Introduction
In the last decades, increasing knowledge about the threats due to antibiotic resistance drove the livestock industry to promote antibiotic-free production(Reference Cervantes1). After the complete ban of in-feed antibiotic growth promoters in the European Union (Regulation (EC) No 1831/2003)(Reference Union2) and the ensuring of a judicious use of medically important antimicrobials in food-producing animals in the USA (‘Veterinary Feed Directive’, 2015)(3), a market for alternatives to antibiotic growth promoters grew increasingly(Reference Karavolias, Salois and Baker4). Animal products labelled as antibiotic-free (raised without antibiotics) or no antibiotic ever (animal never exposed to antibiotics or ionophores) found a rising marketing opportunity and exponentially increased over the years(Reference Gadde, Kim and Oh5), but these strategies are posing challenges in the management of pathologies in animal husbandry. In the current post-antibiotic era, botanicals have gained an increasing interest among feed additives, due to their important biological activities including anti-inflammatory, antioxidant, antimicrobial and immunomodulatory(Reference Windisch, Schedle and Plitzner6). Botanicals are also reported in the literature as phytogenic feed additives, phytonutrients, phytobiotics or plant-based feed additives, and they represent a wide group of compounds with different biological activities. On the basis of their origin, botanicals can be obtained by herbs and spices, from which it is possible to extract oils. Numerous methods have been developed to recover these molecules from raw plant material(Reference Hashemi and Davoodi7). In relation to the process of extraction, it is possible to identify the extracts as essential oils (EO) or oleoresins (OR). EO are a group of volatile aromatic compounds obtained by steam distillation or hydrodistillation as the most applied conventional methods, whereas OR are a mixture of EO and resins obtained by non-aqueous solvent extraction(Reference Shahzadi, Nadeem and Hanif8). All the aforementioned botanicals can be classified as plant extracts, meaning the products directly derived by extraction from the raw materials (dry or wet) obtained from herbs and spices. EO and OR are a mixture of active compounds that can vary widely in composition, depending on several factors including climate, plant nutrition and stress(Reference Carson, Hammer and Thormar9). The chemical diversity of compounds does not only occur between and within plant families and genera, but also within populations of a single plant species. The variability in composition of EO and OR, as well described by Figueiredo et al. (Reference Figueiredo, Barroso and Pedro10), depends on several physiological, environmental and geographical conditions. EO quality and yield are highly species-dependent and differ basing on the part of the plant used for extraction, such as flowers, green part, bark, wood, pericarp or seeds only, or roots(Reference Figueiredo, Barroso and Pedro10). This variability depends also on other physiological variations, such as the stage of development of the plant part used for the extraction (leaf, flower or fruit), as well as the seasonal difference in temperature, humidity and the stage of plant metabolism over the year(Reference Figueiredo, Barroso and Pedro10). Yield and composition are also extremely dependent on weather conditions as well as the type and composition of the soil. There are also several examples, as reported by Figueiredo et al. (Reference Figueiredo, Barroso and Pedro10), that highlight how the existence of several chemical phenotypes (chemotypes) is strongly related to geographic variations, such as different altitudes or different light exposure, along with genetic differences. Chemical composition and water ratio can be also affected by the process of extraction, the duration of this process or the different pre-extraction drying methods(Reference Hashemi, Khorram, Sohrabi, Hashemi, Khaneghah and de Souza Sant’Ana11). Moreover, some extracts could have a small amount of impurities associated with them. The content of heavy metals in plants may be affected by geochemical characteristics of soil or region where the plant is cultivated. Also rain, atmospheric dust, plant protective agents and fertilisers could be additional factors of plant contamination with heavy metals(Reference Abu-Darwish, Al-Fraihat and Al-Dalain12,Reference Lydakis Simantiris, Fabian and Skoula13) . Among feed additives, another possibility is to use the pure bioactive compounds composing EO/OR, such as the chemically synthesised counterparts of these pure bioactives, referring to them as nature-identical compounds (NIC) or pure botanicals or phytochemicals. The difference in using NIC, rather than plant extracts, is represented by the fact that the former are single pure molecules. By using pure molecules or blends of molecules whose composition is known, the amount of inclusion in the feed is consequently precise. Furthermore, it is possible to formulate products by selecting the most effective compounds and combining them to obtain potential synergies. The present review aims to provide an overview of the effects exerted by botanicals employed as a nutritional strategy on the health status and growth performance of poultry and pigs. Attention is focused on studies in which only NIC were used or studies were the composition of the extracted oil was defined, therefore where the percentage of each active compound was specified. On the other hand, studies using plant extracts without a described composition were excluded because the effects on the animals were not ascribable to a specific concentration of bioactive molecules. In the literature there are already some reviews about the effects of phytogenic feed additives in poultry and pigs, but discrimination has not been made between plant extracts and NIC(Reference Windisch, Schedle and Plitzner6,Reference Murugesan, Syed and Haldar14–Reference Omonijo, Ni and Gong17) .
Botanical properties with relevance to animal nutrition
In the livestock industry one of the aims is to target the main intestinal inflammation pathologies in order to have healthier animals and, as a consequence, better zootechnical performance(Reference Murugesan, Syed and Haldar14,Reference Omonijo, Ni and Gong17) . In this context, there are many botanical properties of great interest like antimicrobial (including antibacterial, antifungal, antiviral and antiprotozoal), anti-inflammatory, antioxidant and immunomodulatory, as well as interest in the impact on gut functions and gut morphology as a consequence of said properties.
