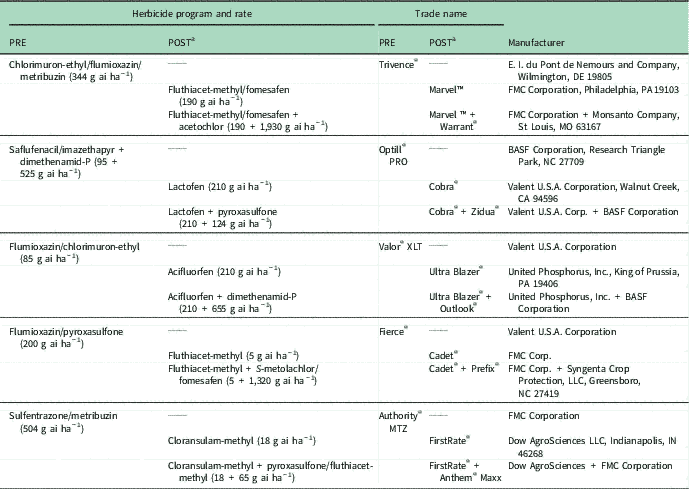
a Crop oil concentrate (Agri-Dex, Helena Chemical Company, Collierville, TN 38017; at 1% v/v) and ammonium sulfate (N-Pak AMS Liquid, Winfield Solutions, LLC, St Paul, MN 55164; at 2.5% v/v) were mixed with the POST herbicide treatments.
The PRE applications were made within 2 d of soybean planting, and POST treatments were applied on June 20 in 2016 and June 9 in 2017, when the average plant height was 8 to 12 cm for Palmer amaranth, 12 cm for velvetleaf, and soybean was at the V3 to V4 stage. Herbicides were applied using a handheld CO2-pressurized backpack sprayer equipped with AIXR 110015 flat-fan nozzles (TeeJet® Technologies, Spraying Systems, P.O. Box 7900, Wheaton, IL 60187) calibrated to deliver 140 L ha−1 at 276 kPa at a constant speed of 4.8 km h−1.
Data Collection
Palmer amaranth and velvetleaf control was visually assessed at 14 and 28 d after PRE (DAPRE), 14 and 28 d after POST (DAPOST), and at soybean harvest on a scale of 0% to 100%, with 0% representing no control and 100% representing complete control. Weed density was recorded at 14 and 28 DAPRE and 14 and 28 DAPOST by counting Palmer amaranth and velvetleaf in two 0.25-m2 quadrats placed randomly between the two center soybean rows in each plot and was converted into number of plants per square meter. Palmer amaranth plants surviving herbicide treatments were cut at the soil surface at 56 DAPOST from two randomly selected 0.25-m2 quadrats, and the samples were put into paper bags and placed in an oven at 65 C for 5 d. Aboveground biomass for velvetleaf was not collected in this study. Palmer amaranth aboveground biomass data were converted into percent biomass reduction compared with the nontreated control using the equation:

where C is the aboveground biomass of the nontreated control plot and B is the biomass of an individual treated plot.
Soybean injury was assessed visually at 14 DAPRE, 7 DAPOST, and 21 DAPOST on a scale of 0% to 100% (0% representing no injury and 100% representing complete death) based on leaf chlorosis and necrosis, malformation of leaves, purpling of the veins, and plant stunting. Soybean plant stand was counted at 28 DAPRE from a randomly selected 1-m length of each of the center two rows per plot. Soybean was harvested from the center two rows in each plot using a plot combine (Gleaner K2, AGCO, 4205 River Green Parkway, Duluth, GA 30096), and the grain yield was adjusted to 13% moisture content.
An economic analysis was performed to assess the profitability of the herbicide programs. Gross profit margin was calculated using the equation:

where R is the gross revenue calculated by multiplying soybean yield by the average grain price received at harvest in 2017 (US$0.38 kg−1; USDA-AMS 2017) and W is the weed management cost, including the cost of herbicides, adjuvants, and custom applications (US$18.11 ha−1 application−1; Barnes et al. Reference Barnes, Knezevic, Sikkema, Lindquist and Jhala2017). Herbicide prices were obtained from three independent commercial sources (Cargill, Country Partners Cooperative, Crop Production Services) in Nebraska, and were averaged and used to calculate the herbicide cost per hectare.
