Clinical studies have indicated that diet is an important factor in the onset and development of chronic human disease including cancer, CVD, insulin resistance and obesity( 1 ). Ruminant-derived foods are a significant source of fat in the human diet( Reference Hulshof, van Erp-Baart and Anttolainen 2 ), and therefore there has been substantial interest in altering the fatty acid (FA) composition of ruminant-derived foods to lower the incidence of chronic diseases( Reference Shingfield, Bonnet and Scollan 3 ). Dietary supplements of oilseeds and plant oils are effective in lowering the concentrations of medium-chain saturates and increasing cis-9-18 : 1 and PUFA in milk( Reference Shingfield, Bonnet and Scollan 3 ), changes that are more aligned with public health recommendations, but often induce milk fat depression (MFD) in lactating cows fed low fibre high-concentrate diets( Reference Griinari, Dwyer and McGuire 4 – Reference Rico and Harvatine 7 ).
Several theories have been proposed to explain diet-induced MFD( Reference Bauman and Griinari 8 ), that is characterised by decreases in milk fat within a few days, with little or no change in the secretion of milk protein and lactose( Reference Bauman and Griinari 9 – Reference Harvatine, Boisclair and Bauman 11 ). Of these, the biohydrogenation theory of diet-induced MFD( Reference Bauman and Griinari 8 ) is the most widely accepted, which attributes the causal mechanism to changes in ruminal lipid metabolism leading to the formation of specific biohydrogenation intermediates that directly inhibit milk fat synthesis. Trans-10, cis-12-CLA formed during the isomerisation of 18 : 2n-6 in the rumen( Reference Wallace, McKain and Shingfield 12 ) is the only intermediate shown unequivocally to inhibit milk fat synthesis in lactating cows( Reference Baumgard, Corl and Dwyer 13 ). However, increases in milk fat trans-10, cis-12-CLA concentrations on diets causing MFD are often lower than would be expected based on the observed enrichment in milk fat to post-ruminal trans-10, cis-12-CLA infusion, suggesting other biohydrogenation intermediates or other mechanisms may also be involved( Reference Shingfield and Griinari 10 , Reference Glasser, Ferlay and Doreau 14 ). Studies involving abomasal infusion of a mixture of FA have provided evidence to suggest that cis-10, trans-12-CLA( Reference Sæbø, Sæbø and Griinari 15 ) and trans-9, cis-11-CLA( Reference Perfield, Lock and Griinari 16 ) may also inhibit milk fat synthesis in the lactating cow. Even though inclusion of plant oils in high-concentrate low-fibre diets typically causes MFD( Reference Griinari, Dwyer and McGuire 4 – Reference Roy, Ferlay and Shingfield 6 ) direct measurements of biohydrogenation intermediates formed in the rumen of lactating cows under these circumstances are limited( Reference Loor, Ueda and Ferlay 17 ).
In the present investigation, cows were fed a high- or low-concentrate diet containing no additional lipid or sunflower oil (SO) to test the hypothesis that increases in ruminal synthesis of CLA isomers with known or putative anti-lipolytic activity explain the decrease in milk fat synthesis on a diet causing MFD. Experimental treatments were formulated to cause varying effects on ruminal lipid metabolism and milk fat synthesis allowing specific alterations in the formation of biohydrogenation intermediates and end products associated with a low fibre high-oil diet causing MFD to be identified. In addition to the detailed characterisation of ruminal biohydrogenation intermediates, the effect of experimental treatments on rumen fermentation, nutrient utilisation, enteric methane production and their relation to rumen microbiome, milk fat quality and mammary transcriptome were evaluated but results are not presented here.
Methods
Cows, experimental design and treatments
All experimental procedures were approved by the National Animal Ethics Committee (approval no. ESAVI/794/04.10.03/2011) in accordance with the guidelines established by the European Community Council Directive 86/609/EEC( 18 ). Four multiparous Finnish Ayrshire cows (89 (sem 11·8) d postpartum and 691 (sem 34·8) kg live weight) fitted with rumen cannulae (i.d. 100 mm; Bar Diamond, Inc.) were used in a 4×4 Latin square with a 2×2 factorial arrangement of treatments with 35-d experimental periods. Each period consisted of a 14-d adaptation, 12-d sample collection interval and 9-d washout. Treatments comprised isonitrogenous diets based on grass silage (forage:concentrate (FC) ratio 65:35 and 35:65 on a DM basis, respectively) containing either 0 (treatments low-concentrate diet containing no added oil (L) and high-concentrate diet containing no added oil (H), respectively) or high- or low-concentrate diet containing 50 g/kg diet DM of SO (treatments HSO and LSO, respectively). SO (Tuko Logistics Ltd) replaced concentrate ingredients (Table 1). From day 27 until the end of each 35-d period all cows were fed the L diet to minimise treatment carry-over effects and restore milk fat yield to pre-treatment levels. All experimental diets were offered ad libitum as a total mixed ration and fed in four equal amounts at 06.00, 09.00, 16.30 and 19.30 hours. Diets were prepared as total mixed rations to avoid selection of dietary components and maintain the target FC ratio. Cows were housed in individual tie stalls within a dedicated metabolism unit, with free access to water and a salt block, and milked at 07.00 and 16.45 hours.
Table 1 Formulation and chemical composition of experimental dietsFootnote *

L, low-concentrate diet (forage:concentrate (FC) ratio 65:35 on a DM basis) with no additional lipid; LSO, low-concentrate diet (FC ratio 65:35) containing 50 g sunflower oil/kg diet DM; H, high-concentrate diet (FC ratio 35:65) with no additional lipid; HSO, high-concentrate diet (FC ratio 35:65) containing 50 g sunflower oil/kg diet DM.
* Values are means of n 4 determinations.
† Restrictively fermented grass silage prepared from the primary growth of mixed timothy (Phleum pratense) and meadow fescue (Festuca pratensis) swards (54:46, respectively), grown at Jokioinen (60°49’N, 23°28’E) treated with a formic acid-based ensiling additive (0·76 formic acid and 0·055 ammonium formate, AIV 2 Plus; Valio Ltd). Mean fermentation characteristics pH 3·95; in DM (g/kg); lactic acid 63·5, acetic acid 22·1, propionic acid 0·18, formic acid 19·1 and water-soluble carbohydrate 27·0, soluble N (g/kg total N) 634 and ammonium N (g/kg total N) 63·9. Grass silage contained 228 g/kg DM (as fed).
