Detailed understanding of how children's emotional and behavioral disturbances develop is critical for effective guidance of treatment and prevention efforts (Kring & Sloan, Reference Kring and Sloan2009; Sroufe, Reference Sroufe1997). To date, research has shown that the etiology of psychopathology is multifaceted and warrants consideration of factors at multiple levels and across developmental time (Cicchetti, Reference Cicchetti and Zelazo2013; Doom & Gunnar, Reference Doom and Gunnar2013). In particular, although experiences of stress and adversity in early life have been associated with increased risk of developing psychopathology later in life (Baldwin et al., Reference Baldwin, Caspi, Meehan, Ambler, Arseneault, Fisher and Poulton2021; Jones-Mason, Behrens, & Gribneau Bahm, Reference Jones-Mason, Behrens and Gribneau Bahm2019; McEwen & Akil, Reference McEwen and Akil2020; McLaughlin, Reference McLaughlin2016; Wesarg, Van Den Akker, Oei, Hoeve, & Wiers, Reference Wesarg, Van Den Akker, Oei, Hoeve and Wiers2020; Widom, Reference Widom2000), not all who encounter adversity experience significant disturbances or exhibit impairment, with risk for particular individuals being very difficult to predict, relative to risk at a population level (e.g., Baldwin et al., Reference Baldwin, Caspi, Meehan, Ambler, Arseneault, Fisher and Poulton2021). This is because the pathways toward maladaptation or resilience are complex and depend upon a variety of individual, family, and broader contextual factors, crossing levels and systems, throughout development (Bush & Boyce, Reference Bush and Boyce2016; Cicchetti & Rogosch, Reference Cicchetti and Rogosch1996; Masten et al., Reference Masten, Hubbard, Gest, Tellegen, Garmezy and Ramirez1999; Suor, Sturge-Apple, Davies, Cicchetti, & Manning, Reference Suor, Sturge-Apple, Davies, Cicchetti and Manning2015; Ungar & Theron, Reference Ungar and Theron2020). Moreover, the varied impact of adversity can manifest in a range of diverse health outcomes, making it difficult to track. Examinations that consider this multifinality (i.e., the relation of a transdiagnostic risk factor to multiple outcomes including typical functioning and/or psychopathologies; Cicchetti & Rogosch, Reference Cicchetti and Rogosch1996), and the early life phenotypes that lie on the pathways to disease, may help identify and support those at risk early in development. Therefore, the current study implemented a multilevel, multidomain approach to explore longitudinal associations between early life adverse experiences, the coordinated regulation of multiple physiological systems, and their linkage to variations in executive functioning – an important set of cognitive abilities that aid in normative developmental processes and have been associated with later risk for psychopathology (Bloemen et al., Reference Bloemen, Oldehinkel, Laceulle, Ormel, Rommelse and Hartman2018).
Frameworks for examining the complex etiology of developmental psychopathology
This special issue examines developmental psychopathology within the National Institute of Mental Health's Research Domain Criteria (RDoC) framework, which was developed to move away from a clinical, diagnosis-driven model of assessing and treating psychopathology. The RDoC initiative conceptualizes disorders using a dimensional framework of behavioral, neurodevelopmental, and biological measures (First, Reference First2012; Insel et al., Reference Insel, Cuthbert, Garvey, Heinssen, Pine, Quinn and Wang2010). This pathophysiology-based framework focuses on underlying mechanisms that contribute to the full range of variation in symptomatology from typical functioning to psychopathology, with a focus on the complex and multilevel influences (e.g., across genetic, physiological, and environmental domains) on transdiagnostic vulnerabilities to developing psychopathology (Cuthbert & Insel, Reference Cuthbert and Insel2013). The five primary research domains within this framework – negative valence, positive valence, cognitive systems, social processes, and regulatory systems –encompass the primary interacting constructs that can be measured across seven primary units of analysis: genetics, molecules, cells, circuits, physiology, behavior, and self-reports. Contemporary research has emphasized the utility of applying an RDoC framework to the study of risk and resilience processes. For example, a review of recent studies of child maltreatment identified variations in both structural and functional brain regions of children exposed to maltreatment, including key areas that are involved in the stress response (Kaufman, Gelernter, Hudziak, Tyrka, & Coplan, Reference Kaufman, Gelernter, Hudziak, Tyrka and Coplan2015). These alterations in areas such as the prefrontal cortex and amygdala are also associated with problems in executive functioning abilities such as attention and impulse control as well as specific features of psychiatric disorders, suggesting neurophysiological pathways to disorder development among children exposed to early adversity.
Over the past decade, RDoC has advanced the etiopathology of mental disorders, with particular emphasis on neuroplasticity and epigenetics. However, considerations of developmental timing and mechanisms within the RDoC framework are more obscure. Currently, RDoC uniformly applies “development” to all constructs and units of analysis within the RDoC matrix, but it remains ambiguous as to how researchers define and incorporate development into study designs (Casey, Oliveri, & Insel, Reference Casey, Oliveri and Insel2014; Cuthbert, Reference Cuthbert2014). Recently, Mittal and Wakschlag (Reference Mittal and Wakschlag2017) proposed a thoughtful conceptualization of development and environment to this pathophysiology-based framework of psychopathology. Specifically, they argue that harnessing a neurodevelopmental approach to understanding atypicality in the developmental patterning of behavior across domains can highlight early deviations associated with later onset of psychological disorders. Recent evaluations of the RDoC framework have called for such incorporations of developmental influence in expressions of normative behavior and expected neural–behavioral relations (Beauchaine & Hinshaw, Reference Beauchaine and Hinshaw2020).
A theoretical precursor to the RDoC framework, biological embedding, emphasized a multilevel and developmental approach to understanding the ways in which our social environment can “get under the skin” to influence the trajectories of children's development (Hertzman, Reference Hertzman1999; Hertzman & Boyce, Reference Hertzman and Boyce2010). During the first few years of life, a period of high developmental plasticity, biological systems may be especially sensitive to environmental inputs, presenting a unique opportunity for adversities to alter these developing biological processes (e.g., epigenetic, neural, physiological, and immunological) in persistent ways that influence health and development across the life course (Bush, Lane, & McLaughlin, Reference Bush, Lane and McLaughlin2016; Nelson, Reference Nelson2013; Shonkoff, Reference Shonkoff2012). Such experiences occur across a variety of contexts proximal to the child (e.g., witnessing domestic violence, adversity experienced by the child, poverty) and more distal environmental factors (e.g., neighborhood violent crime). Well aligned with the RDoC framework, studies of biological embedding suggest alterations associated with early adverse experiences emerge across varied biological structures and physiological systems (Bush et al., Reference Bush, Jones-Mason, Coccia, Caron, Alkon, Thomas and Adler2017; Evans & Kim, Reference Evans and Kim2007, Reference Evans and Kim2013; Kim et al., Reference Kim, Evans, Angstadt, Ho, Sripada, Swain and Phan2013; Luby et al., Reference Luby, Belden, Botteron, Marrus, Harms, Babb and Barch2013; McEwen, Reference McEwen2019; McEwen & Akil, Reference McEwen and Akil2020).