The benefit–cost ratio indicates the value of the money spent on a herbicide program. It summarizes the rate of return (US$) per unit capital (US$) invested for weed management. Benefit–cost ratio was calculated using the equation:

where R T is the gross revenue of a herbicide program, R C is the gross revenue for the nontreated control, and W is the weed management cost.
Statistical Analysis
Palmer amaranth and velvetleaf control, their density, aboveground biomass reduction, and soybean injury and yield data were subjected to ANOVA using PROC GLIMMIX in SAS (SAS Institute, Cary, NC). Before ANOVA, all data were tested for the normality and homogeneity of error variances using the Shapiro-Wilk test and Bartlett’s test, respectively. Visual estimates of weed control and biomass reduction data were arc-sine square-root transformed before analysis; however, back-transformed data are presented with the means separated using Fisher’s protected LSD test, where α = 0.05. In the model, treatments and years were considered fixed effects, whereas blocks were considered random effects. To determine the relative efficacy of the herbicide programs (PRE-only vs. PRE fb POST; and PRE fb POST vs. PRE fb POST-WR) for Palmer amaranth and velvetleaf control, density, and aboveground biomass reduction, along with soybean injury and yield, a priori orthogonal contrasts (single degree of freedom contrasts) were performed.
Results and Discussion
Year-by-treatment interactions for weed control, density, and aboveground biomass reduction were nonsignificant (P ≥ 0.05); therefore, data from 2016 and 2017 were combined. Rainfall within 30 DAPRE applications and throughout the season was sufficient for the activity of the soil-applied residual herbicides (Table 2).
Table 2 Average air temperature and total precipitation during the 2016 and 2017 growing seasons and the 30-yr average at South Central Agricultural Laboratory of the University of Nebraska–Lincoln, Clay Center, Nebraska.Footnote a
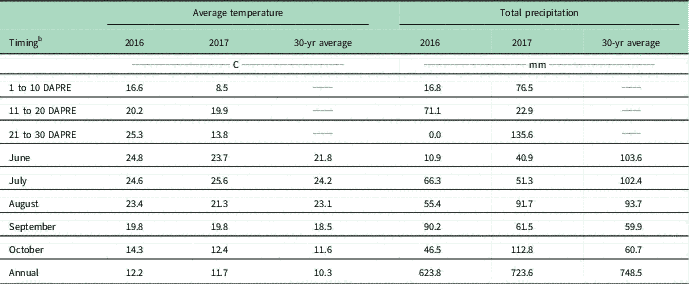
a Air temperature and precipitation data were obtained from HPRCC, the High Plains Regional Climate Center (2017).
b DAPRE, days after PRE herbicide application.
Palmer Amaranth Control, Density, and Biomass Reduction
The PRE herbicides tested in this study controlled Palmer amaranth 97% to 100% at 14 and 28 DAPRE and reduced weed density to ≤1 plant m−2 compared with 32 plants m−2 in nontreated control at 14 DAPRE (Table 3). Similarly, field studies conducted in Kansas showed that chlorimuron-ethyl/flumioxazin/metribuzin, saflufenacil/imazethapyr plus dimethenamid-P, flumioxazin/pyroxasulfone, and sulfentrazone/metribuzin controlled Palmer amaranth ≥97% at 14 DAPRE in soybean (Hay Reference Hay2017). A study conducted in soybean fields in five midwestern and southern states including Nebraska showed that flumioxazin/pyroxasulfone controlled Palmer amaranth ≥95% at all sites at 28 DAPRE (Meyer et al. Reference Meyer, Norsworthy, Young, Steckel, Bradley, Johnson, Loux, Davis, Kruger, Bararpour, Ikley, Spaunhorst and Butts2015). Additionally, Sarangi et al. (Reference Sarangi, Sandell, Kruger, Knezevic, Irmak and Jhala2017) reported that saflufenacil/imazethapyr plus dimethenamid-P, flumioxazin/chlorimuron-ethyl, and flumioxazin/pyroxasulfone resulted in 92% to 97% control of GR waterhemp, a species closely related to Palmer amaranth, in GR soybean in Nebraska. Ward et al. (Reference Ward, Webster and Steckel2013) emphasized that PRE-applied residual herbicides are the cornerstone for early-season Palmer amaranth control in soybean.