Antimicrobial activity
There are hundreds of reports about the in vitro antimicrobial power of bioactive compounds of botanicals, including activity against bacteria, fungi, viruses and protozoa(Reference Carson, Hammer and Thormar9). The antimicrobial mode of action of individual components of EO can have a single target or address multiple targets(Reference Nazzaro, Fratianni and De Martino18). Terpenes interact with the cell surface of bacteria(Reference Nazzaro, Fratianni and De Martino18), binding phospholipids and leading to the disaggregation of membrane structures and to ion leakage, reduction of membrane potential, collapse of the proton pump and depletion of the ATP pool(Reference Ultee, Bennik and Moezelaar19). In addition to interacting with membrane phospholipids, interference with membrane proteins and intracellular targets are also suggested(Reference Burt20,Reference Bakkali, Averbeck and Averbeck21) . Uncoupling of oxidative phosphorylation, inhibition of active transport, loss of pool metabolites, and disruption of synthesis of DNA, RNA, proteins, lipids and polysaccharides might follow(Reference Zengin and Baysal22). Several classes of botanicals, such as terpenes as well as polyphenols, aldehydes and organosulfur compounds, are able to inhibit the bacterial cell division protein FtsZ, a prokaryotic homologue of tubulin. FtsZ plays a key role in bacterial cell division by assembling into a contractile ring (Z-ring) at the midcell site of the future septum, so the suppression of FtsZ expression and the consequent Z-ring formation leads to the inhibition of bacterial cell division(Reference Hwang and Lim23). Moreover, the same classes of compounds are quorum sensing (QS) inhibitors. QS is a bacterial intracellular communication system that allows different activities such as virulence factor expression and biofilm formation(Reference Nazzaro, Fratianni and Coppola24). These cytotoxic effects were investigated for different bioactive compounds, as well summarised by Carson & Hammer(Reference Carson, Hammer and Thormar9) and Nazzaro et al. (Reference Nazzaro, Fratianni and De Martino18), and as described more in detail in the following paragraphs. Nevertheless, Diego Garcìa-Gonzalo’s research team(Reference Chueca, Berdejo and Gomes-Neto25,Reference Berdejo, Chueca and Pagán26) found that the use of some EO and their single terpenoid constituents at sub-inhibitory doses, for a prolonged time in vitro, facilitated the selection of mutant strains displaying an increased minimal inhibitory concentration(Reference Chueca, Berdejo and Gomes-Neto25,Reference Berdejo, Chueca and Pagán26) , underlining the importance of the inclusion of active principles. Regarding antiprotozoal action, this is ascribable mainly to organosulfur compounds and to some terpenes. It is due to the disruption of the flagellar membrane, mitochondrial swelling, and alteration in the organisation of the nuclear and kinetoplast chromatin, as seen by electron microscopy(Reference Anthony, Fyfe and Smith27). On the other hand, some individual constituents of botanicals, at sub-inhibitory doses, were proved to facilitate the selection of resistant strains in vitro (Reference Chueca, Berdejo and Gomes-Neto25,Reference Berdejo, Chueca and Pagán26) . Plant extracts contain many compounds in variable amounts and this reduces the risk of inducing resistance in bacteria, while using pure compounds this risk does exist at a sublethal level. These findings suggest avoidance of sub-inhibitory concentrations of bioactives and underline the importance of selecting the right inclusions of active principles, as well as the importance of selecting different components that can act in synergy.
Antiviral activity of some bioactive compounds, mostly terpenes or aldehydes, was also tested in vitro, mainly against the influenza virus, as reviewed by Carson & Hammer(Reference Carson, Hammer and Thormar9). The antiviral mode of action can be attributed to the aforementioned ability to disrupt membranes, so that viral envelopes may also be disrupted by botanical active ingredients(Reference Carson, Hammer and Thormar9).
Immunomodulatory, anti-inflammatory and antioxidant activity
Several botanical bioactives reported an immunomodulatory effect mediated in the host, either as immunosuppressors or as immunostimulators(Reference Anastasiou and Buchbauer28). The possible immunomodulatory action of phytochemicals is also connected to their antioxidant and anti-inflammatory activity. Different classes such as polyphenols, terpenes, organosulfur compounds and aldehydes are reported to have the ability to scavenge free radicals(Reference Salehi, Mishra and Nigam29–Reference Huang and Lee32). In fact, polyphenols seem to have a role in regulating expression of pro-inflammatory mediators and enzymes involved in the NF-κB and mitogen-activated protein kinases signalling pathways, while terpenes modulate the anti-inflammatory response by inhibiting lipoxygenase, prostaglandins and leukotrienes(Reference Miguel31). Some aldehydes and polyphenols also inhibit the secretion of pro-inflammatory cytokines(Reference Salehi, Mishra and Nigam29,Reference Miguel31) . Furthermore, polyphenols and some terpenes reduce excessive oxidative stress in pigs, by reducing plasma lipid peroxidation and lowering malondialdehyde (MDA) levels(Reference Qin and Hou33). Indeed, there is a tight connection between antioxidant and anti-inflammatory function, as reactive oxygen species (ROS) are involved in a wide spectrum of diseases, including chronic inflammation(Reference Reuter, Gupta and Chaturvedi34). This means that the antioxidant mechanism of action mediated by botanical components could also be the explanation of their anti-inflammatory properties.
These properties enhance the general health status of animals, thereby improving intestinal health. Various aromatic plant bioactives were reported to exert beneficial effects in animals by improving gut morphology and expression of tight junction proteins(Reference Grilli, Tugnoli and Passey35–Reference Sun, Lei and Wang38), promoting the integrity of the intestinal barrier(Reference Grilli, Tugnoli and Passey35,Reference Zou, Xiang and Wang37) .
Role in the chemosensory system
There is a growing evidence that botanicals play a role in the chemosensory system by the activation of transient receptor potential (TRP) channels(Reference Lee, Buber and Yang39). TRP channels are expressed thorough the digestive tract and play several roles, for example in taste, chemosensation, mechanosensation, pain and control of motility by neurons(Reference Holzer40). They have been identified as mediators for the taste sensations of several spicy molecules. The involved TRP channels are TRPV1 (curcumin, allicin, capsaicin), TRPA1 (allicin, thymol, cinnamaldehyde) and TRPV3 (thymol, carvacrol, vanillin)(Reference Lee, Buber and Yang39,Reference Xu, Delling and Jun41,Reference Zhi, Dong and Kong42) . Recent advances in TRP research started to underline their role in immune and inflammatory cells and in immune-mediated diseases. For this reason, perturbation of homeostatic TRP channels functionality may contribute to various pathological conditions(Reference Parenti, De Logu and Geppetti43), so their activation by botanical components is gaining increasing interest.
Practical aspects of botanicals as feed additives
Beside the aforementioned positive effects, there are some limitations to the use of these compounds as feed additives.
First and foremost, botanicals are susceptible to rapid degradation in the stomach and consequent low availability at the intestinal level. In relation to this aspect, appropriate releasing techniques could be necessary. Michiels et al. (Reference Michiels, Missotten and Dierick44) showed how terpenes and aldehydes are mainly and almost completely absorbed in the stomach and in the proximal small intestine of piglets, revealing the difficulty in reaching the lower part of the intestine. To overcome this limitation, some research groups investigated encapsulation or other coating technologies in order to minimise absorption and degradation in the proximal intestinal tract. For example, Grilli et al. (Reference Grilli, Tugnoli and Passey35,Reference Grilli, Vitari and Domeneghini45,Reference Grilli, Messina and Tedeschi46) fed weaning piglets or broilers with a mixture of NIC and organic acids microencapsulated in a matrix of hydrogenated fats, observing an effect of the active ingredients along the whole intestinal tract. As another example, David Nisbet’s research group(Reference Beier, Petrujkic and Sedej47) investigated the use of a terpene glycoside form compared with the free form of the same terpene. They observed that the glycoside form showed 60 % higher resistance in absorption in pigs(Reference Petrujkić, Sedej and Beier48) and antimicrobial activity in the crops in poultry(Reference Epps, Harvey and Byrd49). Moreover, Hébert et al. (Reference Hébert, Yuan and Dieter50) demonstrated that the microencapsulation of an aldehyde allowed the delivery of a higher dose, avoiding the acute toxic effects of a bolus dose. Microencapsulation of botanicals allows slow release of the active ingredients, preventing the immediate absorption of such compounds upon exiting the stomach(Reference Piva, Pizzamiglio and Morlacchini51). The longer permanence along the gastrointestinal tract of active compounds allows them to act on intestinal morphology and microflora and shows a prolonged local availability of the bioactive compounds for the host(Reference Piva, Pizzamiglio and Morlacchini51). Moreover, encapsulation protects these molecules from degradation induced by external agents, such as light, temperature and pH variations. Despite botanicals being usually listed as a flavorant, some of them can have an unfavourable effect on feed intake because of their pungency. Also in this context, encapsulation plays another important role in taste-masking(Reference Rassu, Nieddu and Bosi52).