The role of physiological stress response systems in multilevel frameworks
Variations in physiological stress regulation may be particularly important for mapping transdiagnostic outcomes in contexts of early adversity because physiological responses underlie our everyday functioning across cognitive, behavioral, and emotional processes, including response to stressful or threatening events (Doom & Gunnar, Reference Doom and Gunnar2013; Gunnar, Reference Gunnar and Nelson2000; McEwen & Akil, Reference McEwen and Akil2020). Stress-sensitive physiological systems such as the autonomic nervous system (ANS) and hypothalamic–pituitary–adrenal (HPA) axis are central for navigating various challenges, both those that are normative and those associated with adverse environments. Theories of allostasis posit that, in response to environmental demands, physiological responses across multiple systems engage in an ongoing calibration in order to support homeostasis (McEwen & Wingfield, Reference McEwen and Wingfield2003). However, when children's environments consist of chronic or repeated threat to their well-being (e.g., parents with substance abuse, experiences of maltreatment or neglect, parental separation) these stress-responsive physiological systems can become strained, contributing to the overall “wear and tear” on the body (i.e., allostatic load). In the long term, this wear and tear on these systems reduces the capacity for adaptive physiological responses to future stressors (McEwen, Reference McEwen2008). Such physiological dysregulation may appear as reactivity and/or recovery processes that are inadequate to meet environmental demands or may meet immediate demands but increase potential risks for negative long-term physical or psychosocial health outcomes. In fact, alterations in both the ANS and HPA axis may be the primary pathways through which allostatic load develops (Eriksson, Räikkönen, & Eriksson, Reference Eriksson, Räikkönen and Eriksson2014; Lupien, Juster, Raymond, & Marin, Reference Lupien, Juster, Raymond and Marin2018).
Research exploring the impact of early life stress on physiological regulatory systems has found that exposure to early adversity is associated with differences in both ANS and HPA indicators (Bunea, Szentágotai-Tătar, & Miu, Reference Bunea, Szentágotai-Tătar and Miu2017; Bush et al., Reference Bush, Jones-Mason, Coccia, Caron, Alkon, Thomas and Adler2017; Jones-Mason et al., Reference Jones-Mason, Behrens and Gribneau Bahm2019; Raymond, Marin, Majeur, & Lupien, Reference Raymond, Marin, Majeur and Lupien2018; Simmons et al., Reference Simmons, Badcock, Whittle, Byrne, Mundy, Patton and Allen2016; Tarullo & Gunnar, Reference Tarullo and Gunnar2006). For example, a meta-analysis found moderate to large effects of early stress predicting blunted cortisol responses (Bunea et al., Reference Bunea, Szentágotai-Tătar and Miu2017). Such alterations in physiological function associated with early adversity may be a potential mechanism by which later psychopathology develops. However, most studies of biological embedding examine socioemotional or behavioral problems that serve as antecedents to psychological disorder diagnosis, while research exploring early developmental biobehavioral processes underlying these antecedents, such as executive functioning, that may confer transdiagnostic vulnerability, is less common.
Executive functioning as an early life predictor of risk for psychopathology
Executive functioning encompasses complex cognitive processes central to children's normative development including inhibitory control, working memory, and cognitive flexibility (Moriguchi, Chevalier, & Zelazo, Reference Moriguchi, Chevalier and Zelazo2016). Executive functioning abilities have well-documented associations with social interactions (Moriguchi, Reference Moriguchi2014), sensation seeking and risk taking behaviors (Harms, Zayas, Meltzoff, & Carlson, Reference Harms, Zayas, Meltzoff and Carlson2014; Nigg, Reference Nigg2017), academic achievement (Samuels, Tournaki, Blackman, & Zilinski, Reference Samuels, Tournaki, Blackman and Zilinski2016), high-school graduation rates (Castellanos-Ryan et al., Reference Castellanos-Ryan, Pingault, Parent, Vitaro, Tremblay and Seguin2017), and many more aspects of children's social, emotional, and cognitive processing that are integral for healthy functioning. Similarly, deficits or deviations from the normal progression in executive functioning across development have been linked to a number of psychological disorders (Bloemen et al., Reference Bloemen, Oldehinkel, Laceulle, Ormel, Rommelse and Hartman2018; Nigg, Reference Nigg2017; Rinsky & Hinshaw, Reference Rinsky and Hinshaw2011). For example, a recent investigation exploring whether executive functioning abilities can be used as a transdiagnostic indicator for later psychopathology found that deviations in executive functioning at age 11 predicted a latent general psychopathology factor (composed of patterns across internalizing, externalizing, attention deficit/hyperactivity, and autism spectrum indicators) across ages 11, 14, 16, and 19 (Bloemen et al., Reference Bloemen, Oldehinkel, Laceulle, Ormel, Rommelse and Hartman2018).
Early adverse experiences may disrupt executive functioning (DePrince, Weinzierl, & Combs, Reference DePrince, Weinzierl and Combs2009; Kirke-Smith, Henry, & Messer, Reference Kirke-Smith, Henry and Messer2014; Merz & McCall, Reference Merz and McCall2011; Moriguchi et al., Reference Moriguchi, Chevalier and Zelazo2016; Sheridan, Peverill, Finn, & McLaughlin, Reference Sheridan, Peverill, Finn and McLaughlin2017; Ursache, Noble, & Blair, Reference Ursache, Noble and Blair2015; Wade, Zeanah, Fox, & Nelson, Reference Wade, Zeanah, Fox and Nelson2019), though varied associations have been reported. For example, children's reports of neglectful caregiving were associated with lower parent reports of executive functioning in adolescence; however, experiences of abuse and community violence did not significantly predict adolescents’ executive functioning (Sheridan et al., Reference Sheridan, Peverill, Finn and McLaughlin2017). Yet, a well-powered study exploring the influence of both early life trauma exposure and trauma-related symptoms in preschoolers found no statistically significant associations of either on young children's executive function task performance (Cohodes, Chen, Lieberman, & Bush, Reference Cohodes, Chen, Lieberman and Bush2020). These mixed patterns of associations between early adverse environments and executive functioning point to the need for further investigation in this realm, although studies utilizing objective measures of executive functioning in young children are mostly likely to be most informative.
Associations among physiology, executive functioning, and early adversity
In particular, physiological regulation may be especially salient for understanding the mechanism by which deficits in executive functioning arise following early adverse exposures. Physiological regulation across multiple complex and interconnected systems undergird an individual's abilities to respond to threat or challenge as well as sustain a calm and attentive focus (McEwen, Reference McEwen2007; Porges, Reference Porges2009). In recent years, studies have begun to demonstrate the importance of physiological regulation across multiple systems for executive functioning (Berry, Blair, Ursache, Willoughby, & Granger, Reference Berry, Blair, Ursache, Willoughby and Granger2014; Lupien, Maheu, Tu, Fiocco, & Schramek, Reference Lupien, Maheu, Tu, Fiocco and Schramek2007; Obradović & Finch, Reference Obradović and Finch2016; Obradović, Portilla, & Ballard, Reference Obradović, Portilla and Ballard2016). For example, a laboratory assessment in 3.5-year-old children found that a moderate parasympathetic withdrawal during challenge best supported children's executive functioning abilities (Marcovitch et al., Reference Marcovitch, Leigh, Calkins, Leerks, O'Brien and Blankson2010). Similarly, a cross-sectional investigation found that executive function was strongly and positively correlated with resting parasympathetic, but not sympathetic activity (Williams et al., Reference Williams, Cribbet, Tinajero, Rau, Thayer and Suchy2019). Further, studies of cortisol show somewhat conflicting findings, with some finding higher cortisol reactivity is associated with decreased executive functioning (Wagner et al., Reference Wagner, Cepeda, Krieger, Maggi, D'Angiulli, Weinberg and Grunau2016), and others showing no association with cortisol reactivity (Guevara & Murdock, Reference Guevara and Murdock2019).