‡ Prepared from rapeseed containing low glucosinolate concentrations (Avena Nordic Grain Ltd).
§ Urea (Sigma-Aldrich).
|| Sunflower oil containing (g/100 g total fatty acids) 16 : 0 (6·14), 18 : 0 (3·91), cis-9-18 : 1 (27·9), cis-11-18 : 1 (0·66) and cis-9, cis-12-18 : 2 (59·1) as major components (Tuko Logistics Ltd).
¶ Premix (Onni, Melica Finland Ltd) declared as containing (g/kg) Ca (190), Mg (60), Na (135), Zn (2·19), Mn (0·45), Cu (0·40); (mg/kg), I (55), Co (35), Se (30), dl-α-tocopherol (550), retinol (66) and cholecalciferol (1).
** DM content (g/kg fresh weight) of L, LSO, H and HSO treatments 474, 479, 668 and 672, respectively.
Sampling and chemical analysis
Intake of all cows was measured daily. Representative samples of grass silage, total mixed rations and feed refusals were collected daily from day 21 to day 25 of each experimental period, composited within each period for each cow and stored at −20°C. The chemical composition of experimental diets was determined using standard methods( Reference Shingfield, Jaakkola and Huhtanen 19 ). Samples of SO were collected over the same interval and analysed for FA content and composition. Daily milk yields of all cows were recorded throughout the experiment, but only measurements from day 22 to day 25 of each experimental period were used for the statistical analysis. Milk samples were collected at each milking, from day 22 to day 25, preserved with Bronopol (Valio Ltd) until milk fat, crude protein (CP) and lactose were predicted by IR spectroscopy (Milko-Scan 133B; Foss Electric). Near IR detection of milk constituents was calibrated using milk samples for which reference measurements had previously been made.
The flow of digesta at the omasum canal was determined using the omasal sampling technique( Reference Ahvenjärvi, Vanhatalo and Huhtanen 20 ) and a triple indigestible marker system using Cr-EDTA, Yb-acetate and indigestible neutral detergent fibre (iNDF) as markers for liquid, small and large particulate phases, respectively( Reference Shingfield, Ärölä and Ahvenjärvi 21 ). Cr-EDTA (1000 g) prepared according to standard methods( Reference Binnerts, van’t Klooster and Frens 22 ) and Yb-acetate (5·0 g) obtained from a commercial source (DKSH Nordic A/S) were dissolved in 6 litres of distilled water and infused separately into the rumen at a constant rate (4·2 ml/min) using a peristaltic pump (Watson-Marlow) starting at 15.00 hours on day 18 of each period. To facilitate rapid equilibration of marker concentrations in the rumen, cows also received priming doses of Cr-EDTA (1500 g/d) and Yb-acetate (7·5 g/d) at the start of marker administration.
Spot samples (500 ml) of digesta entering the omasal canal were collected four times daily at 3-h intervals from day 22 to day 24. Sampling started at 06.00 hours and was advanced 1 h each day to cover a 12-h period that was considered representative of the entire feeding cycle. After each sampling, digesta was immediately stored at −20°C. At the end of the study, samples of digesta were thawed at 20°C, composited for each cow for each period and separated into large particle, small particle and liquid fractions by filtration and centrifugation( Reference Ahvenjärvi, Vanhatalo and Huhtanen 20 ). Each phase was freeze-dried and stored at −20°C, whereas subsamples of each fraction for FA analysis were stored at −80°C.
Marker concentrations and chemical composition of omasal digesta were determined using standard methods( Reference Ahvenjärvi, Vanhatalo and Huhtanen 20 ). Digesta flow entering the omasal canal was calculated after mathematical reconstruction according to a triple marker method( Reference Ahvenjärvi, Vanhatalo and Huhtanen 20 ). Marker administration was based on the amounts excreted in faeces. Thereafter, appropriate amounts of freeze-dried digesta fractions were weighed to provide a 10-g composite sample before FA analysis.
Faecal marker excretion was determined by total collection performed over 96 h starting at 18.00 hours on day 21 of each experimental period. Urine was separated from faeces by means of a light harness and flexible tubing attached to the vulva. Concentrations of iNDF, Cr and Yb in faeces were determined using the same methods applied to omasal digesta( Reference Ahvenjärvi, Vanhatalo and Huhtanen 20 ).
Lipid analysis
Fatty acid methyl esters (FAME) of lipid in SO and freeze-dried samples of silage and concentrates were prepared in a one-step extraction-transesterification procedure using chloroform and 2 % (v/v) sulfuric acid in methanol( Reference Shingfield, Ahvenjärvi and Toivonen 23 ). Feed FA content was determined using tritridecanoin (T-135; Nu-Chek-Prep Inc.) as an internal standard and tripalmitin (T-5888; Sigma-Aldrich) as an external standard( Reference Shingfield, Ahvenjärvi and Toivonen 23 ). Following the addition of an internal standard (tridecanoic acid, N-13A; Nu-Chek-Prep Inc.), the pH of omasal digesta was adjusted to 2·0 using 2 mol/l hydrochloric acid( Reference Shingfield, Ahvenjärvi and Toivonen 23 ). Lipid in omasal digesta was extracted with a mixture (3:2; v/v) of hexane and isopropanol and FAME were prepared by a two-step base-acid catalysed procedure using sodium methoxide and 2 % (v/v) sulfuric acid in methanol as catalysts( Reference Halmemies-Beauchet-Filleau, Kairenius and Ahvenjärvi 24 ).
FAME were quantified using a gas chromatograph (model 6890N; Agilent Technologies) fitted with a CP-Sil 88 column (100 m×0·25 mm i.d., 0·2 µm film thickness; Agilent Technologies) and flame ionization detector. The total FAME profile in a 2-µl sample volume at a split ratio of 1:50 was determined using a temperature gradient( Reference Shingfield, Ahvenjärvi and Toivonen 23 ) and H2 as the carrier gas operated at constant pressure (206·8 kPa) and nominal initial flow rate of 2·1 ml/min. Individual isomers of 18 : 1 were further resolved in a separate analysis under isothermal conditions at 170°C( Reference Shingfield, Ahvenjärvi and Toivonen 23 ). Under these conditions trans-10, cis-15-18 : 2 and trans-11, cis-15-18 : 2 eluted as a single peak. To resolve these isomers, GC analysis was repeated using a SLB-IL111 column (100 m×0·25 mm i.d., 0·2 µm film thickness; Sigma-Aldrich) and He as a carrier gas (nominal initial flow rate of 1·0 ml/min) at constant pressure 264·8 kPa and a temperature gradient (initial oven temperature of 168°C maintained for 30 min, increased at a rate of 1°C/min to a final temperature of 200°C held for 10 min). FAME not available as authentic standards were identified in omasal digesta by GC-MS analysis of FAME and 4,4-dimethyloxazoline derivatives prepared from total FAME according to earlier reports( Reference Halmemies-Beauchet-Filleau, Kairenius and Ahvenjärvi 24 , Reference Shingfield, Kairenius and Ärölä 25 ).