Nature-identical compounds and bioactives of plant extracts as a tool to improve zootechnical performance
The number of papers published on the use of botanicals as feed additives has increased dramatically in the last decades. A wide variety of herbs and spices have been used in poultry and pigs(Reference Omonijo, Ni and Gong17,Reference Lillehoj, Liu and Calsamiglia53) , but the large quantity of confusing data makes it difficult to associate specific effects to specific bioactive compounds. For this reason, the positive effects on performance and general health status of poultry and pigs are here reviewed by selecting only those studies where animals were fed with NIC or where the composition of the EO/OR was defined, more specifically where a specific percentage of active compounds was indicated. The selected studies are only in vivo trials where a negative control was always present (no botanicals in the feed) and where the following effects were investigated: zootechnical performance, susceptibility to infection during microbial challenges, and response in terms of health status (inflammatory and oxidative response, immune response, effects on intestinal health). In vitro studies were only mentioned to describe the specific mechanism of action of EO single components and NIC, in order to better understand the effects in vivo. When a compound was fed in a blend and/or encapsulated in a matrix, the precise concentration of the bioactive ingredient was calculated, referring to the percentage of inclusion specified. Moreover, to allow a better comparison among the studies, all the inclusions in the present review are reported in mg/kg. Our attention was focused on phenolic compounds (apigenin, quercetin, curcumin and resveratrol), organosulfur compounds (allicin), terpenes (eugenol, thymol, carvacrol, artemisinin and capsaicin) and aldehydes (cinnamaldehyde and vanillin), as described below and summarised in Table 1.
Table 1. Pure botanicals used as feed additives in poultry and pigs and their effect on general health status and growth performance*
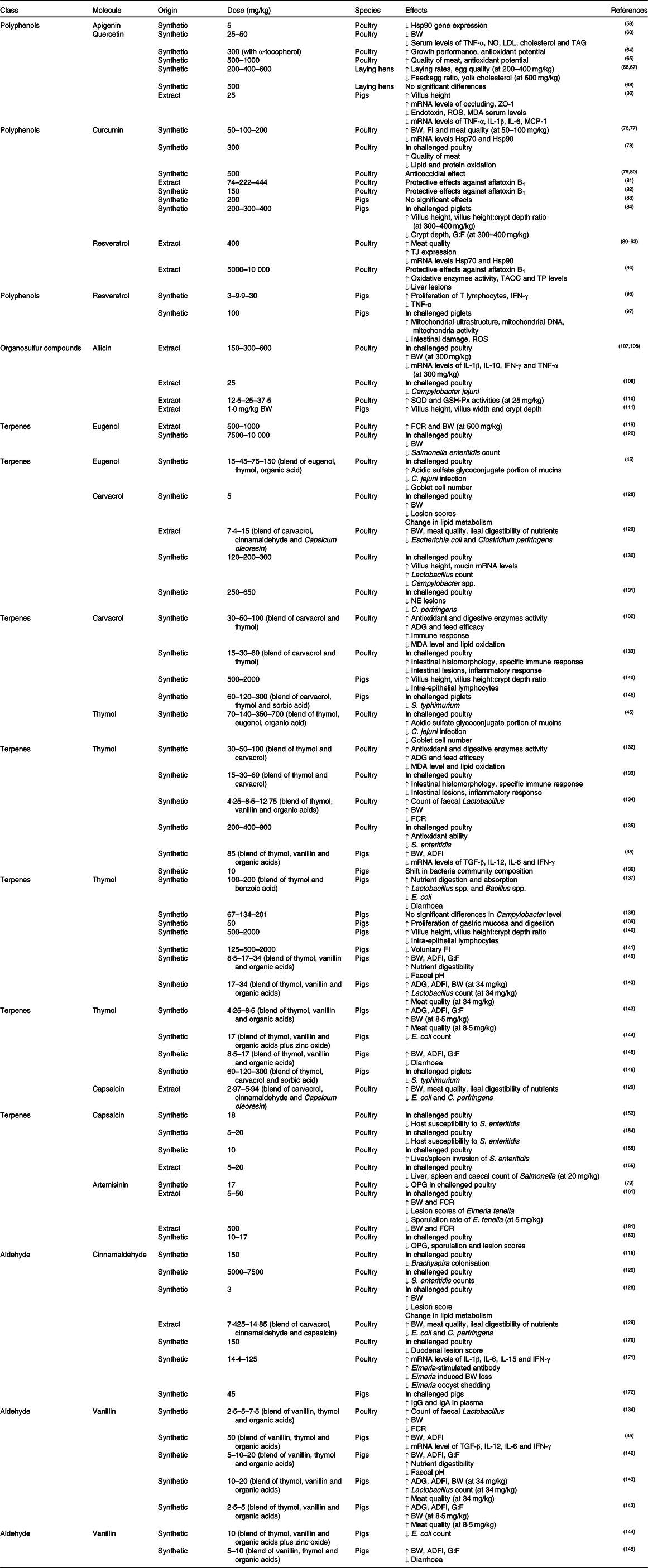
Hsp, heat shock protein; BW, body weight; ZO-1, zonula occludens-1; ROS, reactive oxygen species; MDA, malondialdehyde; MCP-1, monocyte chemotactic protein-1; FI, feed intake; G:F, gain:feed ratio; TJ, tight junctions; TAOC, total antioxidant capacity; TP, total protein; IFN-γ, interferon-γ; SOD, superoxide dismutase; GSH-Px, glutathione peroxidase; FCR, feed conversion rate; NE, necrotic enteritis; ADG, average daily gain; ADFI, average daily feed intake; TGF-β, transforming growth factor β; OPG, oocysts shedded per g of faeces.
* Only nature-identical compounds (synthetic origin) or plant extracts with a defined composition were taken into consideration. When a blend of compounds was fed, or when compounds were encapsulated in a matrix, the reported dose refers to the specific inclusion of the single compound in the mixture. All the inclusions were equalised to mg/kg.
Polyphenols
Polyphenols constitute the largest class of dietary phytochemicals and they possess anti-inflammatory, antiviral and antioxidant properties(Reference de Cássia da Silveira e Sá, Andrade and de Sousa54). Thanks to their aromatic rings, polyphenols directly react with hydroxyl, alkoxyl and lipid peroxyl radicals (ROO) and form water and a stable radical with the ability to delocalise the unpaired electron(Reference Haslam55). Because of these activities, flavonoids are considered putative nutraceuticals that may help, prevent or treat chronic inflammatory conditions(Reference Mascaraque, González and Suárez56).
Apigenin
Apigenin (4',5,7-trihydroxyflavone) is naturally found in parsley and celery and has attracted attention because of its anti-inflammatory activities both in vitro and in vivo (Reference Bisht, Wagner and Bulmer57). Apigenin (5 mg/kg) caused a reduction in heat shock protein 90 (Hsp90) gene expression in chickens(Reference Iqbal, Liu and Nabi58) through a mechanism that involves direct binding to the C-terminal region of Hsp90(Reference Begum, Prasad and Thayalan59). Since abnormal levels of Hsp90 have been observed in inflamed tissues, apigenin might regulate intestinal inflammation.