Exploring pathways from early social environments to physiological regulation to executive functioning, Blair et al. (Reference Blair, Granger, Willoughby, Mills-Koonce, Cox, Greenberg and Investigators2011) found that baseline cortisol partially mediated the effects of positive, but not negative, parenting on executive functioning in 2-year-old children. Specifically, greater positive parent parenting was associated with lower baseline cortisol in children, which in turn, was associated with higher executive functioning. Similarly, cortisol reactivity also served a mediator for the relation between early adversity and later executive functioning in a sample of 11-year-old children (Conradt et al., Reference Conradt, Abar, Lester, LaGasse, Shankaran, Bada and Hammond2014). Further, a longitudinal study of children's cortisol and executive functioning over time found little evidence for bidirectional effects between executive functioning and cortisol over time; however. both indicators acted as potential pathways from early risk (i.e., low income and cumulative risk) to later adjustment problems (Lengua et al., Reference Lengua, Thompson, Moran, Zalewski, Ruberry, Klein and Kiff2020). Although more studies are needed, early life executive functioning appears to be shaped by both positive and negative social environmental factors, potentially through stress–biology pathways.
Implementing a multisystem physiology approach
Importantly, physiological regulation occurs across multiple, collaborative systems and recent synthesis points to a need to explore how multiple biological and behavioral systems work in concert to shape the development of psychopathology before problems arise (Koss & Gunnar, Reference Koss and Gunnar2018). Multisystem examinations of physiology have theoretical roots in allostasis, which posit calibration across multiple biological systems to maintain and restore homeostasis (McEwen, Reference McEwen2012). As one of the most comprehensive theories of multisystem stress responding, the adaptive calibration model (ACM) proposes variability in both resting and reactivity levels of sympathetic nervous system (SNS), parasympathetic nervous system (PNS), and HPA axis that comprise four patterns: sensitive (high PNS rest and reactivity, moderate SNS rest and reactivity, and moderate HPA rest and high reactivity), buffered (moderate-to-high PNS rest and reactivity, low-to-moderate SNS rest and reactivity, moderate HPA rest and reactivity), vigilant (low PNS rest and low-to-moderate PNS reactivity, high SNS rest and reactivity, and moderate-to-high HPA rest and reactivity), and unemotional (low rest and reactivity across all systems). Prior empirical evidence has found evidence of profiles of ANS and HPA regulation similar to the vigilant and buffered responses in the ACM (Ellis, Oldehinkel, & Nederhof, Reference Ellis, Oldehinkel and Nederhof2017; Roubinov, Boyce, Lee, & Bush, Reference Roubinov, Boyce, Lee and Bush2020). However, little research has taken a multisystem perspective in exploring the associations between physiological regulation and variations in executive functioning. As a notable exception utilizing a different analytic approach, a recent study found that while neither indicator predicted executive functioning alone, baseline cortisol (and index of HPA activation) and respiratory sinus arrhythmia (RSA; an indication of PNS activation) interacted to predict executive functioning such that children who had combined responses of lower RSA and lower cortisol at rest or higher RSA and higher cortisol at rest had higher executive functioning scores compared to children with low resting RSA and high resting cortisol (Braren et al., Reference Braren, Brandes-Aitken, Perry, Williams, Lyons, Rowe-Harriott and Blair2020).
Prior studies of multisystem physiological regulation across the ANS and HPA axis have found distinct profiles of multisystem responding among older children and adolescents akin to those proposed in the ACM (Ellis et al., Reference Ellis, Oldehinkel and Nederhof2017; Roubinov et al., Reference Roubinov, Boyce, Lee and Bush2020). We have also found evidence of similar discrete profiles in the current sample (Rudd, Bush, Alkon, & Roubinov, Reference Rudd, Bush, Alkon and Roubinov2021). Specifically, in a sample of racially/ethnically diverse, low-income children at 18 and 36 months we identified profiles of generally moderate activity (moderate arousal) and heightened baseline activity (anticipatory arousal) at both timepoints as well as a profile of typically adaptive patterns across all systems (active copers) at 18 months only and a profile of heightened HPA axis activity (HPAaxis responders) at 36 months only. Membership in the multisystem physiological profile of anticipatory arousal over time was associated with higher children's internalizing problems in exploratory models; however, associations with executive functioning have yet to be examined.
Current study
The current study tests the pathways from early life adversity to later psychopathology with a multilevel, multidomain, prospective examination of the associations between early life adversity, multisystem physiological regulation, and later executive functioning. Utilizing a longitudinal, racially/ethnically and socioeconomically diverse sample of mother–child dyads, we examined children's exposures to adverse experiences from birth to 18 months old, physiological reactivity and regulation across the ANS and HPA axis at 36 months, and their task-based executive functioning at age 5. Building upon previously established latent profile analyses (LPA) in this sample (Rudd et al., Reference Rudd, Bush, Alkon and Roubinov2021), we derived profiles of heart rate (HR), respiratory sinus arrythmia (RSA), pre-ejection period (PEP), and cortisol during periods of rest and reactivity to characterize multisystem physiological regulation. After selecting the best fitting measurement model, we simultaneously assessed the direct and indirect associations from early adversity to multisystem physiological profiles to variations in executive functioning (see Figure 1).

Figure 1. Conceptual model of the full path analysis, with latent profile analysis to a distal outcome.
We expected that identified profiles of multisystem physiological regulation would replicate those from our previous investigation, yielding three primary profiles characterized as HPA axis responders, anticipatory arousal, and moderate arousal. We hypothesized that reports of more adverse events before 18 months of age would be related to lower executive functioning scores and that multisystem physiological regulation would be significantly associated with adverse experiences early in life as well as variations in executive functioning later in childhood. In line with the ACM and our previous investigations (Roubinov et al., Reference Roubinov, Boyce, Lee and Bush2020; Rudd et al., Reference Rudd, Bush, Alkon and Roubinov2021), we hypothesized that children who experienced more types of adverse events would be more likely to evidence a multisystem physiological response characterized by anticipatory arousal and that membership in this profile would be associated with the lowest executive functioning scores. Further, we hypothesized that profiles with regulatory responses thought to best support executive functioning (i.e., low arousal at rest, moderate reactivity; Marcovitch, et al., Reference Marcovitch, Leigh, Calkins, Leerks, O'Brien and Blankson2010) would be associated with the highest executive functioning scores.
Method
Participants
Participants included 113 mother–infant dyads who were part of a longitudinal study to explore the effects of environmental stress, maternal weight, and health on child health and development (see Bush et al., Reference Bush, Lane and McLaughlin2016 for details). Inclusion criteria for the women were: (a) English speaking, (b) between 18–45 years of age, (c) 8–23 weeks pregnant with singleton, (d) have a pre-pregnancy body mass index (BMI) of 25–40 kg/m2, and (e) incomes less than 500% of the Federal Poverty Level (i.e., $73,550 for a family of 2), which is considered low income given the cost of living in the geographic area from which participants were drawn (US Department of Health and Human Services, 2011). Women were excluded if they (a) had medical conditions that were known to interfere with baseline body composition or gestational weight gain, (b) were currently taking medications related to weight loss, diabetes, antidepressants, antipsychotics, opiate drugs, or corticosteroids, or (c) had received gastric bypass surgery. The Institutional Review Board approved all study protocols and written informed consent was collected from the women before initiation of any data collection with mother or child.