Fatty alcohol chains linked to plasmalogens by an alk-1-enyl (vinyl) ether bond in omasal digesta were analysed as dimethyl acetals (DMA) under the same conditions as FAME. DMA were identified based on GC-MS analysis and comparison with previous reports in the literature( Reference Alves, Santos-Silva and Cabrita 26 ). The distribution of CLA isomers in omasal digesta was determined by HPLC using four silver-impregnated silica columns (ChromSpher 5 Lipids, 250×4·6 mm i.d., 5 μm particle size; Agilent Technologies) coupled in series and 0·1 % (v/v) acetonitrile in heptane as the mobile phase( Reference Halmemies-Beauchet-Filleau, Kairenius and Ahvenjärvi 24 ). Under these conditions, cis-10, trans-12-CLA and trans-10, cis-12-CLA elute as a single peak. Both isomers were resolved by repeating the HPLC analysis using a 2 % (v/v) acetic acid in heptane mobile phase( Reference Sæbø, Sæbø and Griinari 15 ).
Statistical analysis
The retrospective power analyses were implemented with GLIMMIX procedure of SAS (version 9.3; SAS Institute) according to an approach presented by Stroup( Reference Stroup 27 ). The power analysis was performed for milk fat content as the response variable. The targeted treatment means were considered to be 26 g/kg for HSO and 40 g/kg for the other three treatments. The variance estimates used in the power analysis were obtained from the actual data, as the analysis was implemented retrospectively. The variance estimates were 0·32 for the random cow effect and 19·8 for the residual. This yielded the powers of 75 % for interaction between forage ratio and oil supplementation and 96 % for both HSO v. LSO and HSO v. H which show that the experimental design was suitable for the designed purpose.
DM intake and the yield of milk and milk constituents recorded during days 22–25 of each experimental period were averaged before statistical analysis. Measurements of intake, milk production and flow of nutrients at the omasum were analysed by ANOVA for a 4×4 Latin square design with a 2×2 factorial arrangement of treatments, with a statistical model that included the fixed effects of period, concentrate level, SO supplementation and their interaction, and the random effect of cow using the Mixed procedure of SAS. Least-square means with their standard errors are reported and treatment effects were considered significant at P<0·05 and considered a trend at P=0·05–0·10.
Results
Chemical composition of experimental diets
Silage was of high quality, both in terms of nutritive value (online Supplementary Table S1) and fermentation characteristics (Table 1). Lipid in grass silage contained relatively high proportions of 16 : 0, 18 : 2n-6 and 18 : 3n-3, whereas cis-9-18 : 1 and 18 : 2n-6 predominated in concentrates and SO (online Supplementary Table S1).
Nutrient intake and milk production
By design, silage DM intake was lower (P<0·001) on H than L treatments, whereas high-concentrate diets increased (P<0·01) total DM intake (Table 2). SO had no effect (P>0·05) on silage DM intake, but tended to lower (P=0·09) total DM intake. Intakes of organic matter (OM), CP, water-soluble carbohydrate and gross energy were higher (P<0·01), and that of neutral detergent fibre (NDF) and potentially digestible NDF (pdNDF) were lower (P=0·001) for H than L treatments. SO tended (P<0·10) to lower the intake of OM, CP, water-soluble carbohydrate and fibre, whereas the decrease in starch intake to SO was greater (P<0·05 for FC×SO interaction) when included in the L than H diet (Table 2).
Table 2 Effect of dietary forage:concentrate (FC) ratio and sunflower oil (SO) on nutrient intake in lactating cowsFootnote * (Least-square mean values with their pooled standard errors; n 16)
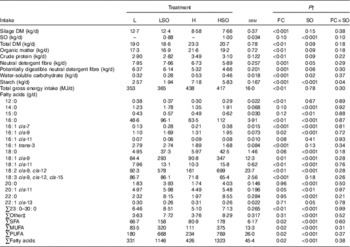
L, low-concentrate diet (FC ratio 65:35 on a DM basis) with no additional lipid; LSO, low-concentrate diet (FC ratio 65:35) containing 50 g SO/kg diet DM; H, high-concentrate diet (FC ratio 35:65) with no additional lipid; HSO, high-concentrate diet (FC ratio 35:65) containing 50 g SO/kg diet DM.
* Values represent the mean over days 22–25 of each experimental period.
† Significance of effects due to dietary FC ratio, supplements of SO and their interaction (FC×SO).
‡ Refers to the sum of 17 : 0, iso-18 :0, cis-9-17 : 1, cis-9, cis-12-16 : 2, trans-9-18 : 1, trans-11-18 : 1, trans-16/cis-14-18 : 1, cis-9, trans-12-18 : 2, trans-9, cis-12-18 : 2, trans-9, trans-12-18 : 2, cis-6, cis-9, cis-12-18 : 3, cis-6, cis-9, cis-12, cis-15-18 : 4, cis-11, cis-14-20 : 2, 21 : 0, cis-15-24 : 1 and unidentified fatty acids.
Both dietary FC ratio (P<0·05) and SO (P<0·001) altered total FA intake (Table 2). Intakes of SFA, MUFA and PUFA were greater on the high- than low-concentrate diets (P<0·05) and increased by SO (P<0·001). SO increased (P<0·01) the intake of 14 : 0, 15 : 0, 16 : 0, cis-6-16 : 1, cis-9-16 : 1, 18 : 0, cis-9-18 : 1, cis-11-18 : 1, 18 : 2n-6, 20 : 0, cis-11-20 : 1, 22 : 0 and 23 : 0 to 30 : 0, but lowered (P<0·05) that of cis-13-22 : 1. Compared with L treatments, intake of 16 : 0, cis-7-16 : 1, cis-9-16 : 1, cis-9-18 : 1, cis-11-18 : 1 and 18 : 2n-6 was higher (P<0·05) and 12 : 0, trans-3-16 : 1, 18 : 3n-3 and 23 : 0 to 30 : 0 ingestion was lower (P<0·01) on the H treatments (Table 2).