Quercetin
The flavonoid quercetin (3,3',4',5,7-pentahydroxyflavone) exhibits various biological effects such as antioxidant, anti-inflammatory, antiviral, antibacterial, anticoccidial and anti-apoptotic(Reference Anand, Arulmoli and Parasuraman60,Reference del Cacho, Gallego and Pages61) . The antimicrobial power of quercetin appears to be related to its ability to reduce the expression of genes involved in QS and by inhibiting bacterial biofilm formation(Reference Ta and Arnason62), whereas the antioxidant activity is attributed to its ability to scavenge free radicals or electrons or chelate metal cations. Female chickens fed with an inclusion of 25 or 50 mg/kg of quercetin alone or combined with δ-tocotrienol showed reduction in body weight (BW) but, on the other hand, a reduction in TNF-α, serum NO, serum total cholesterol, LDL-cholesterol and TAG levels was also observed(Reference Qureshi, Reis and Qureshi63). Sohaib et al. (Reference Sohaib, Butt and Shabbir64) assessed the effects of quercetin (300 mg/kg) in broilers, resulting in improved growth performance and antioxidant potential (increased stability of lipid against oxidation in meat). Similar results, in terms of breast meat quality and antioxidant potential, were reported supplementing 500–1000 mg of quercetin/kg of feed(Reference Goliomytis, Tsoureki and Simitzis65). Liu et al. (Reference Liu, Li and Liu66,Reference Liu, Liu and Hu67) found that supplementation of quercetin (200, 400 and 600 mg/kg) improved laying hen performance and egg quality, with an increase in laying rates, while reductions in the feed:egg ratio and yolk cholesterol were registered. In contrast, no differences in laying hen performance were reported from Iskender et al. (Reference Iskender, Yenice and Dokumacioglu68) with 500 mg/kg of quercetin. Concerning pigs, quercetin exerts a protective effect on intestinal integrity. In particular, supplementation of 25 mg/kg of quercetin in finishing pigs increased villus height (VH) and mRNA levels of occludin and zonula occludens-1. On the other hand, quercetin reduced concentrations of endotoxin in the serum, ROS and MDA and mRNA levels of TNF-α, IL-1β, IL-6 and monocyte chemotactic protein-1(Reference Zou, Wei and Xiang36).
Curcumin
Curcumin is a polyphenol that has anti-inflammatory, antioxidant (ROS scavenging and inhibition of lipid peroxidation), anti-proliferative, antibacterial and anthelminthic properties(Reference El-Bahy and Bazh69,Reference Boroumand, Samarghandian and Hashemy70) . Its antibacterial activity is related to the action on different targets: damaging of bacterial membrane(Reference Tyagi, Singh and Kumari71), inhibition of biofilm formation of many pathogens(Reference Ta and Arnason62,Reference Kali, Bhuvaneshwar and Charles72) , and inhibition of the assembly of the division protein FtsZ in Staphylococcus and Enterococcus (Reference Rai, Singh and Roy73). The strong anti-inflammatory power is due to the ability of curcumin to inhibit the transcription factors NF-κB and cyclo-oxygenase-2, with the consequent inhibition of the secretion of TNFα and other pro-inflammatory cytokines(Reference Kumar, Ahuja and Sankar74,Reference Vecchi Brumatti, Marcuzzi and Tricarico75) . For this reason, there are several studies focused on the administration of curcumin as an anti-inflammatory agent in the intestine. Some studies demonstrated that dietary curcumin supplementation (50, 100 and 200 mg/kg) in broiler chickens prevented heat stress-impaired growth performance, possibly through improving the antioxidant defence system(Reference Zhang, Hu and Lu76,Reference Zhang, Hu and Lu77) . The same levels of curcumin reduced the gene expression of Hsp70 and Hsp90(Reference Zhang, Hu and Lu76). Rajput et al. (Reference Rajput, Ali and Naeem78) reported reduction of lipid and protein oxidation and improvement of quality of breast meat in coccidiosis-infected chickens fed with 300 mg/kg of curcumin. The curcumin anticoccidial effect was detected only at 500 mg/kg.(Reference Allen, Danforth and Augustine79,Reference Peek, Halkes and Mes80) Moreover, protective effects at various concentrations, as shown in Table 1, are reported in poultry fed with aflatoxin B1 (Reference Gowda, Ledoux and Rottinghaus81,Reference Zhang, Qi and Zhao82) . Concerning swine, Ilsley et al. (Reference Ilsley, Miller and Kamel83) and Xun et al. (Reference Xun, Shi and Zhou84) reported that administration of 200, 300 or 400 mg/kg of curcumin did not affect the growth of piglets infected with enterotoxigenic Escherichia coli. Decreasing in crypt depth and increasing in VH and VH:crypt depth ratio were also reported(Reference Xun, Shi and Zhou84).
Resveratrol
Resveratrol (3,5,4'-trihydroxystilbene) has been largely described as exhibiting anti-inflammatory, antioxidant (ROS scavenger) and cell growth-modulatory effects(Reference Araújo, Gonçalves and Martel85,Reference Ergün, Ergün and Öktem86) . Some molecular mechanisms have been suggested to explain the effects of resveratrol including the down-regulation of the transcription factors NF-κB and activator protein 1, the down-regulation of pro-inflammatory cytokines and the interaction with drug-metabolising enzymes(Reference Salehi, Mishra and Nigam29,Reference Das and Das87,Reference Szkudelska and Szkudelski88) . Antimicrobial power was also reported in vitro due to the ability of resveratrol to inhibit bacterial biofilm formation(Reference Ta and Arnason62) as well as Z-ring formation inhibition via suppression of FtsZ expression in E. coli (Reference Hwang and Lim23). Heat-stressed broilers treated with 400 mg/kg of resveratrol showed increased expression of intestinal tight junctions, re-establishment of small-intestinal microbial profiles(Reference Zhang, Zhao and Wang89,Reference Zhang, Zhao and Yang90) , reduction of gene expression of Hsp70 and Hsp90(Reference Liu, He and Xie91) and beneficial effects on meat quality(Reference Zhang, Wang and Zhao92,Reference Zhang, Yang and Zhao93) . Furthermore, resveratrol (5000–10 000 mg/kg) reduced aflatoxin B1-induced changes in broilers in terms of oxidative damage by improving plasma total antioxidant capacity and total protein, and reducing liver lesions(Reference Sridhar, Suganthi and Thammiaha94). For pigs, Fu et al. (Reference Fu, Cui and Yang95) reported an immunostimulant effect in piglets, with positive effects on the activation and proliferation of T lymphocytes, up-regulation of interferon-γ and down-regulation of TNF-α with inclusion of 3, 9·9 or 30 mg of resveratrol/kg of feed. The positive effect of this compound in weaned piglets was also connected to an enhancement of barrier function, with increased transepithelial electrical resistance, as demonstrated by an in vitro trial studying two porcine cell lines, IPEC-1 and IPEC-J2(Reference Ling, Wan and El-Nezami96). Cao et al. (Reference Cao, Shen and Wang97) found that the supplementation of 100 mg/kg of resveratrol in piglets attenuated intestinal damage induced by oxidative stress, protected intestinal mitochondria functionality by decreasing ROS production and increasing mitochondrial DNA content. These findings imply that resveratrol can induce mitophagy in the intestine to alleviate the intestinal barrier dysfunction induced by oxidative stress(Reference Cao, Shen and Wang97).