The current study sample included only dyads who participated in physiological data collection at the 36-month assessment (N = 113; M = 38.59 months, SD = 3.31) and had valid executive functioning data (N = 100). Outcome data on children's age-normed executive functioning were primarily collected from the age-5 assessment (Mage = 5.69 years, SD = 0.54). However, there were several instances (N = 10) where a child did not have valid executive functioning data at the age-5 study visit but did have complete data for the same task at the prior age-4 study visit (Mage = 4.57 years, SD = 0.33). Given the age-normed nature of these standardized scores, and to allow for the largest sample size and greatest sample representation, these children were also included, yielding the total sample of 100 children who completed the task-based executive functioning assessment and were between 4 and 5 years of age (Mage = 5.64 years, SD = 0.61). The sample included 52.2% female children and was racially and ethnically diverse, including 29.9% African American, 27.8% mixed race or other, 21.7% Latinx, 7.0% White, and 4.3% Asian. Approximately two-thirds (68%) of mothers were married or partnered; 54% were multiparous; and approximately 22% completed high school or less, 57% had some college or vocational training, and 21% earned a college degree. Although the sample was predominantly low income, family incomes ranged from $2,500 to $175,000 (Median = $20,500).
Procedures
At each timepoint, mothers were invited to complete in-person assessments at the university clinic or in their home. Mothers completed semi-structured questionnaires when the children were 18 months old, 36 months old, and 5 years old. At 36 months, child physiology was assessed during an age-appropriate developmental challenges protocol (DCP) which includes challenges across cognitive, sensory, and emotional domains (for protocol details, see Bush et al., Reference Bush, Lane and McLaughlin2016; Stephens et al., Reference Stephens, Bush, Weiss and Alkon2020). Each challenge condition was paired with a parallel nonstressful control condition. Research suggests that using task-specific controls to compute reactivity provides a more accurate reactivity score by controlling for cognitive and motor activation and isolating physiological reactivity to the psychological elements of the challenge (Bush, Alkon, Obradović, Stamperdahl, & Boyce, Reference Bush, Alkon, Obradović, Stamperdahl and Boyce2011).
Prior to the initiation of the DCP and placement of electrodes for ANS data collection, researchers collected the first saliva sample from the children. At the start of the protocol, resting ANS cardiac measures were collected during a 2-minute neutral, prerecorded audio story. Children then completed tasks in the following order: a repeat after me task (control condition for the cognitive task), picture naming task (cognitive challenge), water drink (control condition for sensory challenge), sour lemon juice taste (sensory challenge), watching a calm video clip (control condition for emotional challenge), and watching a scary video clip (emotional challenge). Following the completion of the protocol, electrodes were removed, and the final saliva sample was collected at approximately 30 min after the DCP in order to capture peak cortisol stress reactivity (Granger et al., Reference Granger, Kivlighan, El-Sheikh, Gordis, Stroud, J. L. and C. G.2008). At the age-5 assessment children were also asked to complete an executive functioning computer task. The task was adaptive, with the starting point depending on the child's age. Task completion ranged between 2–7 min.
Measures
Demographics
Gestational age and child sex were obtained via labor and delivery medical records and child age at assessment was calculated based on their birthday. At age 4, mothers reported on their highest level of education as well as total household income and household size, which were used to calculate a continuous score of income adjusted for household size (Sebelius, Reference Sebelius2011). Child age and sex were used as covariates in the measurement model when constructing latent profile membership, and maternal education and income were used as covariates in prediction of children's executive functioning.
Children's traumatic events
Mothers reported on their children's experiences of traumatic events at the 18-month visit using an adapted version of the Trauma Exposure Symptom Inventory – Parent Report Revised (TESI-PRR; Ippen et al., Reference Ippen, Ford, Racusin, Acker, Bosquet, Rogers and Edwards2002). The TESI-PRR is a 24-item measure of children's exposure to a range of traumatic events, including reports of separation from a primary caregiver, maltreatment, and accidental traumas such as being in a serious accident that resulted in injury. The current study used an abbreviated 13-item version of this scale that did not include any offenses that would necessitate reporting to child protective services, such as instances of sexual abuse or ongoing physical abuse. Responses were coded dichotomously as 0 (not exposed) or 1 (exposed) to that type of adversity, and a total adversity score was calculated by summing all items. The TESI has been validated against existing measures of children's adversity exposure (Berent et al., Reference Berent, Crusto, Lotyczewski, Greenberg, Hightower and Kaufman2008).
Autonomic nervous system (ANS)
ANS regulation during the DCP was collected continuously from children when they were 36 months old using BioNex hardware and BioLab acquisition software version 3.0. The full ANS collection and scoring methods protocols have been previously described for this time point of collection (Stephens et al., Reference Stephens, Bush, Weiss and Alkon2020). After familiarizing children with the equipment, four disposable spot electrodes were placed on the children's neck and chest to collect impedance and respiratory measures and three spot electrodes were placed on the right clavicle, lower left rib, and right abdomen for electrocardiogram (ECG) measures (Bush et al., Reference Bush, Lane and McLaughlin2016). Data were monitored on the computer for signal and noise and data were stored offline for later analysis. Data were filtered, extracted, and then scored in 30-s intervals using Mindware software (HRV 3.1.0 F and IMP 3.1.0H, Mindware Technologies, Ltd, www.mindwaretech.com). Data cleaning procedures involved examining for artifacts, checking all outliers (>3SD), and deleting individual data files for children if more than 25% of the 30-s epochs were unscorable (N = 4).
Multisystem physiological profiles have been previously validated using latent profile analysis in the current sample (Rudd et al., Reference Rudd, Bush, Alkon and Roubinov2021). This prior study included physiological data collected from children at both 18 and 36 months in order to evaluate developmental change in multisystem functioning over time. Due to the young age of children, the 18-month assessment could not include task-specific controls. Thus, our prior study calculated 18-month reactivity scores using the general baseline task and to maintain equivalency in our physiological measures over time, we also calculated physiological reactivity measures at 36 months using the general baseline task (Rudd et al., Reference Rudd, Bush, Alkon and Roubinov2021). As previously noted (see p. 12), the 36-month assessment included task-specific controls. To leverage this additional information in the current study, we use task-specific controls to derive RSA and PEP reactivity at 36 months (as described below).
Resting RSA and RSA reactivity
RSA is the naturally occurring variation in HR that occurs as a function of respiration. RSA was estimated as the natural logarithm of the variance of heart period within the high-frequency bandwidth associated with respiration at this age, 0.15 to 1.04 Hz (Bar-Haim, Marshall, & Fox, Reference Bar-Haim, Marshall and Fox2000; Landrot et al., Reference Landrot, Roche, Pichot, Teyssier, Gaspoz, Barthelemy and Patural2007). Measures of resting RSA were derived by calculating the mean RSA magnitude during the 2-minute prechallenge resting period when children listened to an audio-recorded story. Mean magnitude of RSA was also separately calculated for each of the control tasks and their corresponding challenge tasks across the cognitive, sensory, and emotional domains. Subsequently, three task-specific RSA reactivity scores were derived by regressing RSA during each challenge task on RSA during the matched control task and saving the standardized residuals. Finally, the three standardized residual scores were averaged to create a single measure of RSA reactivity. Negative residual scores are indicative of greater RSA reactivity (a decrease in RSA; parasympathetic withdrawal) while positive residual scores are indicative of lower RSA reactivity (an increase in RSA; parasympathetic activation).