Treatments had no effect (P>0·05) on the yields of milk, energy corrected milk or lactose (Table 3). However, milk protein output was higher (P<0·01) on H than L diets. SO decreased milk fat content and secretion when included in the H (mean responses –1·28 % and −392 g/d, respectively; P<0·01 for FC×SO interaction), but not L diet (mean responses +0·25 % and +26 g/d, respectively; P<0·01 for FC×SO interaction).
Table 3 Effect of dietary forage:concentrate (FC) ratio and sunflower oil (SO) on milk production in lactating cowsFootnote * (Least-square mean values with their pooled standard errors; n 16)

L, low-concentrate diet (FC ratio 65:35 on a DM basis) with no additional lipid; LSO, low-concentrate diet (FC ratio 65:35) containing 50 g SO/kg diet DM; H, high-concentrate diet (FC ratio 35:65) with no additional lipid; HSO, high-concentrate diet (FC ratio 35:65) containing 50 g SO/kg diet DM.
* Values represent the mean over days 22–25 of each experimental period.
† Significance of effects due to dietary FC ratio, supplements of SO and their interaction (FC×SO).
‡ Energy-corrected milk calculated as milk (kg/d)×(3·83×fat (%)+2·42×protein (%)+1·654×lactose (%)+2·07)/3140( Reference Sjaunja, Baevre and Junkkarinen 55 ).
Nutrient flow at the omasum
Flow of OM, pdNDF and starch at the omasum (P<0·05) was higher and the amount of volatile fatty acids (VFA) and iNDF was lower (P<0·05) on H than L treatments (Table 4). SO decreased (P<0·05) VFA, total N and non-ammonia N at the omasum, the magnitude of which tended (P<0·10 for FC×SO interaction) to be greater for the H than L diet.
Table 4 Effect of dietary forage:concentrate (FC) ratio and sunflower oil (SO) on nutrient flow at the omasum in lactating cowsFootnote * (Least-square mean values with their pooled standard errors; n 16)
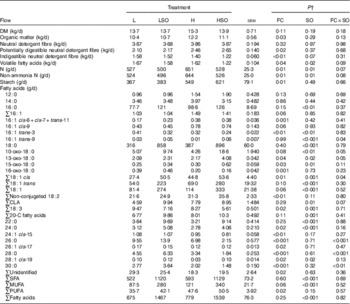
L, low-concentrate diet (FC ratio 65:35 on a DM basis) with no additional lipid; LSO, low-concentrate diet (FC ratio 65:35) containing 50 g SO/kg diet DM; H, high-concentrate diet (FC ratio 35:65) with no additional lipid; HSO, high-concentrate diet (FC ratio 35:65) containing 50 g SO/kg diet DM.
* Values represent the mean over days 22–24 of each omasal digesta sampling.
† Significance of effects due to dietary FC ratio, supplements of SO and their interaction (FC×SO).
For both L and H diets, SO increased (P<0·001) total FA flow at the omasum. However, both dietary FC ratio and SO altered the abundance and relative proportions of specific FA escaping the rumen. Changes to higher amounts of concentrates in the diet were characterised by increases (P<0·05) in unresolved cis-6, cis-7, and trans-11-16 : 1, 15-oxo-18 : 0, non-conjugated 18 : 2 and PUFA, and decreases (P<0·05) in trans-3-16 : 1, 16-oxo-18 : 0, total 18 : 3, 24 : 0, cis-15-24 : 1, cis-17-26 : 1 and cis-19-28 : 1 at the omasum (Table 4).
SO increased (P<0·05) the flow of 16 : 0, 18 : 0, 15-oxo-18 : 0, trans-18 : 1, total 18 : 1, CLA, total 20-C FA, 22 : 0, 24 : 0, cis-19-28 : 1, SFA and MUFA, and decreased (P<0·01) trans-3-16 : 1 and total 18 : 3 at the omasum (Table 4). However, supplements of SO resulted in greater increases in trans-9-16 : 1, 10-oxo-18 : 0 and 13-oxo-18 : 0 at the omasum when included in the H than L diet, whereas the reverse was true for total cis-18 : 1 (P<0·05 for FC×SO interaction). Furthermore, SO increased 26 : 0, 28 : 0 and 30 : 0 when included in the L diet, but decreased the amounts of these FA at the omasum following inclusion in the H diet (P<0·01 for FC×SO interaction).
SO resulted in the accumulation of trans-18 : 1 isomers in the rumen, with increases in specific isomers being dependent on the dietary FC ratio (Table 5). On the H diet, SO resulted in a greater increase (P<0·05 for FC×SO interaction) in trans-4 to trans-8-18 : 1 and trans-10-18 : 1 at the omasum compared with the L diet. In contrast, SO resulted in higher amounts of trans-9 and trans-11-18 : 1 at the omasum when included in the L than H diet (P<0·05 for FC×SO interaction). Irrespective of FC ratio, SO increased (P<0·001) trans-12 to trans-16-18 : 1 at the omasum. Trans-10-18 : 1 was the major 18 : 1 intermediate on the HSO treatment, whereas trans-11-18 : 1 was the most abundant on the LSO diet. Furthermore, SO increased (P<0·05) cis-13, cis-15 and cis-16-18 : 1, and resulted in greater increases of cis-9 and cis-12-18 : 1 at the omasum when included in the L than H diet (P<0·05 for FC×SO interaction). Amounts of cis-11, cis-13 and cis-16-18 : 1 and trans-5, trans-12 to trans-14-18 : 1 at the omasum were higher (P≤0·05) on the H than L diet.
Table 5 Effect of dietary forage:concentrate (FC) ratio and sunflower oil (SO) on the flow of 18-carbon unsaturated fatty acids at the omasum in lactating cowsFootnote * (Least-square mean values with their pooled standard errors; n 16)
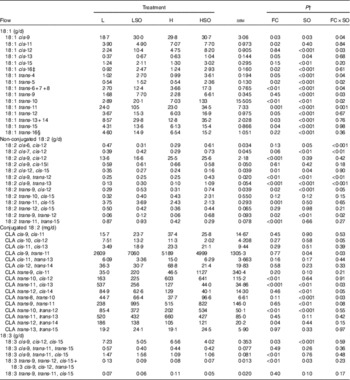
L, low-concentrate diet (FC ratio 65:35 on a DM basis) with no additional lipid; LSO, low-concentrate diet (FC ratio 65:35) containing 50 g SO/kg diet DM; H, high-concentrate diet (FC ratio 35:65) with no additional lipid; HSO, high-concentrate diet (FC ratio 35:65) containing 50 g SO/kg diet DM.