Table 3 Palmer amaranth and velvetleaf control and density as affected by PRE herbicide treatments in no-till non-GE soybean in Nebraska.Footnote a ,Footnote b
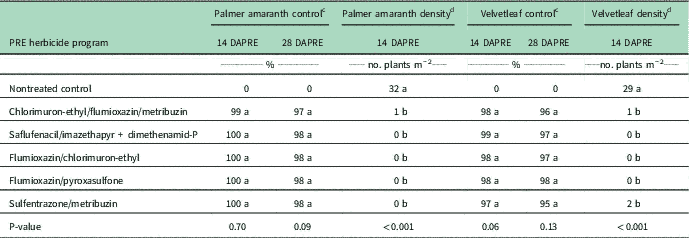
a Abbreviation: DAPRE, d after PRE herbicide application.
b Data presented in this table were pooled across both years (2016 and 2017).
c Data were arc-sine square-root transformed before analysis; however, back-transformed original mean values are presented based on the interpretation of the transformed data, and the weed control data for the nontreated control plots were not included in the analysis.
d Means presented within each column with no common letter(s) are significantly different according to Fisher’s protected LSD, where α = 0.05.
Averaged across herbicide programs, PRE fb POST programs controlled Palmer amaranth 95% at 14 DAPOST compared with 76% control with PRE-only programs (Table 4). The PRE fb POST-WR programs controlled Palmer amaranth 99% at 14 DAPOST. Due to a decline in the residual activities of the PRE soil-applied herbicides, PRE-only programs resulted in 66% control of Palmer amaranth at 28 DAPOST compared with 86% control for PRE fb POST programs (Table 4). Most of the PRE fb POST-WR programs resulted in ≥97% control of Palmer amaranth at 28 DAPOST. Similarly, Chahal et al. (Reference Chahal, Ganie and Jhala2018) reported that mixing soil-residual herbicides with foliar-active POST herbicides controlled HPPD and PSII inhibitor–resistant Palmer amaranth season-long in Nebraska. Averaged across programs, PRE-only herbicide programs reduced Palmer amaranth density by 67% at 28 DAPOST compared with the nontreated control (36 plants m−2 with PRE-only programs vs. 110 plants m−2 in nontreated control; Table 4). The PRE fb POST-WR programs resulted in 3 Palmer amaranth plants m−2 compared with 14 plants m−2 in PRE fb POST programs. Due to the availability of sufficient soil moisture early in the season in 2017, Palmer amaranth emergence was more than two times higher in 2017 compared with 2016. Palmer amaranth density was 48 to 76 plants m−2 in 2016 compared with 108 to 220 plants m−2 in 2017 in nontreated control (data not shown).
Table 4 Palmer amaranth control, density, and aboveground biomass reduction as affected by herbicide programs in no-till non-GE soybean in Nebraska.Footnote a ,Footnote b
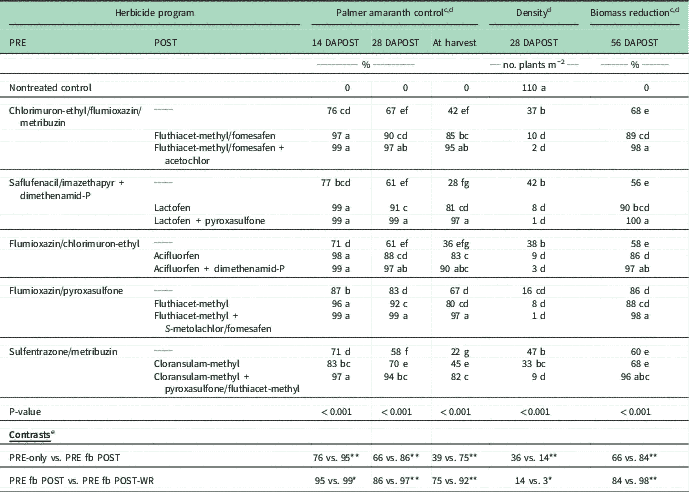
a Abbreviations: DAPOST, d after POST herbicide application; POST-WR, POST with residual.
b Data presented in this table were pooled across both years (2016 and 2017).
c Data were arc-sine square-root transformed before analysis; however, back-transformed original mean values are presented based on the interpretation of the transformed data.
d Means presented within each column with no common letter(s) are significantly different according to Fisher’s protected LSD, where α = 0.05.
e A priori orthogonal contrasts; ** = significant (P < 0.01) and * = significant (P < 0.05).