Resting PEP and PEP reactivity
PEP is a systolic time interval representing the elapsed duration from the beginning of electrical stimulation until the ejection of blood from the left ventricle. PEP data were extracted and scored using impedance technologies where the ECG and impedance waveforms were used to obtain PEP measures quantified as the time interval in milliseconds from the onset of the ECG Q-wave to the B point of the dZ/dt wave (Berntson et al., Reference Berntson, Lozano, Chen and Cacioppo2004). Measures of resting PEP and PEP reactivity were derived in the manner described above for RSA. Negative residual PEP scores are indicative of greater PEP reactivity (a decrease in PEP; PEP shortening or sympathetic activation) while positive residual scores are indicative of lower PEP reactivity (an increase in PEP; PEP lengthening or sympathetic deactivation).
Resting HR and HR reactivity
HR is influenced by dynamic interactions among the parasympathetic, sympathetic, and other cardiovascular reflexes (Porges & Furman, Reference Porges and Furman2011), and thus is not a “pure” measure of parasympathetic or sympathetic activity. Once RSA and PEP data were cleaned and scored, HR was calculated as the number of R-peaks in each 30-s epoch. Measures of resting HR and HR reactivity were derived in the manner described above for RSA and PEP. Positive HR residual scores are indicative of HR acceleration (i.e., a stress reactivity response) to the challenge protocol.
Cortisol
Saliva samples were collected using the Salimetrics Children's Swab (Salimetrics Inc., State College, PA, USA) which was placed in the children's mouth for approximately 30 s until saturated and then placed in a swab storage tube. Samples were then stored at −20°C until they were ready to be sent by courier on dry ice to a laboratory at the University of Trier, Germany to be assayed for cortisol. Assays were conducted in duplicate using a time-resolved fluorescence immunoassay (DELFIA; Dressendörfer, Kirschbaum, Rohde, Stahl, & Strasburger, Reference Dressendörfer, Kirschbaum, Rohde, Stahl and Strasburger1992). After thawing, samples were mixed and centrifuged, and cortisol was assayed using a commercial immunoassay with chemiluminesence detection (Cortisol Luminescence Immunoassy: IBL- Hamburg, Hamburg, Germany; detection limit of 0.179 nmol/l). Cortisol values were normalized using natural log transformation prior to conducting data analyses (McCarthy et al., Reference McCarthy, Hanrahan, Kleiber, Zimmerman, Lutgendorf and Tsalikian2009). Cortisol rest was defined as the cortisol value during the first collection before the start of the protocol. To measure cortisol reactivity, a standardized residual score was calculated by regressing the post-protocol cortisol value on the pre-protocol value, adjusting for time of day at the first sample. A positive residual score indicates heightened cortisol reactivity during the challenge protocol.
Executive functioning
Objectively measured executive functioning was assessed at a planned follow-up visit when the children were between 4 and 6 years old using the Minnesota Executive Functioning scale (MEFS; Carlson and Zelazo, Reference Carlson and Zelazo2014). In this standardized tablet-based assessment, children were seated in front of an iPad equipped with the MEFS software, a standardized assessment of executive functioning skills designed for children ages 2–7 years. In this task, children were presented with a set of cards that they had to sort into one of two boxes based on a set of increasingly complex rules. For example, children were instructed to sort five cards into the boxes according to one rule, and then to switch and sort five additional cards by an opposing or conflicting rule. The task was adaptive, with the starting point depending on the child's age, and task completion ranged between 2–7 min, depending on the child's response time and success rate. The MEFs taps working memory, inhibitory control, and cognitive flexibility and has high test–retest reliability (Carlson & Zelazo, Reference Carlson and Zelazo2017; Carlson & White, Reference Carlson, White and Taylor2013) and criterion validity (Prager, Sera, & Carlson, Reference Prager, Sera and Carlson2016). The MEFS has been nationally normed based on the child's age and standardized scores are automatically generated using an algorithm that combines accuracy and response time (M = 100, SD = 15).
Analytic plan
All LPAs were performed in Mplus Version 8 (Schultzberg et al., Reference Schultzberg, Muthén, Asparouhov and Hamaker2017). LPA models were constructed using eight physiological indicators (controlling for child age and sex): baseline HR, RSA, PEP, cortisol and HR, RSA, PEP, and cortisol reactivity. Multisystem physiological profiles have been previously validated in the current sample (Rudd et al., Reference Rudd, Bush, Alkon and Roubinov2021); however, as mentioned above, the current investigation uses task-specific controls rather than a general baseline task when calculating HR, RSA, and PEP reactivity. Because these LPA indicators are computed slightly differently from our prior study, we repeated model fitting procedures here to ensure that we conclude the appropriate number of latent profiles of multisystem physiology; however, we did not anticipate this would result in major changes to our previously derived multisystem physiological profiles. Model building and parameter constraint procedures directly followed those that were conducted in the previous study (Rudd et al., Reference Rudd, Bush, Alkon and Roubinov2021) and assessed latent profile solutions ranging from two to five profiles (i.e., allowing regulatory indicators to cluster in two to five distinct profiles). The Bayesian information criterion (BIC; Schwarz, Reference Schwarz1978) was primarily used to evaluate competing models, with lower BIC representing better fit. This strategy enables relative contrasts among both nested and nonnested models (West et al., Reference West, Taylor and Wu2012). Consistent with recommended procedures (Masyn, Reference Masyn and Little2013), we also assessed profile solutions on the basis of substantive interpretation, the proportion of the sample within each profile, the Akaike information criterion (AIC; Shibata, Reference Shibata and Willems1989), and model entropy (Ram & Grimm, Reference Ram and Grimm2009).
Following selection of the best fitting profile solution for children's multisystem physiological functioning, we employed the manual three-step Bolck–Croon–Hagenaars (BCH) method to assess how early life adversity is associated with the multisystem profiles and how multisystem profiles are associated with executive functioning in a single, comprehensive model. This method is the recommended approach to analyze structural associations between latent profile membership, causal predictors, and distal outcomes (Asparouhov & Muthén, Reference Asparouhov and Muthén2014; Nylund-Gibson, Grimm, & Masyn, Reference Nylund-Gibson, Grimm and Masyn2019). The BCH model was executed according to the protocol set forth by Nyland and colleagues (Nylund-Gibson et al., Reference Nylund-Gibson, Grimm and Masyn2019). The first step of this technique involves identifying the best fitting unconditional model and saving the posterior probabilities of modal profile membership as well as the error associated with each profile membership. In the second step, these classification errors for each individual are computed, and the inverse logit of the individual error rates are transformed into weights that are used in the third step of the distal calculation. In the third step, these weights are used, rather than the modal profile assignment, to predict the distal outcome while accounting for error. In the current study, distal outcome analysis yielded logistic regression tests of the predictor's effect on profile membership as well as estimates of mean differences in executive functioning outcomes across multisystem physiological profiles based on a Wald chi-squared test. For logistic analyses, the anticipatory arousal profile was used as the comparison group, since our hypotheses centered around this profile being the at-risk group compared to the others. This technique has many advantages over other distal outcome approaches, including being able to handle unequal variance in the outcome across profiles and increased stability in individual's latent profile membership across steps (Nylund-Gibson et al., Reference Nylund-Gibson, Grimm and Masyn2019).
