Conservation agricultural (CA) practices—defined by integration of reduced tillage, residue retention, and diverse crop rotations—are frequently promoted for their potential to improve soils, but management of weeds is often a major constraint to their adoption (Hobbs Reference Hobbs2007; Kumar et al. Reference Kumar, Singh, Chhokar, Malik, Brainard and Ladha2013; Reicosky Reference Reicosky2015). Strip tillage (ST) has garnered interest as a potentially useful component of CA cropping systems involving a wide range of crops, including sugar beets (Beta vulgaris L.) (Overstreet Reference Overstreet2009), maize (Zea mays L.)(Al-Kaisi et al. Reference Al-Kaisi, Archontoulis, Kwaw-Mensah and Miguez2015), wheat (Triticum aestivum L.)(Hossain et al. Reference Hossain, Gathala, Tiwari and Hossain2014), and vegetables (Brainard et al. Reference Brainard, Peachey, Haramoto, Luna and Rangarajan2013; Luna and Staben Reference Luna and Staben2002). In ST, crops are planted directly into tilled strips, while the soil between these strips is left undisturbed. This form of reduced tillage has the potential to reduce erosion, maintain or improve soil quality (Lemke et al. Reference Lemke, VandenBygaart, Campbell, Lafond, McConkey and Grant2012), and reduce input costs through lower fuel and labor use (Luna and Staben Reference Luna and Staben2002) compared with full-width tillage (FWT). ST also provides benefits compared with no-till (NT) by facilitating seedbed preparation for sensitive crops, like vegetables, and incorporation of soil amendments in the crop root zone. However, weed management in ST systems is often more challenging then under FWT, since primary tillage that disrupts weeds is reduced, and residues left on the soil surface can interfere with herbicide or cultivation efficacy (Banks and Robinson Reference Banks and Robinson1986; Brainard et al. Reference Brainard, Peachey, Haramoto, Luna and Rangarajan2013). These problems are particularly acute in cropping systems for which few herbicide options are available (e.g., vegetables), and for which some form of physical weed management is often required.
This study was motivated in part by the lack of available information on spatial and temporal variation in weed emergence response to tillage and cover-cropping practices in ST-based CA systems. Although many studies have evaluated the impact of tillage and cover cropping on weed emergence, very few have done so on a scale necessary for understanding the unique behavior of weeds in spatially heterogeneous environments characteristic of ST systems. Understanding how weed emergence differs in distinct zones in ST systems should be helpful for identifying complementary management practices that improve the performance of such systems. These are likely to include optimization of weed management practices that target weeds differently in distinct zones within the field, such as zonal cover cropping, combinations of in-row (IR) and high-residue cultivation tools for the between-row (BR) zone, or banded herbicide applications (Brainard et al. Reference Brainard, Peachey, Haramoto, Luna and Rangarajan2013; Lowry Reference Lowry2015). In addition, improved understanding of weed emergence patterns in ST may suggest adjustments in the location and timing of zone-specific management practices that influence weed emergence, including irrigation (e.g., subsurface drip) and fertilization (e.g., banded, slow-release fertilizers).
Studies evaluating the impacts of tillage on weed emergence often do not control for changes in the distribution of seeds in the soil profile resulting from tillage and hence provide limited information on the direct effects of tillage-induced changes in edaphic conditions. For example, emergence of Powell amaranth and related species, including common waterhemp (Amaranthus rudis Sauer) and redroot pigweed (Amaranthus retroflexus L.), is sometimes much higher in NT compared with tilled soils (Leon and Owen Reference Leon and Owen2006; Oryokot et al. Reference Oryokot, Murphy and Swanton1997; Refsell and Hartzler Reference Refsell and Hartzler2009). However, what is unknown is the extent to which these differences in emergence were due to greater concentration of seeds near the soil surface under NT or differences in recruitment due to changes in edaphic conditions. In addition to redistributing seeds, tillage can impact weed emergence through a variety of mechanisms, including changes in germination stimuli and seed dormancy status. Weed seed germination and hence emergence is typically stimulated by tillage, which aerates the soil, releases a flush of inorganic nutrients, creates good seed–soil contact, exposes seeds to light, and alters temperature regimes (Mohler Reference Mohler2001). Tillage effects on predators and decay agents of seeds can also be profound and further complicate predictions of emergence. For example, reduced tillage systems may provide improved habitats for ground-dwelling seed predators (Shearin et al. Reference Shearin, Reberg-Horton and Gallandt2007) and hence reduce emergence of certain species through increases in rates of predation.