* Values represent the mean over days 22–24 of each omasal digesta sampling.
† Significance of effects due to dietary FC ratio, supplements of SO and their interaction (FC×SO).
‡ Coelutes with cis-8, cis-12-18 : 2 as a minor component.
§ Coelutes with cis-14-18 : 1.
|| Coelutes with cis-9-19 : 1.
SO resulted in greater increases in several 18 : 2 isomers, including cis-6, cis-12-18 : 2, cis-7, cis-12-18 : 2, cis-9, trans-12-18 : 2, cis-9, trans-13-18 : 2, trans-9, cis-12-18 : 2 and trans-9, trans-12-18 : 2 when included in the H than L diet (P<0·05 for FC×SO interaction; Table 5). Irrespective of dietary FC ratio, SO decreased (P<0·05) cis-12, cis-15-18 : 2 at the omasum. Increases in the proportion of dietary concentrates decreased (P≤0·001) trans-11, cis-15-18 : 2 and trans-11, trans-15-18 : 2 and increased (P<0·001) cis-9, cis-12-18 : 2 at the omasum.
Flows of trans-10, cis-12-CLA and trans-10, trans-12-CLA were higher (P<0·01) and that of trans-12, trans-14-CLA was lower (P<0·05) on H compared with L treatments (Table 5). SO increased (P<0·05) trans-9, trans-11-CLA and trans-10, trans-12-CLA at the omasum. When included in the L diet, SO increased the flow of cis-10, cis-12-CLA and cis-9, trans-11-CLA, but decreased the amount of both isomers at the omasum when included in the H diet (P<0·05 for FC×SO interaction). Furthermore, SO resulted in larger increases in trans-8, trans-10-CLA flow when included in the H than L diet (P<0·05 for FC×SO interaction). In contrast, decreases in trans-11, cis-13-CLA to SO were greater when included in the L than H diet (P<0·05 for FC×SO interaction), whereas the reverse was true for trans-12, cis-14-CLA (P=0·05 for FC×SO interaction). No cis-10, trans-12-CLA was detected in the omasal digesta.
SO lowered (P<0·05) cis-9, cis-12, cis-15-18 : 3 and unresolved trans-9, trans-12, cis-15-18 : 3 and cis-9, cis-12, trans-15-18 : 3 at the omasum (Table 5). Flows of cis-9, cis-12, cis-15-18 : 3, unresolved trans-9, trans-12, cis-15-18 : 3 and cis-9, cis-12, trans-15-18 : 3 and cis-9, trans-11, cis-15-18 : 3 were higher (P<0·05) on L than H treatments. SO increased (P<0·05) 20 : 0, cis-9+trans-14-20 : 1, cis-11-20 : 1 and trans-13-20 : 1 and 22 : 0 at the omasum (online Supplementary Table S2), whereas increases in dietary concentrates resulted in higher (P≤0·05) amounts of cis-9, cis-11 and trans-11+cis-15-20 : 1, trans-13-20 : 1, 20 : 2n-6 and cis-13-22 : 1 at the omasum. Including SO in the H diet resulted in a greater increase of trans-12-20 : 1 at the omasum compared with the L diet (P=0·05 for FC×SO interaction).
Dietary FC ratio and SO supplements altered the flow of odd- and branched-chain fatty acids (OBCFA) at the omasum (Table 6). Flows of 3-phenyl-3 : 0, anteiso-13 : 0, 15 : 0, iso-15 : 0, trans-5-15 : 1, trans-10-17 : 1, iso-18 : 0 and 25 : 0 were decreased (P≤0·05) and that of 11-cyclohexyl-11 : 0 was increased (P<0·01) on H compared with L treatments. SO increased (P<0·01) 19 : 0 and 23 : 0, but lowered (P<0·05) iso-15 : 0, trans-5-15 : 1 and iso-18 : 0 at the omasum. Flows of S3,R7,R11,15-tetramethyl-16 : 0, cis-14-23 : 1, 27 : 0 and 29 : 0 were increased by SO supplements in the L diet, but decreased when included in the H diet (P<0·05 for FC×SO interaction). Furthermore, decreases in the amounts of R3,R7,R11,15-tetramethyl-16 : 0 at the omasum were greater when SO was included in the L than H diet, whereas the reverse was true for anteiso-15 : 0 (P<0·05 for FC×SO interaction).
Table 6 Effect of dietary forage:concentrate (FC) ratio and sunflower oil (SO) on the flow of odd- and branched-chain and dicarboxylic fatty acids at the omasum in lactating cowsFootnote * (Least-square mean values with their pooled standard errors; n 16)
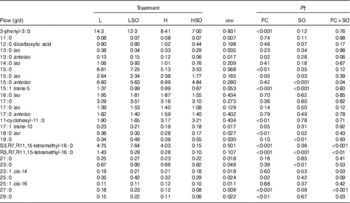
L, low-concentrate diet (FC ratio 65:35 on a DM basis) with no additional lipid; LSO, low-concentrate diet (FC ratio 65:35) containing 50 g SO/kg diet DM; H, high-concentrate diet (FC ratio 35:65) with no additional lipid; HSO, high-concentrate diet (FC ratio 35:65) containing 50 g SO/kg diet DM.
* Values represent the mean over days 22–24 of each omasal digesta sampling.
† Significance of effects due to dietary FC ratio, supplements of SO and their interaction (FC×SO).
SO lowered (P<0·01) total DMA flow at the omasum, decreases that tended to be greater when included in the H than L diet (P=0·06 for FC×SO interaction; Table 7). Furthermore, SO decreased (P<0·05) DMA of 15 : 0, 16 : 0, 17 : 0, iso-17 : 0 and 18 : 0 at the omasum. Increases in dietary concentrates lowered (P<0·05) DMA of 13 : 0, iso-14 : 0, 15 : 0, anteiso-15 : 0, iso-15 : 0, 26 : 0, 28 : 0 and 30 : 0, and increased (P<0·05) DMA of 16 : 0, 17 : 0, iso-17 : 0, trans-10-18 : 1 and cis-9-18 : 1. Inclusion of SO resulted in larger decreases in DMA of trans-11-18 : 1, cis-11-18 : 1 and cis-12-18 : 1 at the omasum when included in the H than L diet (P<0·05 for FC×SO interaction), whereas the decrease in anteiso-13 : 0 DMA to SO was higher when included in the L than H diet (P<0·05 for FC×SO interaction).