Organosulfur compounds
This class of molecules comprise alliin, allicin and its derived compounds like diallyl trisulfide, diallyl disulfide, diallyl sulfide and allyl methyl disulfide. Some organosulfur compounds have shown antiparasitic activity. Among them, ajoene, a sulfur-rich molecule from garlic, seems to interfere with protein and lipid trafficking in the parasite and host cell membranes, irreversibly damaging the parasite(Reference Anthony, Fyfe and Smith27). Moreover, ajoene lowers the expression of important genes involved in the bacterial QS(Reference Jakobsen, van Gennip and Phipps98). Several studies have also observed that these compounds exert antioxidant and anti-inflammatory properties, via modulating antioxidant-related enzymes such as 3-hydroxy-3-methylglutaryl-CoA reductase, glutathione S-transferase and catalase(Reference Borek99). To our knowledge there are no studies where single pure compounds were used in poultry and pigs, so in this section we have listed only those studies where the exact composition of the organosulfur compound was specified.
Allicin
Allicin (S-allyl-2-propenyl thiosulfinate) is the main biologically active component of garlic (Allium sativum). Allicin possesses various biological activities among which antiviral, antimicrobial, antifungal and antiparasitic are included(Reference Agarwal100,Reference Alpers101) . Again, it is known as an activator of TRPA1 and TRPV1 channels(Reference Macpherson, Geierstanger and Viswanath102), which are implicated in inflammation, infection and immunity(Reference Fernandes, Fernandes and Keeble103). In addition, allicin has an immunomodulatory function, modulating the NF-κB transcription pathway(Reference Ban, Yuk and Woo104,Reference Gu, Wu and Fu105) and preventing oxidative damage by scavenging ROS(Reference Capasso106). Wang et al. (Reference Wang, Jiao and Zhao107) found that allicin supplemented at 300 or 600 mg/kg significantly ameliorated the retarded BW in reticuloendotheliosis virus-infected chickens, with a partial alleviation of the immune dysfunction induced by the infection. Moreover, allicin down-regulated the expression of IL-1β, IL-10, INF-γ and TNF-α. These results are in accordance with the previous study of this research group, which demonstrated that allicin (300 mg/kg) could improve the immune function of chickens(Reference Liang, Wang and Wang108). Robyn et al. (Reference Robyn, Rasschaert and Hermans109) treated chicks via drinking water supplemented with allicin at a final concentration of 25 mg/kg, then chickens were subsequently orally inoculated with 103 colony-forming units/ml of Campylobacter jejuni. There was an observable trend for lower caecal Campylobacter jejuni numbers in broilers raised on allicin-containing drinking water, but no significant difference in BW. Moreover, allicin showed increased antioxidant capacity in chickens fed with 25 mg of allicin/kg of feed; in particular, superoxide dismutase and glutathione peroxidase activities increased(Reference Gong-chen, Lu-lu and Jing110). Allicin, dispensed to weaned piglets at 1·0 mg/kg BW, improved the development of the gastrointestinal tract in terms of villus width, VH and crypt depth(Reference Tatara, Tomaszewska and Dudek111).
Terpenes
Terpenes are a large group of natural bioactive compounds including monoterpenes, diterpenes, triterpenes, hemiterpenes and sesquiterpenes, according to the number of isoprene units. Several terpenoids have pharmacological and biological activities and are therefore interesting for medicine and biotechnology(Reference Singh and Sharma112). Monoterpenes are present in many EO and medicinal plants(Reference de Cássia da Silveira e Sá, Andrade and de Sousa54), with eugenol, thymol and carvacrol as the most studied molecules. Toxic effects on membrane structure and function generally highlight the antimicrobial action of monoterpenoids. In fact, as a result of their lipophilic character, monoterpenes preferentially partition from an aqueous phase into membrane structures. This leads to membrane expansion, increased membrane fluidity and permeability, disturbance of membrane-embedded proteins, inhibition of respiration and alteration of ion transport processes(Reference Sikkema, de Bont and Poolman113).
Eugenol
Eugenol is a phenolic monoterpene extracted from cloves (Syzigium aromaticum) and basil (Ocimum basilicum). It is largely used for its antimicrobic, antiviral, anthelmintic, antioxidant and anti-inflammatory properties(Reference Jaganathan, Supriyanto and Jaganathan114). The anti-inflammatory action of eugenol arises from inhibiting prostaglandin synthesis and neutrophil/macrophage chemotaxis. The in vitro antibacterial activity of eugenol against pathogens is well documented and explained by alteration of bacterial membranes and of ions and ATP transport(Reference Nazzaro, Fratianni and De Martino18,Reference Upadhyay, Arsi and Wagle115,Reference Verlinden, Pasmans and Mahu116) , inhibition of bacterial virulence factors and biofilm formation(Reference Nazzaro, Fratianni and De Martino18), as well as inhibition of QS(Reference Ta and Arnason62,Reference Sybiya Vasantha Packiavathy, Agilandeswari and Musthafa117) , even at sub-inhibitory concentrations(Reference Zhou, Zheng and Tang118). Scherer et al. (Reference Scherer, Bogusz and de Albuquerque119) demonstrated that the addition of 500 or 1000 mg/kg of microencapsulated eugenol in poultry improved the feed conversion rate (FCR) and increased weight gain compared with control, equalling the diet supplementated with 10 mg/kg of avilamycin. Discordant results regarding BW were reported by Kollanoor-Johny et al. (Reference Kollanoor-Johny, Mattson and Baskaran120), finding that supplementation with 7500 and 10 000 mg/kg of eugenol decreases the final BW of poultry, but consistently decreased Salmonella enteritidis count in challenged chickens, with a significant reduction of the pathogen populations in the caecal samples. Different results for BW are probably linked to the concentration of eugenol used. Grilli et al. (Reference Grilli, Vitari and Domeneghini45) demonstrated that the combination of a microencapsulated blend of eugenol with thymol and organic acids allowed a significant reduction in Campylobacter jejuni infection in broiler chickens, starting with an inclusion of 15 mg/kg. Even histochemical results showed that the treatment with 45 mg/kg was able to reduce goblet cell number and to increase the acidic sulfated glycoconjugate portion of mucins, supporting the highly protective role of the intestinal mucus against pathogenic micro-organisms.