Weed seeds in ST fields face very different environments depending on whether they are in the tilled IR zone or the untilled BR zone, and cover crop residues add to this spatial complexity. Under ST, residues are incorporated in the tilled IR zone, but left on the surface as a mulch layer in the untilled BR zone. Emergence is typically decreased by incorporated residues through physical, chemical, and biological means often termed “residue-mediated effects.” For example, incorporating oats reduced weed density by over 90% compared with a tilled soil without cover crop residues (Radicetti et al. Reference Radicetti, Mancinelli and Campiglia2013). The magnitude of these effects can be variable—incorporated oat residue decreased hairy galinsoga (Galinsoga quadriradiata Cav.) emergence by 50% in one year, but had no effect in another (Kumar et al. Reference Kumar, Brainard and Bellinder2009). Surface cover crop residues, such as those located in the BR zone in ST, can also have large impacts on weed emergence (Bernstein et al. Reference Bernstein, Stoltenberg, Posner and Hedtcke2014; Davis Reference Davis2010; Mirsky et al. Reference Mirsky, Curran, Mortensen, Ryan and Shumway2011), often larger than those of incorporated residues when these are compared directly (Kruidhof et al. Reference Kruidhof, Bastiaans and Kropff2009). These effects may be driven by changes in abiotic factors, including light penetration, physical obstruction, soil temperature, soil moisture, and soil chemical properties (Teasdale and Mohler Reference Teasdale and Mohler1993, Reference Teasdale and Mohler2000). In addition, surface residues may also provide a habitat for agents of decay or predation that contribute to pre-emergence mortality; interactions between light, temperature, and particularly soil moisture may be important regulators of these organisms (e.g., Green Reference Green2010; Quinn Reference Quinn2015; Shearin et al. Reference Shearin, Reberg-Horton and Gallandt2007).
Tillage and cover crop effects on weed emergence and crop yields may be particularly dependent on rainfall patterns. For example, higher soil moisture has been observed in both the IR (Haramoto and Brainard Reference Haramoto and Brainard2012) and BR zones (Dahiya et al. Reference Dahiya, Ingwersen and Streck2007) of ST fields relative to similar locations in fields with FWT. This may favor germination and emergence in ST relative to FWT in dry years. Surface cover crop mulches may also increase soil moisture and enhance this effect; emergence of lettuce (Lactuca sativa L.) seedlings was greater under a cereal rye (Secale cereale L.) mulch than in bare soil in a dry year but lower in normal and wet years (Kruidhof et al. Reference Kruidhof, Bastiaans and Kropff2009). Based on a meta-analysis of 610 studies comparing NT-based CA practices to FWT, Pittelkow et al. (Reference Pittelkow, Liang, Linquist, van Groenigen, Lee, Lundy, van Gestel, Six, Venterea and van Kessel2015) concluded that crop yield benefits generally required residue and were dependent on rainfall, with the greatest benefits occurring in dry climates. Although these findings in part reflect improved moisture retention in CA systems, differences in weed emergence response to CA may also have played an important role in some cases.
Powell amaranth and its close relatives (e.g., redroot pigweed) are problematic weeds in multiple CA cropping systems throughout the world and are noted for their prolific seed production (Brainard and Bellinder Reference Brainard and Bellinder2004; McLachlan et al. Reference McLachlan, Murphy, Tollenaar, Weise and Swanton1995). Worldwide, Powell amaranth has developed resistance to Group 2, 5, and 7 herbicides (Heap Reference Heap2016). As such, improved understanding of its response to management practices may help improve the productivity of systems in which it occurs. Previous studies have established the importance of soil temperature and moisture in regulating emergence of Amaranthus species. For example, common waterhemp emergence was delayed in cooler NT soils (Leon and Owen Reference Leon and Owen2006); Amaranthus seedlings emerged faster in NT than in tilled soils in a dry year, which the authors attribute to increased soil moisture (Oryokot et al. Reference Oryokot, Murphy and Swanton1997). Moisture conditions may also influence Powell amaranth through indirect effects on soil nutrient availability or the presence of decay agents. For example, amaranth seedlings are sensitive to damping-off pathogens, including Pythium species (Sealy et al. Reference Sealy, Kenerley and McWilliams1990), which are known to proliferate under moist conditions. Powell amaranth germination is also known to be sensitive to inorganic nitrogen concentrations (Brainard et al. Reference Brainard, DiTommaso and Mohler2006), which are strongly influenced by soil moisture and will typically increase in response to tillage events.