Table 7 Effect of dietary forage:concentrate (FC) ratio and sunflower oil (SO) on the flow of aldehydes at the omasum in lactating cowsFootnote * (Least-square mean values with their pooled standard errors; n 16)
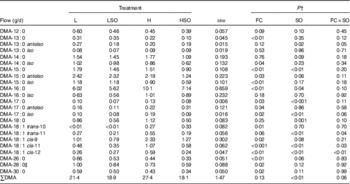
L, low-concentrate diet (FC ratio 65:35 on a DM basis) with no additional lipid; LSO, low-concentrate diet (FC ratio 65:35) containing 50 g SO/kg diet DM; H, high-concentrate diet (FC ratio 35:65) with no additional lipid; HSO, high-concentrate diet (FC ratio 35:65) containing 50 g SO/kg diet DM; DMA, dimethyl acetal.
* Values represent the mean over days 22–24 of each omasal digesta sampling.
† Significance of effects due to dietary FC ratio, supplements of SO and their interaction (FC×SO).
‡ Coelutes with 9-oxo-18 : 0.
Ruminal biohydrogenation
Dietary FC ratio had no effect (P>0·05) on apparent biohydrogenation of 18-C unsaturated FA whilst SO increased (P<0·001) apparent biohydrogenation of cis-9-18 : 1, 18 : 2n-6 and 18 : 3n-3 in the rumen (Table 8). No 18 : 4n-3 was detected in omasal digesta.
Table 8 Effect of dietary forage:concentrate (FC) ratio and sunflower oil (SO) on apparent biohydrogenation of 18-carbon unsaturated fatty acids in the rumen of lactating cowsFootnote * (Least-square mean values with their pooled standard errors; n 16)

L, low-concentrate diet (FC ratio 65:35 on a DM basis) with no additional lipid; LSO, low-concentrate diet (FC ratio 65:35) containing 50 g SO/kg diet DM; H, high-concentrate diet (FC ratio 35:65) with no additional lipid; HSO, high-concentrate diet (FC ratio 35:65) containing 50 g SO/kg diet DM.
* Values represent the mean over days 22–24 of each omasal digesta sampling.
† Significance of effects due to dietary FC ratio, supplements of SO and their interaction (FC×SO).
Discussion
High-concentrate diets containing high amounts of PUFA typically cause MFD in lactating cows( Reference Bauman and Griinari 9 – Reference Harvatine, Boisclair and Bauman 11 ). Changes in milk fat composition and secretion during diet-induced MFD on low fibre high-oil diets have been characterised( Reference Griinari, Dwyer and McGuire 4 – Reference Rico and Harvatine 7 ) but quantitative measurements of ruminal lipid metabolism and flow of FA escaping the rumen are limited( Reference Loor, Ueda and Ferlay 17 , Reference Shingfield, Kairenius and Ärölä 25 , Reference Piperova, Sampugna and Teter 28 ). Measurements of FA and DMA at the omasum were performed in cows fed diets formulated to cause changes in ruminal lipid metabolism and milk fat synthesis allowing alterations in ruminal biohydrogenation and formation of intermediates associated with a diet causing MFD to be characterised in detail.
By design, inclusion of SO in the high-concentrate diet decreased milk fat content and secretion relative to other treatments (mean decreases of −25·4 to −30·3 and −20·2 to −31·9 %, respectively). The magnitude of decreases in milk fat synthesis on the HSO diet is within the expected range reported for cows fed low fibre high-oil diets( Reference Bauman and Griinari 9 , Reference Shingfield and Griinari 10 ) or receiving post-ruminal infusions of FA preparations containing trans-10, cis-12-CLA( Reference Harvatine, Perfield and Bauman 29 ) and therefore representative of the MFD phenotype. In more extreme cases, decreases in milk fat on diets causing MFD can approach a physiological maximum of −50 %, but this is often accompanied by lowered intake( Reference Roy, Ferlay and Shingfield 6 , Reference Rico and Harvatine 7 , Reference Shingfield, Reynolds and Hervás 30 ) complicating the establishment of cause and effect.
Flow of total FA at the omasum was higher than intake on all treatments (344, 321, 353 and 216 g/d for L, LSO, H and HSO, respectively). The higher FA flow can be explained by the contribution of microbial lipid to total FA escaping the rumen. On most diets, dietary intake accounts for between 75 and 80 % of FA reaching the duodenum( Reference Doreau and Ferlay 31 , Reference Schmidely, Glasser and Doreau 32 ). Dietary lipid supplements containing PUFA have been shown to lower the net balance of FA in the rumen of lactating and growing cattle( Reference Doreau and Ferlay 31 , Reference Schmidely, Glasser and Doreau 32 ), but there were no indications in the present study that SO, even when offered in relatively high amounts, decreased FA flow at the omasum relative to intake.
On all diets, the intake of cis-9-18 : 1, cis-11-18 : 1, 18 : 2n-6 and 18 : 3n-3 exceeded flow at the omasum. Dietary FC ratio did not alter the extent of FA biohydrogenation in the rumen, whereas SO increased apparent ruminal biohydrogenation of unsaturated FA. Increases in dietary concentrates have been reported to have no effect on the extent of cis-9-18 : 1 metabolism, but decrease ruminal biohydrogenation of 18 : 2n-6 and 18 : 3n-3 in lactating cows( Reference Loor, Ueda and Ferlay 17 , Reference Kalscheur, Teter and Piperova 33 , Reference Dewhurst, Moorby and Vlaeminck 34 ). More extensive biohydrogenation of unsaturated FA on diets containing SO was associated with higher dietary FA intake consistent with the findings of a recent meta-analysis examining the relationship between the intake and flow of FA at the duodenum in ruminants( Reference Glasser, Schmidely and Sauvant 35 ). These observations provide further support that the main role of biohydrogenation in the rumen is to decrease the abundance of unsaturated FA in the rumen to minimise the inhibitory effects on microbial growth.