Results
Preliminary analyses
Descriptive statistics for the current sample are presented in Table 1. On average, the children in the current sample experienced more than one type of early adverse experience (M = 1.20, SD = 1.23, Range = 0–5 event types) and had executive functioning scores that were slightly below national norms (M = 96.72, SD = 7.16). Bivariate correlations among all study variables are presented in Figure 2. Of note, children's experience of adverse events was not significantly associated with executive functioning at the bivariate level.

Figure 2. Bivariate relations among all study variables.
Table 1. Descriptive statistics of demographics, early adversity, physiological regulation, and executive functioning
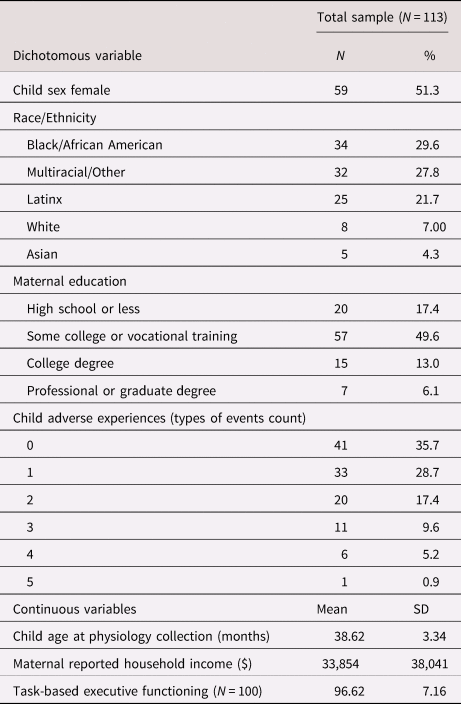
Model building for latent profile analysis
Model building contrasts generally revealed better fit for models specifying two- and three-profile solutions as compared to models specifying one, four, or five solutions, which had much larger BIC values (see Table 2). BIC of the two- and three-profile solutions were comparable, with the two-profile being smaller, but within the bounds of being considered negligible differences (Wasserman, Reference Wasserman2000). Smaller AIC values and comparable entropy supported our selection of the three-profile solution over the two-profile solution. Further, the three-profile models also had more meaningful and substantively interpretable profile distributions (e.g., comparable number of children within each profile) and patterns of indicator means (e.g., nonoverlapping confidence intervals) compared to the two-profile models. Based on these considerations, we retained the three-profile solution.
Table 2. Model fit indices for latent profile analyses (LPAs) with one to five profile solutions

Note: Bold indicates final retained profile solutions. AIC = Akaike information criterion, BIC = Bayesian information criterion.
Characteristics of the latent profiles
Characteristics for each latent profile are presented in Table 3 and Figure 3. Among the three profiles in the 36-month model solution, one profile comprised children who exhibited the highest cortisol reactivity and lowest resting cortisol across all profiles. Resting HR, PEP, RSA, and PEP reactivity were moderate and RSA reactivity was higher than both other profiles. This, pattern of heightened HPA axis reactivity bore similarity with the HPA axis responders profile derived in our previous study (Rudd et al., Reference Rudd, Bush, Alkon and Roubinov2021); thus, the same term was also used to describe children in this profile. A second profile included children whose multisystem functioning was characterized by the lowest resting HR, high resting RSA, and PEP comparable to that in the HPA axis responder profile, along with moderate HR, RSA, and PEP reactivity, and moderate cortisol rest and reactivity. Given these typically adaptive patterns of physiological functioning at rest, ANS and HPA reactivity to challenge, and the degree to which they bore similarity to our previous investigation, we adopted the same term (active copers/mobilizers) to describe children in this profile. The final profile was characterized by the lowest levels of resting RSA and highest resting HR across all profiles, which reflects heightened autonomic activation prior to the initiation of the stress protocol. Resting cortisol and cortisol reactivity were moderate and similar in magnitude to the active copers/mobilizers profile. Reflecting the heightened levels of arousal at rest, this final profile was termed anticipatory arousal/ANS responders. The patterning of this profile resembled that which was observed in our prior study and was named as such.

Figure 3. Graphical representation of three latent profile analysis models using standardized averages of latent indicator means.
Note: Resting values are graphed such that zero (the center line) is sample average values. Reactivity is graphed such that farther from zero (the center line) indicates greater reactivity. Positive values for HR and cortisol represent greater reactivity, while greater reactivity for respiratory sinus arrhythmia (RSA) and pre-ejection period (PEP) are represented by negative values.
Table 3. Profile-specific sample size, latent-indicator means, and confidence intervals of latent-indicator means for the final three-profile model

HPA = hypothalamic–pituitary–adrenal; HR = heart rate; PEP = pre-ejection period; RSA = respiratory sinus arrhythmia
Note: A lack of overlap in the confidence intervals for a given indicator among profiles suggests the profiles differ significantly on that indicator.
a Difference from the anticipatory arousal/ANS responders.
b Differences from all other profiles.
c Difference from the active copers/mobilizers profiles only.
d Difference from the HPA axis profile only.
Predictors and distal outcomes
Table 4 presents the means and standard deviations for study predictor and outcome variables across profiles. The three-step BCH approach assessed the associations between covariates (i.e., family income and maternal education), early life adversity, multisystem profile membership, and later executive functioning. There was not a direct effect between early adversity and executive functioning (p = .265). Logistic regressions within the prediction model evaluated whether early adversity was associated with profile membership (see Table 4). For every standard deviation increase in early adversity, children were 2.183 times more likely to be in the anticipatory arousal/ANS responders profile than the HPA axis responders’ profile (OR = 2.183; p = .028). and 1.972 times more likely to be in the anticipatory arousal/ANS responders profile than the active copers/mobilizers profile (OR = 1.972; p = .001). In other words, for each additional type of adversity experienced, children were more likely to respond to challenges at 36-months with a multisystem stress response characterized by patterns of an anticipatory arousal/ANS response. Of note, post-hoc comparisons between HPA axis responders and active copers/mobilizers profiles were not significantly different (p = .178).
Table 4. Class-specific means of the predictor and outcome, odds ratio comparisons by profile, and Wald test comparison by profile

Note: Odds ratio calculations are displayed for all pairwise comparisons. Although the anticipatory arousal/ autonomic nervous system (ANS) responders profile was the reference group for calculations in the final model, we re-calculated these values to display the odds of being in the anticipatory arousal/ANS responders profile to present findings in line with our hypotheses. Comparisons of executive functioning differences by profile were computed using a Wald chi-squared test.
* p < .05.
In terms of 36-month multisystem physiology relations with later executive function, there was a significant association with profile membership such that children in the anticipatory arousal/ANS responders profile had lower executive functioning scores (M = 93.65, SD = 8.67) than the active copers/mobilizers profile (M = 99.00, SD = 8.00; χ2 = 5.35, p = .033) but were not significantly different from the HPA axis responders profile (M = 97.49, SD = 6.93; χ2 = 3.84, p = .144). Post-hoc comparisons between HPA axis responders and active copers profiles were also not statistically different (χ2 = 1.51, p = .506).