Weed emergence is the end result of a number of processes, including the loss of seed dormancy, germination, and pre-emergence seed and seedling mortality. The main goal of this experiment was to better understand whether processes occurring after dormancy release vary between different zones in ST relative to FWT, and thus how the potential for emerged weeds differs in these zones, both with and without cover crop residues. The specific objectives of this experiment were twofold: (1) characterize the effects of tillage and cover crop residue on IR and BR emergence of Powell amaranth and (2) evaluate how rainfall may mediate these effects. We hypothesized that emergence of Powell amaranth would be reduced under ST and in the presence of oat residue, and that these effects would be most pronounced in the untilled BR zone of strip till. In addition, we hypothesized that both tillage and cover crop effects would be mediated in part by rainfall. In particular, we hypothesized that low-rainfall conditions would enhance emergence in ST where a cover crop is present due to moisture conservation, while high-rainfall conditions would suppress emergence.
Materials and Methods
Plot Establishment
This experiment was conducted in three different sections of a 1.6 ha field in 2010, 2011, and 2012 at the Kellogg Biological Station in Hickory Corners, MI (42.4058°N, 85.3845°W). Soil type at this site consists primarily of an Oshtemo coarse-loamy soil series (mixed, mesic Typic Hapludalf) with pockets of Kalamazoo fine loamy soil. Temperature and precipitation data during these 3 yr from a nearby weather station are summarized in Table 1 and Figure 1; this weather station is located approximately 700 m from the experimental site. Prior to use in this experiment, the field was in NT soybeans or NT chemical fallow (prior to the section used in 2012). We examined four treatments—a fully factorial combination of two tillage levels (ST and FWT) and two cover crop levels (oats or none). These treatments were assigned to main plots that were 3.1 m wide by 4.3 m long.
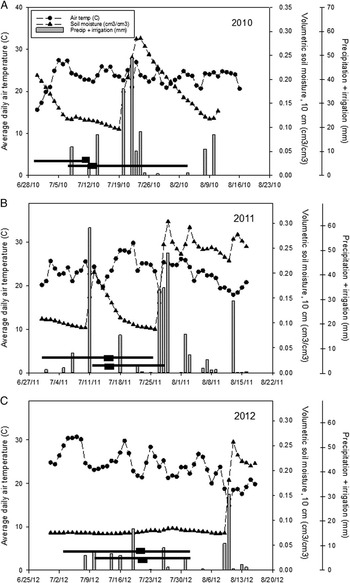
Figure 1 Air temperature, volumetric soil moisture, and precipitation + irrigation in 2010, 2011, and 2012. Soil moisture levels reflect only precipitation (not supplemental irrigation) and are provided only to illustrate relative differences in baseline precipitation and soil moisture conditions between years. The periods during which emerged weeds were counted are denoted by horizontal black lines; periods of peak emergence are shown with a black rectangle.
Table 1 Monthly average temperature and monthly total precipitation (plus overhead irrigation applied to the entire experiment) for April to August in 2010, 2011, and 2012 at the Kellogg Biological Station in Hickory Corners, MI.

a Ten year average monthly temperature and average total monthly precipitation from 2002 to 2011.
b Irrigation applied in June 2012 was applied prior to sowing of experimental seeds.
c Rainfall in July 2011 was scattered, with 59 mm falling before July 6 and 117 mm falling within 3 d (July 27–29). Overhead irrigation was added to the entire experiment on July 15 and 19 when needed (by visual estimation of a nearby cabbage crop).
Field operations are summarized in Table 2. The oat cover crop was sown at 93 kg ha−1 with an NT drill (John Deere model 750, John Deere Equipment Company, Moline, IL). Glyphosate was applied prior to oat planting in 2011 and 2012, but not in 2010, as few emerged weeds were observed in this year. All plots were fertilized in mid-May based on soil nutrient analysis, and typical fertilizer rates for a small grain (2010: 19-19-19 provided 43 kg of N, 19 kg of P, and 35 kg of K, respectively, ha−1; 2011: 47 kg N ha−1 with urea; 2012: 10 kg N ha−1 with urea). Weeds were not controlled in the cover crop plots during oat growth; glyphosate application and/or hand weeding was used to control weeds in all plots without oats. Cover crop and weed biomass were sampled prior to burndown glyphosate application in mid-June by clipping all biomass at the soil surface from two 0.25 m2 quadrats in each plot. Oats were flail mowed 7 to 12 d after glyphosate application. Due to poor oat growth in 2012, oat residue was raked from areas adjacent to the plots and spread into plot areas to increase biomass to comparable levels to that grown in 2010 and 2011.