PRE fb POST-WR herbicide programs including saflufenacil/imazethapyr plus dimethenamid-P fb lactofen plus pyroxasulfone, and flumioxazin/pyroxasulfone fb fluthiacet-methyl plus S-metolachlor/fomesafen resulted in 97% control of Palmer amaranth at harvest, which was comparable to the control obtained with chlorimuron-ethyl/flumioxazin/metribuzin fb fluthiacet-methyl/fomesafen plus acetochlor (95% control), and flumioxazin/chlorimuron-ethyl fb acifluorfen plus dimethenamid-P (90% control) (Table 4). Orthogonal contrasts showed that PRE fb POST herbicide programs resulted in higher Palmer amaranth control (75%) than PRE-only programs (39% control) at harvest and that PRE fb POST-WR programs improved Palmer amaranth control over PRE fb POST programs (92% vs. 75% control). In a statewide survey of growers and extension agents in Georgia, Sosnoskie and Culpepper (Reference Sosnoskie and Culpepper2014) reported that growers often use overlapping residual herbicides to obtain season-long Palmer amaranth control in cotton (Gossypium hirsutum L.). Data on reduction in aboveground biomass followed similar trends as Palmer amaranth control and density (Table 4). The PRE fb POST-WR programs resulted in higher biomass reduction (96% to 100%) compared with other herbicide programs.
Several studies reported improved Palmer amaranth control by PRE fb POST herbicide programs in soybean (Butts et al. Reference Butts, Norsworthy, Kruger, Sandell, Young, Steckel, Loux, Bradley, Conley, Stoltenberg, Arriaga and Davis2016; Meyer et al. Reference Meyer, Norsworthy, Young, Steckel, Bradley, Johnson, Loux, Davis, Kruger, Bararpour, Ikley, Spaunhorst and Butts2015; Whitaker et al. Reference Whitaker, York, Jordan and Culpepper2010), though it is expected that the extended emergence period of Palmer amaranth will allow later-emerging cohorts to escape in-crop POST treatments. Neve et al. (Reference Neve, Norsworthy, Smith and Zelaya2011) proposed that mixing foliar-active POST herbicides with soil-residual herbicides can provide season-long control of Palmer amaranth in row crops. To achieve residual control of Palmer amaranth later in the season, very-long-chain fatty acid (VLCFA)-inhibiting herbicides (such as acetochlor, dimethenamid-P, pyroxasulfone, and S-metolachlor) were applied POST in this study along with foliar-active herbicides (ALS and protoporphyrinogen oxidase [PPO] inhibiting). The VLCFA-inhibiting herbicides are known for the residual control of small-seeded broadleaf weed species, including Amaranthus spp. (Geier et al. Reference Geier, Stahlman and Frihauf2006; Grey et al. Reference Grey, Cutts, Newsome and Newell2014; Hay Reference Hay2017; Sarangi et al. Reference Sarangi, Sandell, Knezevic, Irmak and Jhala2015b, Reference Sarangi, Sandell, Kruger, Knezevic, Irmak and Jhala2017).
Velvetleaf Control and Density
The PRE herbicides tested in this study controlled velvetleaf 95% or greater at 14 and 28 DAPRE and resulted in 0 to 2 velvetleaf plants m−2 at 14 DAPRE (Table 3). Similarly, Peterson et al. (Reference Peterson, Thompson and Minihan2017) reported 94% to 100% control of velvetleaf at 18 DAPRE with flumioxazin/chlorimuron-ethyl, flumioxazin/pyroxasulfone, and sulfentrazone/metribuzin in a field study conducted in Manhattan, KS. Mahoney et al. (Reference Mahoney, Shropshire and Sikkema2014) also reported that PRE application of flumioxazin/pyroxasulfone at 200 g ai ha−1 in no-till soybean controlled velvetleaf 94% at 28 DAPRE in Ontario, Canada.
The PRE fb POST herbicide programs substantially improved velvetleaf control (98%) over the PRE-only herbicide programs (89% control) at 14 DAPOST (Table 5). However, averaged across herbicide programs, velvetleaf control was similar in PRE fb POST-WR and PRE fb POST programs at 14 and 28 DAPOST. The majority of soil-residual herbicides included in POST herbicide programs were VLCFA inhibitors, and these herbicides showed partial velvetleaf control (Anonymous 2016; Belfry et al. Reference Belfry, McNaughton and Sikkema2015; Robinson et al. Reference Robinson, McNaughton and Soltani2008). Therefore, the selection of residual herbicides for POST application should be based on the weed species present in the field. Velvetleaf density data at 28 DAPOST followed a similar trend as velvetleaf control, and PRE fb POST programs considerably reduced velvetleaf density to 4 plants m−2, while velvetleaf density was 10 and 45 plants m−2 in the PRE-only programs and nontreated control, respectively (Table 5).