Discussion
The current study provides an innovative evaluation of the developmental pathways from early adverse experiences to multisystem physiological regulation to variations in executive functioning within a racially/ethnically diverse and socioeconomically disadvantaged sample. To our knowledge, this study is the first to examine such associations using an LPA approach with multiple physiological indicators. Although there was no evidence for a direct association between children's experience of early adversity and executive functioning, early adversity significantly predicted physiological profile membership, and membership predicted differences in later executive functioning. Specifically, reports of exposure to more types of adverse events before 18-months of age was associated with increased likelihood of exhibiting a multisystem (parasympathetic, sympathetic, and HPA axis) anticipatory arousal/ANS responders response at 36 months, and this physiological response pattern was associated with the lowest executive functioning scores in our sample. Taken together, our findings suggest that a child's experience of early adversity may become biologically embedded within stress-relevant physiology in a manner that influences their later executive functioning, an important vulnerability factor for later development of psychopathology and other health concerns.
Notably, there were no main effects between children's early adverse experiences and their executive functioning at age 5. Instead of a direct association between types of adversity experienced and maladaptation in the sample on average of executive functioning, only membership in one physiological profile evidenced significant risk for such problems, which suggests that the majority of children in the current sample evidenced resilience in this domain. Although some previous studies have documented significant associations between various aspects of early stress and parent reports of executive functioning (DePrince et al., Reference DePrince, Weinzierl and Combs2009; Sheridan et al., Reference Sheridan, Peverill, Finn and McLaughlin2017), our results are consistent with other investigations that used objective assessments of executive functioning and found null results (Cohodes et al., Reference Cohodes, Chen, Lieberman and Bush2020; Giuliano, Roos, Farrar, & Skowron, Reference Giuliano, Roos, Farrar and Skowron2018). Such differences in associations between parent report and objective measurement of children's executive functioning may be partially related to measurement type, as subjective reports are potentially subject to parent's bias as they report on their own children's abilities. Findings may also reflect a context-specific nature of associations such that certain traumatic experience types may be more salient for specific domains of executive functioning (e.g., inhibitory control vs. working memory). Cohodes et al. did not find evidence for exposure-type specific associations with executive functioning, whereas DePrince et al. (Reference DePrince, Weinzierl and Combs2009) identified familial trauma (e.g., domestic violence, physical maltreatment) as an important predictor, but found no association with nonfamilial trauma (e.g., community violence, natural disasters) for a composite of executive functioning. Our measure of early adversity included reports of both familial and nonfamilial adversities, and the young age of the children resulted in relatively lower levels of types of adverse experiences, limiting our opportunity to explore whether variation in exposure type may have differentially associated with executive functioning.
Despite the lack of direct effects between early adversity and later executive functioning, findings suggest that multisystem physiological regulation may operate as a link between early adversity and deviations in executive functioning. Our analytic approach tested a comprehensive model in which adversity was associated with multisystem physiological functioning and multisystem physiological functioning, in turn, was associated with executive functioning. Although the manual BCH analytic method used here precludes the ability to formally test mediation, findings support a temporal patterning of relations of adversity to multisystem physiology to executive functioning. In particular, children who experienced more types of adverse life events in the first 18 months of life were more likely to respond to a series of challenge tasks with multisystem anticipatory arousal/ANS responders. The first few years of life are marked by heightened developmental plasticity across multiple domains (e.g., brain maturation, myelination, attachment), such as neural connections that mature rapidly during the first two years of life through processes of synaptic blooming (Fox & Rutter, Reference Fox and Rutter2010). This rapid expansion, as well as the subsequent pruning process wherein unused synaptic connections are reduced, is thought to be highly susceptible to environmental influences. Experiences of adversity during this time may be particularly impactful on both the structure and function of the developing brain. Further, given the brain's key role in regulating these interconnected stress response systems, adverse experiences that shape the brain during this time have downstream effects for physiological regulation. Similarly, overactivation of these stress-sensitive systems due to adverse exposures may lead to potentially persistent dysregulated physiological responses (Cicchetti & Walker, Reference Cicchetti and Walker2001; Jones-Mason et al., Reference Jones-Mason, Behrens and Gribneau Bahm2019; McEwen, Reference McEwen2008). In our study, we found that children with more types of adverse events were more likely to respond to our protocol at 36 months with an anticipatory arousal/ANS responders multisystem response. In other words, while listening to a neutral audio-recorded story that presented no obvious challenge, children in this group tended to have heightened arousal across multiple physiological systems, accompanied by low reactivity to challenge. This relative activation at rest may be indicative of hypervigilance, and relates to theoretical assertions that multisystem physiological patterns of “vigilance” develop in early contexts of high stress (Del Giudice, Ellis, & Shirtcliff, Reference Del Giudice, Ellis and Shirtcliff2011; Ellis et al., Reference Ellis, Oldehinkel and Nederhof2017). Remaining in heightened states of arousal when no challenge is present may be an adaptive process in order to navigate an adverse home environment such as those where caregivers may be unpredictable, violent, or absent. However, such long-term activation, especially when paired with low mobilization for challenge responses, may produce problematic mental health outcomes in children across a broad range of contexts (Alink et al., Reference Alink, van IJzendoorn, Bakermans-Kranenburg, Mesman, Juffer and Koot2008; Ottaviani et al., Reference Ottaviani, Thayer, Verkuil, Lonigro, Medea, Couyoumdjian and Brosschot2016).
As noted, we saw that those in the anticipatory arousal/ANS responders profile also had the lowest executive functioning scores in our sample. Previous research has suggested that moderate reactivity responses during executive functioning challenges may best support such abilities (Marcovitch et al., Reference Marcovitch, Leigh, Calkins, Leerks, O'Brien and Blankson2010), and although they displayed high arousal at baseline, those in the anticipatory arousal/ANS responders profile had relatively low reactivity to challenge tasks. Physiological responses support our abilities to cognitively engage with challenges by directing necessary internal resources to meet the task at hand, particularly with respect to attention and working memory (Hansen, Johnsen, & Thayer, Reference Hansen, Johnsen and Thayer2003). Similarly, insufficient reactivity responses may hinder executive functioning performance in the moment when reactivity responses interfere with the ability to flexibly and effectively process environmental stimuli. Interestingly, there were no significant differences in executive functioning between the HPA axis responders and the active copers/mobilizers profiles. These profiles had very similar values across ANS measures at rest and reactivity, with cortisol values across rest and reactivity as the main distinguishing characteristic. Perhaps ANS regulation, which has a faster response time and more closely reflects moment-to-moment regulation in response to task demands (Porges, Reference Porges2009), may be more salient for executive functioning processes that entail dynamic and flexible engagement with environmental stimuli. In fact, both the HPA axis responders and the active copers/mobilizers profiles evidenced these dynamic responses across rest and reactivity which may account for their similar average level of executive functioning scores.