Although SO resulted in a higher 18 : 2n-6 intake, the amount reaching the omasum was not increased. Lipolysis is considered rate limiting for the conversion of unsaturated FA to saturated end products in the rumen( Reference Jenkins, Wallace and Moate 36 ). During incubations with rumen contents, lipolysis of SO is decreased when added at high concentrations( Reference Beam, Jenkins and Moate 37 ) or increased when fibre replaces starch( Reference Gerson, John and King 38 ). Lipolysis was not determined, but substantial metabolism of 18 : 2n-6 between the mouth and omasum on LSO and HSO treatments do not support hydrolysis of ester bonds of TAG in SO limiting biohydrogenation of unsaturated FA or that diet composition has a substantial influence on ruminal lipolysis of SO in vivo. Flow of 18 : 2n-6 at the omasum was almost 2-fold greater on H than L diets that is in agreement with earlier reports of higher ruminal escape of 18 : 2n-6 in lactating cows fed high-concentrate diets( Reference Loor, Ueda and Ferlay 17 , Reference Kalscheur, Teter and Piperova 33 , Reference Dewhurst, Moorby and Vlaeminck 34 ). It has been suggested that non-esterified 18 : 2n-6 could be selectively incorporated into the vacuoles of rumen bacteria( Reference Bauchart, Legay-Carmier and Doreau 39 ), minimising exposure to bacterial isomerases and reductases in the rumen. However, direct measurement of flows of 18 : 2n-6 at the omasum relative to intake do not suggest this is quantitatively important.
On all treatments, the reduction of dietary unsaturated FA in the rumen was incomplete and numerous 16 : 1, 18 : 1, 18 : 2 and 20 : 1 intermediates not supplied from the diet were detected in omasal digesta. Even though the extent of unsaturated FA biohydrogenation in the rumen did not differ between oil containing diets inclusion of SO in the high-concentrate diet altered ruminal biohydrogenation pathways.
During incubations with strained rumen contents 18 : 2n-6 is rapidly isomerised to yield geometric isomers of Δ9,11 and Δ10,12-CLA( Reference Wallace, McKain and Shingfield 12 , Reference Jouany, Lassalas and Doreau 40 , Reference Honkanen, Griinari and Vanhatalo 41 ). The amount of trans-10, cis-12-CLA at the omasum was higher on H than L diets but not altered in response to SO. Earlier experiments have shown that ruminal escape of trans-10, cis-12-CLA is promoted on low-fibre diets( Reference Loor, Ueda and Ferlay 17 , Reference Doreau and Ferlay 31 ) and in response to increased 18 : 2n-6 intake( Reference Shingfield, Ahvenjärvi and Toivonen 42 , Reference Sackmann, Duckett and Gillis 43 ). Under the specified conditions of this experiment, MFD on the HSO treatment was not accompanied by an increase in trans-10, cis-12-CLA that is known to inhibit milk fat synthesis in lactating cows( Reference Bauman and Griinari 9 , Reference Baumgard, Corl and Dwyer 13 , Reference Glasser, Ferlay and Doreau 14 ).
Incubations with mixed rumen contents or pure strains of rumen bacteria( Reference Wallace, McKain and Shingfield 12 , Reference Jouany, Lassalas and Doreau 40 , Reference Honkanen, Griinari and Vanhatalo 41 ) have shown that trans-10, trans-12-CLA is formed from 18 : 2n-6. Flows of trans-10, trans-12-CLA at the omasum were increased by SO and also higher on H than L diets suggesting that substrate supply and rumen environment may influence the specificity of the Δ-12 cis, Δ-11 trans isomerase or the rate at which 10,12-CLA products are reduced. However, flow of trans-10, trans-12-CLA at the omasum was not increased on the HSO treatment causing MFD. Post-ruminal infusion of geometric 10,12 CLA isomers has implicated cis-10, trans-12-CLA as a possible milk fat inhibitor( Reference Sæbø, Sæbø and Griinari 15 ), but no cis-10, trans-12-CLA was detected in omasal digesta consistent with earlier reports( Reference Stroup 27 , Reference Piperova, Sampugna and Teter 28 , Reference Shingfield, Ahvenjärvi and Toivonen 42 ).
Flow of cis-9, trans-11-CLA was increased during SO supplementation of the L but not H diet consistent with reports in growing cattle that sources of 18 : 2n-6 promote cis-9, trans-11-CLA at the duodenum on high-forage diets( Reference Sackmann, Duckett and Gillis 43 ). In contrast, the flow of cis-9, trans-11-CLA at the duodenum was found to be higher in cows following the inclusion of linseed oil into a high- rather than low-concentrate diet( Reference Loor, Ueda and Ferlay 17 ). Such findings indicate that substrate supply is not the sole factor regulating the synthesis of cis-9, trans-11-CLA in the rumen. Even though cis-9, trans-11-CLA is the main product formed during the isomerisation of 18 : 2n-6 in the rumen( Reference Harfoot and Hazlewood 44 ), other geometric 9,11 CLA isomers are also formed( Reference Wallace, McKain and Shingfield 12 , Reference Jouany, Lassalas and Doreau 40 , Reference Honkanen, Griinari and Vanhatalo 41 ). On both L and H diets, SO increased trans-9, cis-11-CLA at the omasum, with the amounts on the high-concentrate diet being numerically several-fold higher, consistent with the observed increases in milk trans-9, cis-11-CLA concentrations on low fibre high-oil diets causing MFD( Reference Roy, Ferlay and Shingfield 6 , Reference Perfield, Lock and Griinari 16 ). Abomasal infusion of a mixture of FA has identified trans-9, cis-11-CLA as a possible inhibitor of milk fat synthesis in the lactating cow( Reference Perfield, Lock and Griinari 16 ).