Carvacrol and thymol
Carvacrol and its isomer thymol represent the major components of thyme (Thymus vulgaris) and oregano (Origanum vulgare) EO. They have been extensively studied for their bactericidal activity as bacterial membrane permeabilisers. In particular, carvacrol causes the collapse of the proton-motive force and depletion of ATP pools, and inhibits bacteria motility by preventing the synthesis of flagellin, the protein that composes flagella(Reference Burt, van der Zee and Koets121). Zengin & Baysal(Reference Zengin and Baysal22) investigated the effects of some terpenes on pathogenic bacteria (Staphylococcus aureus, Salmonella typhimurium, E. coli O157:H7) by scanning electron microscopy. They observed morphological and structural changes: pores in the outer membrane, leakage of the intracellular material and altered permeability of the cell membrane with different susceptibility of the pathogens, according to their lipidic composition of the cell wall(Reference Nazzaro, Fratianni and De Martino18,Reference Lambert, Skandamis and Coote122) . In addition, carvacrol and thymol showed also antibiofilm and anti-QS activities(Reference Nazzaro, Fratianni and Coppola24). Thymol alters different pathways in the cell metabolism of pathogens like Salmonella enterica, even at sublethal concentrations(Reference Di Pasqua, Mamone and Ferranti123). In addition to this well-studied antimicrobial power, it is coming to light how these phenolic compounds also play a role in the integrity of the intestinal mucosa because of their anti-inflammatory and antioxidant properties(Reference Guimarães, Xavier and de Santana124,Reference Shen, Huang and Liao125) . Many studies report a strong antioxidant activity of carvacrol and thymol, and this is related to the fact that phenolic compounds have redox properties; they play an important role in scavenging free radicals and peroxynitrite, and they inhibit lipid peroxidation(Reference Boudry and Perrier126,Reference Braga, Dal Sasso and Culici127) . Lillehoj et al. (Reference Lillehoj, Kim and Bravo128) found enhanced BW and reduced gut lesions in broiler birds fed a diet supplemented with a specific blend (5 mg/kg of carvacrol, 3 mg/kg of cinnamaldehyde and 2 mg/kg of Capsicum oleoresin) and orally challenged with Eimeria acervulina. Findings were also confirmed by Jamroz et al. (Reference Jamroz, Orda and Kamel129). Broilers challenged with Campylobacter jejuni and treated with different concentration of carvacrol (120, 200 and 300 mg/kg) resulted in an increased abundance of Lactobacillus bacteria in broiler caeca and in the reduction of Campylobacter spp. presence(Reference Kelly, Gundogdu and Pircalabioru130). In the same study, concerning gastrointestinal morphology, histological analysis at slaughter indicated an increase in small-intestinal VH, and the increased expression of mucin mRNA in both large and small intestines. Liu et al. (Reference Liu, Diarra and Zhang131) found that adding encapsulated carvacrol (250 and 650 mg/kg) appeared to be effective in lessening the severity of necrotic enteritis lesions. Hashemipour et al. (Reference Hashemipour, Kermanshahi and Golian132) administered a mixture of carvacrol and thymol in a 1:1 ratio (60, 100 and 200 mg/kg) to broilers, observing an increased antioxidant enzyme activity (superoxide dismutase and glutathione peroxidase) and a retarded lipid oxidation underlined by a decreased level of MDA. In parallel, they also observed an improvement in zootechnical performance (average daily gain (ADG) and feed efficiency) at the highest dose, as well as enhanced digestive enzyme activities, and improved immune response of broilers. The use of the same mixture was also reported in Clostridium perfringens-challenged broiler chickens, where dietary supplementation with 60, 120 and 240 mg/kg of a carvacrol–thymol blend alleviated intestinal lesions, improved intestinal histomorphology, decreased the inflammatory response and enhanced the specific immune response(Reference Du, Wang and Gan133). Mohammadi et al. (Reference Mohammadi, Hosseindoust and Kim134) found that using a microencapsulated blend of organic acids, thymol (4·25, 8·5 or 12·75 mg/kg) and vanillin resulted in increased BW and decreased FCR of broiler chickens. Antimicrobial and protective effects of thymol were also reported(Reference Hoffman-Pennesi and Wu135). Broilers fed thymol (200, 400 or 800 mg/kg) had increased antioxidant capacity in serum without effects on performance. The addition of thymol in specific pathogen-free chicks challenged with Salmonella enteritidis offered protection, preventing further mortality. Some studies also reported significant results in pigs. Janczyk et al. (Reference Janczyk, Trevisi and Souffrant136) showed slight effects of dietary thymol (10 mg/kg) on pig jejunal microbial populations, with a shift in the bacterial community composition in thymol-treated pigs. Similar results were reported using different concentrations/combinations of thymol and benzoic acid (1:1, 1:2 and 2:1 ratios), with a greater prevalence of Lactobacillus spp. and Bacillus spp. populations, and a reduction of E. coli in caecal digesta. Improved growth performance, nutrient digestion and absorption, and reduced diarrhoea, were also reported(Reference Diao, Zheng and Yu137). Anderson et al. (Reference Anderson, Krueger and Genovese138) found that the level of Campylobacter did not differ among control and treated groups, probably due to the fact that thymol was not encapsulated (Table 1). They concluded that encapsulation or other protective technologies would be needed to enhance thymol efficacy against Campylobacter in growing swine. Feeding thymol (50 mg/kg) may contribute in activating several pathways related to the proliferation of gastric mucosa and control of digestion. In particular, the most up-regulated genes were peptide transporter 1 (SLC15A1), somatostatin (only in pyloric), pepsinogen C (only in pyloric), pancreatic polypeptide, somatostatin receptors (SSTR1 and SSTR2) and calpain 9 (a calpain specific for the gastrointestinal tract)(Reference Colombo, Priori and Gandolfi139). Michiels et al. (Reference Michiels, Missotten and Hoorick140) demonstrated how carvacrol or thymol can improve gut health in pigs fed with 500 or 2000 mg/kg of either carvacrol or thymol: they reported a higher villus:crypt ratio in the distal small intestine and a reduction of intra-epithelial lymphocytes. They also demonstrated that the bitter taste of thymol might reduce voluntary feed intake of swine, so a lower concentration (125 mg/kg) was suggested(Reference Michiels, Missotten and Ovyn141). Cho & Kim(Reference Cho and Kim142) reported positive effects on weaned piglets, like increased BW and average daily feed intake (ADFI), a greater gain:feed ratio and a better faecal score, by using a blend of thymol (8·5 mg/kg), vanillin and organic acids microencapsulated in a lipid matrix. More recently, the same research group confirmed these results, testing the blend in weaning and growing pigs at different doses (Table 1). In particular, concerning weaning pigs, they found increased ADG, ADFI, BW and Lactobacillus count at the highest dose. For growing pigs higher ADG, ADFI and gain:feed ratio were recorded for all the doses, while increased BW was reported for the highest dose. All the pigs showed improved meat quality(Reference Oh, Kim and Song143). They also tested this blend in combination with microencapsulated zinc oxide, reporting a reduction in E. coli count(Reference Cho, Liu and Yun144). Using the same blend, an improvement of the integrity of the gut mucosa in weaning piglets was found by Grilli et al. (Reference Grilli, Tugnoli and Passey35): higher transepithelial electrical resistance, reduced intermittent short-circuit current and dextran flux were measured in ex vivo Ussing chamber analysis on duodenal and jejunal tissues. Moreover, a decrease in local and systemic inflammatory pressure was also measured. Supplementing this mixture to sows’ diet allowed the observation of beneficial effects on the performance of sows and piglets in terms of BW and ADFI(Reference Balasubramanian, Park and Kim145). Grilli et al. (Reference Grilli, Foresti and Tugnoli146) also found that the synergistic use of microencapsulated thymol and carvacrol (starting from 120 mg/kg of thymol + carvacrol), in combination with an organic acid, decreased Salmonella typhimurium prevalence in the intestine and the presence of lymph nodes in piglets in a controlled challenge environment. Furthermore, the same blend reduced Salmonella typhimurium and Salmonella enterica in faecal samples of animals fed during production conditions. An increasingly attractive property of thymol and carvacrol is represented by the role played in the gut chemosensory system. Thymol, as well as carvacrol and eugenol, is known to activate the receptor TRPV3. Activation of TRPV3 by carvacrol or thymol in the palate, tongue and nasal epithelium leads to warmth perception in the brain whereas, at the intestinal level, it seems to trigger release of serotonin (5-HT)(Reference Braun, Voland and Kunz147), which controls gut motility and chloride secretion by enterocytes(Reference Nezami and Srinivasan148). In fact, Boudry & Perrier(Reference Boudry and Perrier126), using the ex vivo Ussing chamber technique, showed that thymol induces Cl– and HCO3 – secretion of epithelial intestinal cells via a nervous pathway. Thymol and carvacrol also seem to activate TRPA1, which plays a role in pain sensation(Reference Holzer40), and this could be the reason why, for example, these phenols sometimes affect feed intake when fed at high doses and are not encapsulated(Reference Michiels, Missotten and Ovyn141).