Table 5 Velvetleaf control and density as affected by herbicide programs in no-till non-GE soybean in Nebraska.Footnote a ,Footnote b

a Abbreviations: DAPOST, d after POST herbicide application; POST-WR, POST with residual.
b Data presented in this table were pooled across both years (2016 and 2017).
c Data were arc-sine square-root transformed before analysis; however, back-transformed original mean values are presented based on the interpretation of the transformed data.
d Means presented within each column with no common letter(s) are significantly different according to Fisher’s protected LSD, where α = 0.05.
c A priori orthogonal contrasts; ** = significant (P < 0.01); NS, nonsignificant (P ≥ 0.05).
Soybean Injury and Yield
At 14 DAPRE, soybean injury caused by PRE herbicides was minimal. No difference in soybean stand (P = 0.38) was also observed at 28 DAPRE (Table 6). However, at 7 DAPOST, 8% to 12% soybean injury was observed with all POST herbicides, although, injury symptoms were transitory and dissipated at 21 DAPOST (data not shown). The POST application of PPO-inhibiting herbicides (acifluorfen, fomesafen, and lactofen) is known to cause a low to moderate level of soybean injury (Sarangi and Jhala 2015). However, several studies have reported that low levels of herbicide injury do not impact soybean yield (Legleiter et al. Reference Legleiter, Bradley and Massey2009; Patton Reference Patton2013; Riley and Bradley Reference Riley and Bradley2014; Sarangi et al. Reference Sarangi, Sandell, Kruger, Knezevic, Irmak and Jhala2017).
Table 6 Soybean stand count and yield as affected by herbicide programs in no-till non-GE soybean in Nebraska.Footnote a

a Abbreviations: DAPRE, d after PRE herbicide application; POST-WR, POST with residual.
b Means presented within each column with no common letter(s) are significantly different according to Fisher’s protected LSD, where α = 0.05.
c Year-by-treatment interaction was significant for soybean yield; therefore, data from both years are presented separately.
d A priori orthogonal contrasts; ** = significant (P < 0.01), and * = significant (P < 0.05).
The year-by-treatment interaction was significant for soybean yield; therefore, yield data from 2016 and 2017 are presented separately. It is believed that the higher Palmer amaranth density in 2017 led to the lower soybean yield in all the treatments and nontreated control. Averaged across herbicide programs, PRE fb POST programs resulted in higher soybean yield (3,905 and 1,680 kg ha−1 in 2016 and 2017, respectively) compared with the PRE-only herbicide programs (3,552 and 1,192 kg ha−1 in 2016 and 2017, respectively) (Table 6). The PRE fb POST-WR program improved soybean yield by 7% and 40% in 2016 and 2017, respectively, over the PRE fb POST program. Therefore, it is evident that if Palmer amaranth is the major weed in a no-till non-GE soybean field, herbicide strategies that include overlapping soil-residual herbicides as tested in this study may result in season-long control and higher soybean yield. Whitaker et al. (Reference Whitaker, York, Jordan and Culpepper2010) noted that PRE fb POST/POST-WR herbicide programs are necessary for effective management of Palmer amaranth and higher soybean yield. Moreover, Chahal et al. (Reference Chahal, Ganie and Jhala2018) showed that inclusion of soil-residual herbicides in POST treatments increased corn yield and net economic returns. Butts et al. (Reference Butts, Norsworthy, Kruger, Sandell, Young, Steckel, Loux, Bradley, Conley, Stoltenberg, Arriaga and Davis2016) also reported that a PRE fb POST/POST-WR herbicide strategy is an important component in integrated weed management that can effectively manage Amaranthus spp. and increase grain yield. Studies conducted in Nebraska also showed that PRE fb POST/POST-WR herbicide programs resulted in higher waterhemp control and soybean yield in GR and glufosinate-resistant soybean (Jhala et al. Reference Jhala, Sandell, Sarangi, Kruger and Knezevic2017; Sarangi et al. Reference Sarangi, Sandell, Kruger, Knezevic, Irmak and Jhala2017).