Irrespective of dietary lipid content, trans-11-18 : 1 was the major biohydrogenation intermediate on the L treatments. However, including SO in the H diet caused a shift in biohydrogenation pathways resulting in the formation of trans-10 containing products, with the most obvious being trans-10-18 : 1 replacing trans-11-18 : 1 as the major biohydrogenation intermediate formed in the rumen. Alterations in ruminal biohydrogenation favouring the synthesis of trans-10-18 : 1 are known to occur in lactating cows fed high-concentrate diets( Reference Piperova, Sampugna and Teter 28 ), high-starch low-fibre diets containing plant oils( Reference Loor, Ueda and Ferlay 17 ) or diets supplemented with high amounts of PUFA( Reference Shingfield, Kairenius and Ärölä 25 ). However, the amount of trans-10-18 : 1 formed on the HSO treatment (133 g/d) is much higher compared with earlier reports in lactating cows fed diets causing MFD( Reference Shingfield and Griinari 10 , Reference Shingfield, Kairenius and Ärölä 25 , Reference Piperova, Sampugna and Teter 28 ). Changes in diet composition promoting the formation of trans-10-18 : 1 are well characterised( Reference Bauman and Griinari 9 , Reference Shingfield and Griinari 10 ), but the underlying causes are not known. Examination of the effects of pH or dietary FC ratio on the production of biohydrogenation intermediates in dual-flow continuous culture suggested that decreases in pH rather than increases in the proportion of concentrates is the main factor responsible for the shift from trans-11-18 : 1 to trans-10-18 : 1( Reference Fuentes, Calsamiglia and Cardozo 45 ). In contrast, measurements of ruminal FA composition in cows fed diets containing low or high amounts of starch( Reference Zened, Enjalbert and Nicot 46 ) or FA flow at the omasum in cows fed incremental amounts of fish oil( Reference Shingfield, Kairenius and Ärölä 25 ) indicate that trans-10-18 : 1 formation in vivo to be independent of decreases in rumen pH. In the present experiment, greater formation of trans-10-18 : 1 on the HSO treatment was not associated with a decrease in mean daily rumen pH (results not presented). Trans-10-18 : 1 is thought to originate from the reduction of trans-10, cis-12-CLA( Reference McKain, Shingfield and Wallace 47 ). Therefore, a close association between trans-10-18 : 1 and trans-10, cis-12-CLA at the omasum might be expected, but none was observed. Concentrations of trans-10-18 : 1 are known to increase in milk from cows fed diets causing MFD( Reference Bauman and Griinari 9 , Reference Shingfield and Griinari 10 ), but direct evidence that trans-10-18 : 1 inhibits milk fat synthesis remains equivocal. Abomasal infusion of trans-10-18 : 1 was reported to have no effect on milk fat secretion( Reference Lock, Tyburczy and Dwyer 48 ), but these findings have been challenged on the basis that the effective dose at the mammary gland was too low for possible inhibitory effects to be detected( Reference Kadegowda, Piperova and Erdman 49 ). A more recent experiment demonstrated that post-ruminal infusion of a mixture of 18 : 1 methyl esters containing trans-10-18 : 1 lowered milk fat yield in lactating cows( Reference Shingfield, Sæbø and Sæbø 50 ). Further investigations are required to confirm the role of trans-10-18 : 1 in the regulation of milk fat synthesis.
Ruminal biohydrogenation of 18 : 3n-3 involves an initial isomerisation of the cis-12 double bond to yield cis-9, trans-11, cis-15-18 : 3 that is reduced to trans-11, cis-15-18 : 2( Reference Jenkins, Wallace and Moate 36 ). A higher intake of 18 : 3n-3 can account for the increases in cis-9, trans-11, cis-15-18 : 3, trans-11, cis-15-18 : 2 and trans-11, trans-15-18 : 2 at the omasum on L than H treatments. Even though SO did not increase 18 : 3n-3 intake the amount of trans-10, cis-15-18 : 2 at the omasum was higher for HSO than LSO providing additional support for alternative pathways of 18 : 3n-3 metabolism in the rumen( Reference Alves and Bessa 51 ) that appear quantitatively more important in cows fed diets causing MFD.
Appearance of OBCFA at the omasum originate from bacterial membrane lipids synthesised in the rumen using branched-chain amino acids as a primer( Reference Vlaeminck, Fievez and Cabrita 52 ), with the relative abundances of OBCFA differing between bacterial species( Reference Fievez, Colman and Castro-Montoya 53 ). Increases in dietary concentrate decreased the flow of several (3-phenyl-3 : 0, anteiso-13 : 0, 15 : 0, iso-15 : 0, trans-5-15 : 1, trans-10-17 : 1, iso-18 : 0 and 25 : 0) OBCFA, consistent with earlier studies( Reference Loor, Ueda and Ferlay 17 ). Such changes may reflect both changes in the rumen microbial community structure and the availability of substrates for bacterial OBCFA synthesis de novo. Total OBCFA at the omasum was lowest on the HSO diet, that is in agreement with earlier reports that lipid supplements decrease the proportion of OBCFA in lipid at the duodenum in cattle( Reference Loor, Ueda and Ferlay 17 , Reference Pantoja, Firkins and Eastridge 54 ), possibly due to inhibition of bacterial FA synthesis or by dilution with dietary lipid escaping the rumen. Membrane lipid of rumen bacteria is also characterised by the occurrence of DMA, but little is known on dietary factors influencing DMA synthesis in the rumen. SO tended to lower total DMA at the omasum, whereas the relative proportions of concentrate and forage in the diet had a larger influence, findings that are in agreement with recent reports on the effect of diet on DMA in rumen contents of sheep( Reference Alves, Santos-Silva and Cabrita 26 ).
Conclusions
Diets containing different proportions of concentrate and SO supplements resulted in varying effects on ruminal lipid metabolism and milk fat synthesis. Inclusion of SO in a high-concentrate diet caused MFD that was accompanied by alterations in ruminal biohydrogenation pathways promoting the formation of trans-10 containing intermediates. However, ruminal synthesis of trans-10, cis-12-CLA known to inhibit milk fat synthesis was not increased on a high-concentrate diet containing SO, suggesting that ruminal formation of other biohydrogenation intermediates or additional mechanisms also contribute to the MFD phenotype in lactating cows.
Acknowledgements
The authors gratefully acknowledge the contribution of staff at the Metabolism Unit of Natural Resources Institute Finland (Luke; Jokioinen, Finland) for the care of experimental animals and the collection of experimental samples under the supervision of Mari Talvisilta, and fatty acid analysis undertaken in the Luke laboratory by Minna Aalto and Tuija Hakala.
This work was supported by the Finnish Ministry of Agriculture and Forestry, Helsinki, Finland and Valio, Ltd, Helsinki, Finland. L. V. was a recipient of a PhD studentship from the Raisio Science Foundation, Raisio, Finland and the August Johannes and Aino Tiura Agricultural Science Foundation, Helsinki, Finland. None of the funding agencies had role in experimental design, data analysis or writing of this article.
The authors’ contributions are as follows: K. J. S. and J. V. designed the experiment; L. V., P. K., H. L. and T. S. performed the research; L. V., H. L., A. R. B. and K. J. S. analysed experimental data; K. J. S. and L. V. wrote the paper; and K. J. S. had primary responsibility for the final content. All authors have read and approved the final manuscript contents.
The authors have no financial or personal conflicts of interest to declare.
Supplementary material
For supplementary material/s referred to in this article, please visit https://doi.org/10.1017/S0007114517000010