The current finding that anticipatory arousal/ANS responders at 36 months predicted lower-than-average executive functioning at age 5 presents evidence for an important transdiagnostic pathway to later impairment. Importantly, our sample-level average of executive functioning was also lower than national averages, suggesting this group may be at risk for poor outcomes associated with low executive functioning. As described previously, executive functioning is a core adaptive process that underlies much of normative developmental progression and supports children's positive adaptation across cognitive, behavioral, and emotional domains (Moriguchi et al., Reference Moriguchi, Chevalier and Zelazo2016; Samuels et al., Reference Samuels, Tournaki, Blackman and Zilinski2016). However, deficits in executive functioning impinge on children's daily life as they navigate home, school (e.g., academic achievement), and community (e.g., social interactions) contexts, and have been linked to an increased risk for numerous psychopathological outcomes (Bloemen et al., Reference Bloemen, Oldehinkel, Laceulle, Ormel, Rommelse and Hartman2018; Rinsky & Hinshaw, Reference Rinsky and Hinshaw2011). Utilizing a “neural systems first” approach (Insel et al., Reference Insel, Cuthbert, Garvey, Heinssen, Pine, Quinn and Wang2010) to understand how early adverse experiences get under the skin to influence executive functioning may highlight underlying mechanisms that contribute to the full range of variation in symptomatology, from typical presentations to maladaptation. If replicated, early identification of an anticipatory arousal/ANS responders response to challenges in conjunction with a history of adverse life experiences could aid in precision medicine efforts for early targeted interventions.
Although not a primary focus of this study, evaluation of LPA models and profile characteristics showed they were largely similar to those of our previous study (Rudd et al., Reference Rudd, Bush, Alkon and Roubinov2021). This comparison is important because our previous investigation used general resting measures to calculate challenge reactivity (having a calming story read aloud while child sits quietly), while the current study used task-specific control to calculate reactivity. Utilizing task-specific control conditions allows for greater capture and adjustment for the motor activation associated with engaging in a specific task, such as speaking or pointing, and thereby reduces noise and produces a more accurate estimate of psychological challenge-related activation (Bush et al., Reference Bush, Alkon, Obradović, Stamperdahl and Boyce2011). Across both approaches, profiles of anticipatory arousal/ANS responders and HPA axis responders emerged. These profiles also bore similarity to past studies utilizing older samples of children (Roubinov et al., Reference Roubinov, Boyce, Lee and Bush2020). Studies utilizing multisystem physiological assessments are rare, and the current study offers an excellent opportunity for an important replication using a slightly different methodology. However, there were some differences in the current 36-month profile characterizations utilizing this approach. Using task-specific controls, we also observed an active copers/mobilizers profile (i.e., patterns typically associated with adaptive responses across all systems), whereas using a general baseline measure resulted in a moderate arousal profile (i.e., generally moderate levels across all systems with less reactivity than the active copers profile). By parsing out task-related motor activity confounding in this investigation, we may have provided more accurate psychological reactivity calculations with which to assess associations with early adversity and executive functioning.
Strengths and limitations
The current study has a number of unique strengths, including a longitudinal cohort of racially/ethnically and socioeconomically diverse children from birth to age 5 and physiological measures across multiple stress response systems. Having such rich and comprehensive data on a sizeable group of children is important for probing the complex factors influencing developmental trajectories following from adversity. As the first study to use multisystem LPA models to examine associations between early life adversity, PEP, RSA, cortisol, and executive functioning, this study provides a creative and informative model for the examination of other developmental outcomes as well. Our assessment of children's physiological functioning across multiple stress response systems and using indicators of both resting and reactivity expands upon previous single-system focused investigations. Finally, the current study employed a validated and nationally standardized executive functioning task that directly measured children's abilities, rather than relying on parent-report measures.
Despite these notable strengths, a number of limitations should be considered when interpreting these results. First, we employed the gold standard approach to measure antecedents and consequences of LPA within a singular model; however, the BCH method does not yield traditional estimates of mediation effects, leading us to use caution in our phrasing and avoid the term “mediated” in our descriptions. Future advances in analytic and physiological methods may aid in the ability to perform analyses that estimate latent profile characterizations and probe distal predictions within a mediation framework. Further, given robust evidence for children's physiologic functioning to serve as a moderator of the effects of social environment on child functioning (Bush & Boyce, Reference Bush and Boyce2016), it will be important for additional research to explore other ways in which adversity, multisystem physiology, and executive functioning may be associated, including models in which physiology may render children more sensitive to the negative consequences of adversity on executive functioning. Second, adversity occurs across multiple levels (e.g., familial, nonfamilial, community violence, poverty), and the necessary complexity of such models may be best supported by larger samples sizes with greater power to detect associations with multiple predictors and outcomes. The current study is among the first to employ these analytic procedures and has the power to detect relations with one profile, but future research with larger samples may be able to further elucidate profiles’ associations, particularly profiles that capture heightened HPA axis activity. Future research with large samples and methodological richness that explore these associations across time can more fully trace longitudinal links to pathways of multiple risk phenotypes and development of psychopathology. Specifically, studies should include other critical early contributors to profile membership and subsequent executive functioning, such as parenting quality and classroom contexts.
Conclusion
In sum, the current investigation provides important information about potential physiological associations from early adverse experiences to variations in children's executive functioning. Although children in the current sample experienced a range of early adverse experiences, there was no direct association between number of experiences and detriments in executive functioning for the sample on average, and membership in only one physiological profile evidenced significant risk for such problems, suggesting that a large portion of children in our sample have evidenced resilience in this domain. Our findings support recent assertions that adverse childhood experiences alone do not determine an individual's risk for later problems (Baldwin et al., Reference Baldwin, Caspi, Meehan, Ambler, Arseneault, Fisher and Poulton2021), and physiological regulation may be an important mechanism delineating such risk trajectories (Cicchetti, Reference Cicchetti2010). These findings provide an important first step in identifying integrated pathophysiological patterns that may aid in identifying early deviations from expected responses and developmental trajectories. Harnessing a neural-systems, multilevel approach to understanding how early adverse experiences get under the skin to influence executive functioning may aid in precision medicine approaches to intervention by highlighting early physiological dysfunction in conjunction with an adversity history as targets for tailored executive functioning interventions.
Acknowledgments
The authors wish to acknowledge Kim Coleman-Phox, Marialma Gonzales-Cruz, Yurivia Cervantes, Katie Blackburn, Zoe Caron, Alana Cordeiro, Vanessa Tearnan, and Jayme Congdon for their assistance with the SEED data collection as well as Matt Lee for his consultation on statistical analyses. We would also like to thank Drs. Elissa Epel, Nancy Adler, and Barbara Laraia, the principal investigators on the pregnancy study from which the current participant pool was drawn, for providing support for the offspring study and guidance on its design. We are also thankful to the families for their generous participation in this research.
Funding Statement
This work was supported by the National Heart, Lung, and Blood Institute (U01 HL097973; R01 HL116511-02), and the Lisa and John Pritzker Family Fund. Dr. Bush is the Lisa and John Pritzker Distinguished Professor in Developmental and Behavioral Health and also received support from the UCSF-UC Berkeley Schwab Dyslexia and Cognitive Diversity Center, the Lazlo N. Tauber Family Foundation, the Lisa Stone Pritzker Foundation, and the Toxic Stress Research Network funded by the JPB Foundation of New York. Dr. Rouboinov received funding from the National Institute of Mental Health (K23MH1137019) and the Brain and Behavior Foundation Young Investigator Program
Conflicts of Interest
None.