Capsaicin
Capsaicin (trans-8-methyl-N-vanillyl-6-nonenamide) is a monoterpene alkaloid, considered the major pungent component in the genus Capsicum. It is characterised by antioxidant, hypotensive and antimicrobial effects(Reference Anand and Bley149–Reference Rivera, Hassimotto and Bueris151). The antimicrobial targets of capsaicin have not been identified yet(Reference Anand and Bley149) but it can reduce cell-invasiveness of Streptococcus (cell-invasive group A) in vitro (Reference Marini, Magi and Mingoia152). Tellez et al. (Reference Tellez, Jaeger and Dean153) administrated 18 mg/kg of capsaicin in broilers challenged with Salmonella enteritidis, finding that thickness of the caecal lamina propria and epithelial cells was significantly higher, suggesting the possibility that capsaicin induces alterations to the host susceptibility to Salmonella enteritidis invasion. These findings were confirmed by further studies by the same research group, with two different doses of capsaicin (5–20 mg/kg)(Reference McElroy, Manning and Jaeger154). Conflicting results were shown from a study in which the administration of 10 mg/kg of capsaicin increased liver/spleen invasion and caecal colonisation with Salmonella enteritidis in challenged chicks. Conversely, addition of 20 mg/kg of capsaicin resulted in reduced liver, spleen and caecal count of Salmonella (Reference Orndorff, Novak and Pierson155). At the same time, growth of a Streptococcus in the presence of sublethal capsaicin concentrations induced an increase in biofilm production(Reference Marini, Magi and Mingoia152). In recent years, the role of capsaicin in pain reduction and control has become evident, thanks to the discovery of the link between this alkaloid and TRPV channels, in particular the receptor TRPV1(Reference Moran and Szallasi156). A single administration of capsaicin intensifies the feeling of pain, but if the alkaloid is administered several times, the vanilloid receptor is desensitised and the conduction of the pain stimuli is blocked, which results in pain alleviation(Reference Fusco and Giacovazzo157).
Artemisinin
Artemisinin, a sesquiterpene lactone, is an active compound present in Artemisia annua. Sesquiterpenes were shown to be potent inhibitors of neutrophil migration, lysosomal rupture, enzymic activity and prostaglandin synthesis(Reference Hall, Starnes and Lee158). Artemisinin is well known in Malaria’s disease treatment(159) and has anti-inflammatory, antiviral, antibacterial, antiparasitic and insecticidal properties(Reference Zyad, Tilaoui and Jaafari160). Recent studies demonstrated interesting anticoccidial effects of artemisinin in the treatment of Eimeria spp. infection in poultry, which represent one of the main risk factors for necrotic enteritis onset. Pop et al. (Reference Pop, Györke and Tăbăran161) infected 1-d-old chickens with Eimeria acervulina, Eimeria tenella and Eimeria maxima, and treated them with different concentrations of artemisinin (5, 50 and 500 mg/kg) for 14 d. They found that artemisinin did not have a positive effect on the intestinal lesions produced by Eimeria acervulina, but 5 and 50 mg/kg decreased Eimeria tenella lesion scores. Moreover, they observed a reduction in the sporulation rate of Eimeria tenella at all the given concentrations, but the lowest number of sporulated oocysts was recorded for the group supplemented with 5 mg/kg of artemisinin. BW and FCR were higher in the groups treated with 5 and 50 mg/kg. Even though oocysts shedding was not reduced, Allen et al. (Reference Allen, Danforth and Augustine79) obtained a significant drop in oocysts shedded per g of faeces (OPG) with the administration of 17 mg/kg of artemisinin. Another study demonstrated that administering artemisinin (10–17 mg/kg) in feed significantly reduced OPG, sporulation and lesion scores(Reference del Cacho, Gallego and Francesch162). The mode of action of artemisinin implies the production of free radicals and ROS due to the cleavage of its endoperoxide bridge and induction of oxidative stress, resulting in the inhibition of sarco/endoplasmic reticulum calcium ATPase of coccidia(Reference del Cacho, Gallego and Francesch162) and consequently in their death(Reference Mo, Ma and Zhao163). This phytochemical seems to have an adverse effect on weight gain and also on FCR when fed at higher concentrations (500 mg/kg)(Reference Pop, Györke and Tăbăran161), whereas concentrations lower than 150 mg/kg are considered safe(Reference Shahbazfar, Mardjanmehr and Arab164).
Aldehydes
Cinnamaldehyde and vanillin are the most reported aldehydes in the literature for their anti-inflammatory and antioxidant potential. The latter seems to be related to their phenolic ring which inhibits pro-inflammatory mediators and could up-/down-regulate transcriptional factors, like NF-κB, in inflammatory and antioxidant pathways(Reference Ambriz-Pérez, Leyva-López and Gutierrez-Grijalva165).