Economics
Weed management costs for the PRE fb POST and PRE fb POST-WR herbicide programs ranged between US$104.8 and US$205.9 ha−1 (Table 7). Gross profit margins with PRE fb POST-WR programs varied from US$895.3 to US$1,184.3 ha−1, whereas for the PRE fb POST programs, gross profit margins ranged from US$857.5 to US$972.4 ha−1. The results of the benefit–cost ratio analysis showed that greater economic benefit was obtained with the PRE-only program of flumioxazin/chlorimuron-ethyl (benefit–cost ratio = 6.1), flumioxazin/pyroxasulfone (5.6), and chlorimuron/flumioxazin/metribuzin (5.5) due to the lower weed management cost (Table 7); however, the PRE-only programs resulted in poor control of Palmer amaranth (an average of 39% control at harvest) and velvetleaf (85% control at 28 DAPOST) compared with the PRE fb POST/ POST-WR programs. Therefore, application of POST herbicides following a PRE treatment is recommended for long-term sustainable weed management and to reduce the weed seedbank in the soil. In this study, flumioxazin/pyroxasulfone fb fluthiacet-methyl plus S-metolachlor/fomesafen resulted in the highest gross profit margin (US$1,184.3) and a benefit–cost ratio of 5.2.
Table 7 Gross profit margin and benefit–cost ratio of herbicide programs for control of Palmer amaranth and velvetleaf in no-till non-GE soybean in Nebraska.

a Gross revenue was calculated by multiplying average soybean yield (from 2016 and 2017) with the average grain price for non-GE soybean at harvest in 2017 (US$0.38 kg−1).
b Weed management cost included the cost of herbicides, adjuvants, and custom applications (US$18.11 ha−1 application−1); herbicide price was averaged from three independent commercial sources in Nebraska.
c Gross profit margin was calculated as gross revenue minus weed management cost.
d Benefit–cost ratio for a program (US$/US$) = (gross revenue of a program – gross revenue of nontreated control)/ weed management cost.
Practical Implications
Weed management in no-till non-GE soybean is mostly herbicide dependent; therefore, herbicide programs should be selected carefully to provide season-long weed control and high soybean yield. Unlike GR or glufosinate-resistant soybean systems, there are few “rescue” POST herbicides available in non-GE soybean, meaning that a PRE herbicide application is even more critical. In this study, PRE fb POST-WR programs controlled Palmer amaranth 82% to 99% throughout the season and resulted in higher soybean yields (4,194 and 2,349 kg ha−1 in 2016 and 2017, respectively) compared with the PRE-only and PRE fb POST programs. The PRE fb POST herbicide programs controlled velvetleaf 94% at 28 DAPOST, which was similar to the control obtained with the PRE fb POST-WR programs. Although the inclusion of soil-residual herbicides in PRE fb POST programs slightly increased gross profit margins and mostly did not improve the benefit–cost ratio compared with the PRE fb POST programs, this weed management approach is important for season-long management of Amaranthus spp. such as waterhemp and Palmer amaranth. A recent report by USDA-APHIS (2018) stated that inspection officials in China, the largest importer of U.S.-grown soybean (GE and non-GE), have detected considerable weed seed contamination in soybean shipments from the United States, with 80% of those seeds coming from four major weeds, including Amaranthus spp. Therefore, to ensure uninterrupted soybean trade with China, it is essential to control Palmer amaranth season-long to reduce seed contamination in soybean.
POST broadleaf weed control in non-GE soybean is often achieved through ALS- and PPO-inhibiting herbicides. However, ALS inhibitor–resistant Amaranthus spp. and horseweed (Erigeron canadensis L.) are widespread in Nebraska (Jhala Reference Jhala2018; Lawrence Reference Lawrence2017; Sarangi and Jhala Reference Sarangi and Jhala2018; Sarangi et al. Reference Sarangi, Sandell, Knezevic, Aulakh, Lindquist, Irmak and Jhala2015a), and recently a waterhemp biotype from southeast Nebraska has been confirmed resistant to PPO inhibitors (Heap Reference Heap2018a; Stephens et al. Reference Stephens, Sarangi and Jhala2017). Therefore, overlapping residual herbicides not only can provide season-long control of Palmer amaranth, but also can include an additional site of action (if not used previously) in the herbicide program that will mitigate/reduce the selection pressure associated with a single herbicide or site of action.
Acknowledgments
The authors would like to thank George L. Graef, soybean breeder and geneticist at the University of Nebraska–Lincoln, for providing the non-GE soybean seed for this research. We also appreciate the help of Adam Leise, Ian Rogers, and Irvin Schleufer in this project. We further acknowledge the USDA-NIFA-funded Nebraska Extension Implementation Program for supporting this project. No conflicts of interest have been declared.