Table 2 Timeline for field operations in 2010, 2011, and 2012.

a DAT, days after tillage.
Additional fertilizer was spread by hand prior to tillage in all plots, with rates based on soil test recommendations for a typical vegetable crop such as cabbage (Warncke et al. Reference Warncke, Dahl and Zandstra2004). In 2010, 81 kg N ha−1, 100 kg P ha−1, and 69 kg K ha−1 were applied as a combination of monoammonium phosphate, triple superphosphate, potash, and urea. In 2011 and 2012, 78 kg N ha−1, 28 kg P ha−1, and 113 kg K ha−1 were applied as 19-19-19, potash, and urea.
Tillage occurred immediately after fertilization. For ST plots, tillage was accomplished with one pass of a two-row strip tiller (Hiniker model 6000, Hiniker, Mankato, MN) equipped with cutting disks, a shank, berming disks, and a rolling basket. In FWT plots, one pass with a chisel plow was used for primary tillage followed by two passes with a field cultivator for secondary tillage.
A small amount of supplemental irrigation was applied in each year over the entire experiment using an overhead system in order to keep soil moisture conditions above the permanent wilting point. A total of 20 mm was applied in 2010, 18 mm in 2011, and 52 mm in 2012 (Table 1). In 2012, 38 mm was applied in June prior to tillage and the onset of the emergence trial; the remainder was applied to the entire experiment during the course of the emergence periods.
Weed Emergence Evaluation
Seeds of Powell amaranth were collected from adjacent fields in the fall preceding each experiment, separated from chaff using a rub board and seed cleaner, and stratified under moist conditions at 4 C for 4 mo to mimic overwintering conditions in the field. Prior to planting the following summer, seeds were soaked overnight in 2 mM gibberellic acid (Buhler and Hoffman Reference Buhler and Hoffman1999) and dried to induce greater germination. Following this treatment, Powell amaranth germination rates in petri dishes at 25 C with a light/dark cycle of 14/10 h were 32 and 49% in 2011 and 2012, respectively.
Weed seeds were sown either immediately after tillage (0 d after tillage [DAT]), or 7 to 13 DAT to simulate those that may emerge at the time when a crop would typically be planted relative to tillage used to incorporate a cover crop. Seeds were sown into 0.09 m2 subplot quadrats (0.3 m on each side). In the BR zone of ST, the untilled zone, seeds were sprinkled onto the soil surface and lightly packed. In all tilled zones, seeds were mixed in with the top 5 mm of soil and lightly packed. Separate quadrats were located in each of the IR and BR zones in ST, while only one quadrat was located in each FWT plot (separate quadrats were used for each subplot factor when assessed; see Water Manipulation Subplots section below). All BR quadrats were located in non–tire track areas. The number of Powell amaranth seeds sown in each quadrat was 500 in 2010, 700 in 2011, and 600 in 2012. No attempt was made to separate emergence from the ambient seedbank from total emergence. However, observations of Powell amaranth emergence in adjacent areas without supplemental seeds suggested that emergence from the ambient seedbank represented less than 1% of total emergence in the research plots.
We sowed seeds after the tillage events, rather than simulating seed rain prior to tillage or the previous fall. This method of seed placement was chosen to better control seed depth and to better track the fate of experimentally sown seeds. Seeds sown prior to tillage would have been redistributed differently by depth in ST vs. FWT, thereby complicating interpretation. This approach was chosen since our objective was to understand the impact of tillage-induced differences in edaphic factors—not burial depth—on weed emergence. However, it should be noted that seeds stored overwinter and sown following tillage in the spring may have behaved somewhat differently than those overwintering in the field, due to changes in dormancy status or soil–seed contact that may have occurred under field conditions.