Cinnamaldehyde
Cinnamaldehyde is a phenolic aldehyde with antibacterial, antiparasitic, anticoccidial, antioxidant and anti-inflammatory activities(Reference Shen, Jia and Honma166). Cinnamaldehyde inhibits NF-κB, NO synthase and cyclo-oxygenase-2 activity(Reference Boudry and Perrier126), it is a broad spectrum antimicrobial agent(Reference Burt20) and interferes with bacterial QS communication processes(Reference Ta and Arnason62,Reference Niu, Afre and Gilbert167,Reference Gill and Holley168) and FtsZ protein synthesis(Reference Domadia, Swarup and Bhunia169). Moreover, other antimicrobial targets of cinnamaldehyde are the perturbation of the bacterial membrane and the inhibition of bacterial ATPase(Reference Nazzaro, Fratianni and De Martino18). Supplementation with trans-cinnamaldehyde (5000 or 7500 mg/kg) consistently decreased Salmonella enteritidis count in challenged chickens, with a significant reduction of pathogen populations in caecal samples(Reference Kollanoor-Johny, Mattson and Baskaran120). This antimicrobial activity was also reported against Brachyspira intermedia in young pullets(Reference Verlinden, Pasmans and Mahu116). As mentioned in the paragraph about carvacrol, Jamroz et al. (Reference Jamroz, Orda and Kamel129) found improved ileal digestibility of amino acids and reduced E. coli and Clostridium perfringens counts in poultry fed with a blend of cinnamaldehyde, carvacrol and capsaicin (Table 1). The same mixture enhanced BW and reduced gut lesions in broilers orally challenged with Eimeria acervulina (Reference Lillehoj, Kim and Bravo128). Moreover, supplementation with cinnamaldehyde (150 mg/kg) significantly decreased gross lesion scores of the intestinal mucosa in Eimeria acervulina-infected chickens(Reference Orengo, Buendía and Ruiz-Ibáñez170). Lee et al. (Reference Lee, Lillehoj and Jang171) demonstrated that feeding cinnamaldehyde (14·4 or 125 mg/kg) increased the levels of intestinal mRNA encoding IL-1β, IL-6, IL-15 and interferon-γ, reduced BW loss induced by Eimeria acervulina and Eimeria maxima, reduced Eimeria acervulina oocyst shedding and increased the Eimeria tenella-stimulated antibody. Furthermore, dietary inclusion of 45 mg/kg of cinnamaldehyde in pigs challenged with Ascaris suum increased plasma concentrations of IgG and, to a lesser extent, IgA. Moreover, acquisition of Ascaris suum-specific antibody responses was enhanced. Colonisation of Ascaris suum in the intestine was not significantly affected by dietary treatments(Reference Williams, Hansen and Krych172). Anticoccidial and antiparasitic activities are probably related to the immunomodulatory ability of cinnamaldehyde. Moreover, through an ex vivo analysis, Boudry & Perrier(Reference Boudry and Perrier126) found how cinnamaldehyde induced HCO3 – secretion by intestinal epithelial cells. Furthermore, this molecule is known to activate the TRPA1 channel that stimulates secretion of Cl– and HCO3 – in colonic epithelial cells(Reference Kuwahara173).
Vanillin
Vanillin is a phenolic aldehyde widely used as a flavour compound. The antimicrobial action of vanillin targets the bacterial membrane, inhibits the bacterial respiration pathway and inhibits QS communication(Reference Nazzaro, Fratianni and De Martino18,Reference Ponnusamy, Paul and Kweon174) . Vanillin is not only known as antimicrobial, but also for its positive action on the intestinal mucosa. In fact, vanillin also has antioxidant potential, inhibits protein oxidation and lipid peroxidation, increases antioxidant enzyme activities and scavenges free radicals(Reference Tai, Sawano and Yazama175). It also protects DNA and the mitochondrial membrane against oxidative stress in vitro (Reference Kumar, Priyadarsini and Sainis176). Moreover, vanillin activates the TRPV3 channel, like thymol and carvacrol(Reference Xu, Delling and Jun41), whose activation, at the intestinal level, seems to trigger the release of serotonin which controls gut motility and chloride secretion by enterocytes, as mentioned before(Reference Braun, Voland and Kunz147). As stated in the thymol paragraph, many studies have reported positive effects (performance, reduction in pathogens count, maturation of the intestinal mucosa, decrease of local and systemic inflammatory pressure) in poultry and pigs fed a blend of vanillin, thymol and organic acids microencapsulated in a lipid matrix(Reference Grilli, Tugnoli and Passey35,Reference Cho and Kim142–Reference Balasubramanian, Park and Kim145) (Table 1).
Overall conclusions
Gastrointestinal disorders represent one of the major contributors to economic losses in animal production(Reference Paiva and McElroy177,Reference Sjölund, Zoric and Wallgren178) . It is clear that dietary interventions to control or prevent intestinal pathologies should be not only antimicrobial, but also anti-inflammatory. Botanicals and their bioactive compounds gained a growing interest throughout the last 20 years since they show many biological activities of great relevance for the livestock industry. For this reason, in recent years the number of published papers regarding the use of botanicals has increased dramatically. A wide variety of extracts has been used in poultry and pigs as feed additives(Reference Li, Ru and Liu16,Reference Lillehoj, Liu and Calsamiglia53) , and botanicals from different sources are taken into consideration. Available data on the effects of botanicals make it difficult to associate specific effects to specific bioactive compounds because of the high variability in EO/OR composition. For what concerns practical application and the development of feed additives containing these bioactive compounds, there is an urgent need of standardisation. For this reason, NIC that are produced synthetically but are chemically identical to their natural counterpart, are a viable solution in animal nutrition. As the percentage of biochemical actives in a given EO/OR may vary substantially, along with the level of impurities, the inclusion of a defined bioactive compound or a defined blend avoids variability. Therefore, selecting individual substances with a specific biological activity allows achievement of a synergistic effect. Despite that, the present review highlighted how the inclusions of bioactives vary widely in relation to the target to achieve or if the compounds were encapsulated or not, as well as in relation to their chemical structures. In fact, NIC belong to different chemical classes, therefore they exert different mechanisms of action providing different effects. For example, polyphenols showed a predominant antioxidant activity due to their aromatic ring structures, whereas organosulfur compounds seem to exert mainly antibacterial and antiprotozoal activities, even if the molecular mechanism of action is still not fully elucidated. Aldehydes are also mainly antimicrobial due to their ability to interfere with bacterial QS communication, as well as the capacity to inhibit bacterial cell division proteins. Terpenes, the most studied group of natural bioactive compounds, displayed antimicrobial, antioxidant, anti-inflammatory and immunomodulating powers, consequently leading to an improved intestinal barrier integrity.
The studies here reviewed often refer to a blend of bioactive compounds, instead of the single ones, so it is difficult to associate a precise mechanism of action to a precise molecule in vivo. Despite that, looking at the mode of action in vitro, it is possible to understand the potential translated effects in vivo. Furthermore, it is important to look at a blend of components, rather than a single bioactive, in order to avoid induction of resistance in bacteria and to allow botanicals to work in synergy.
NIC represent a promising non-antibiotic tool, or an adjuvant to pharmaceutical treatments, allowing the creation of synergistic blends able to exert many effects translatable as better intestinal health and general health status in livestock, thereby driving better growth performance.
Acknowledgements
The present review received no specific grant from any funding agency, commercial or not-for-profit sectors.
B. R., A. T., A. P. and E. G. contributed equally to the conception and design of the review, conducted the literature review and drafted the manuscript.
A. P. serves on the Board of Directors of Vetagro. E. G. serves as an advisor of Vetagro.