Water Manipulation Subplots
In 2011 and 2012, subplot treatments were included to simulate different rainfall levels. These subplot treatments were applied to separate quadrats (also 0.09 m2, 0.3 m on each side) within the main plots and were located at least 0.5 m from each other. One set of quadrats was exposed to ambient moisture conditions (“ambient”)—including ambient precipitation and the overhead irrigation applied to the entire site. Additional irrigation water was supplied to another set of quadrats (“+water”) with a backpack sprayer; low pressure (68 kPa) was used to avoid washing seeds out of the quadrats. These received the same precipitation and irrigation as ambient treatments, along with an additional 15 mm of water applied in three equal 5 mm applications over 6 d. This amount of additional water was chosen to maintain moist soil at the onset of the experiment in 2011 and was maintained for consistency in 2012. Finally, precipitation was excluded from an additional set of quadrats (“−water”) using exclosures (0.6 by 0.9 m) constructed from plastic sheeting stretched over a flexible plastic frame, with open sides to minimize temperature shifts. The bottom of the frame was in contact with the soil surface, and extended approximately 1.25 cm above the soil surface to avoid lateral movement of surface water into quadrats during rainfall events. Exclosures were placed in the field 1 h before rainfall was expected to begin and removed less than 1 h after rainfall termination to minimize non–moisture related effects (e.g., light, temperature changes). Because precipitation was much higher in 2011 compared with 2012 (Figure 1), soil moisture levels in ambient and +water treatments were likely also higher in 2011 compared with 2012, while −water treatments were similar across the 2 yr.
Data Collection
Emerged seedlings of Powell amaranth were counted and pulled daily until fewer than two seedlings were emerging per quadrat per day for at least 3 d. Evaluation time periods were as follows: July 5 to July 14, 2010 (early) and July 14 to August 6, 2010 (late); July 4 to July 28, 2011 (early) and July 18 to August 16, 2011 (late); and July 6 to August 3, 2012 (early) and July 16 to August 3, 2012 (late). Emergence was summed over the entire period. Weeds of other species were removed by hand as they emerged.
Statistical Analysis
Emergence data were square-root transformed as necessary prior to analysis to improve normality. Data were grouped according to their variances when variances were heterogeneous as determined by a Levene’s test; the best model was selected based on Akaike’s information criterion. The percentage of emerged seedlings of the total seeds sown was the dependent variable. For both emergence timings, this percentage was subjected to an analysis of variance using SAS PROC MIXED (version 9.2, SAS Institute, Cary, NC). Block (replicate) was considered a random factor. In 2010, when the subplot factors were not tested, a two-way ANOVA was used with tillage, cover crop, and the interaction tested. In 2011 and 2012, tillage, cover crop, and the interaction term were main plot factors, while the subplot treatment (ambient, +water, and −water) was the subplot factor. Emergence was analyzed separately by zone (IR and BR) and by year, as initial testing indicated significant zone by treatment and year by treatment interactions. Single degree of freedom contrasts and slicing were used to separate significant interactions where appropriate; α=0.05 was selected as the significance level.
Results and Discussion
Weather Conditions
During the period of cover crop growth (mid-April to late June), 2010 was relatively warm and wet compared with the 10 yr average (Table 1). April 2011 was 1.8 C cooler than the 10 yr average and also wetter. Spring 2012 was warmer and much drier than average, which negatively impacted cover crop growth, resulting in lower oats biomass accumulated in 2012 (Table 3).
Table 3 Average cover crop and weed biomass prior to termination (standard error in parentheses).Footnote a

a Biomass was collected from two 0.25 m2 quadrats per plot.
b Includes supplemental residue raked into plot areas.
Average daily temperature during the duration of the emergence periods is shown in Figure 1, as is daily precipitation (plus whole-experiment irrigation when applied) and volumetric soil moisture measured at a 10 cm depth under sod at the nearby weather station. As such, soil moisture levels presented in Figure 1 reflect only precipitation (not supplemental irrigation) and are provided only to illustrate relative differences in baseline precipitation and soil moisture conditions between years.
In 2010 ambient soil moisture was initially high but decreased throughout the emergence period of early planted seeds (represented by a horizontal black line on Figure 1A), remaining relatively low during the period of peak emergence represented by the black rectangle (Figure 1A). In 2011 ambient soil moisture was initially low during the emergence period of early planted seeds, but increased due to a large precipitation event on July 12, prior to peak emergence during this period (Figure 1B). Finally, soil moisture remained low throughout both emergence periods in 2012 (Figure 1C). During the emergence of late-planted seeds, soil moisture was initially low in 2010, including during the time of peak emergence, and increased after rainfall events starting on July 21. Ambient soil moisture was initially higher during the emergence of late-planted seeds in 2011 but decreased steadily.
Cover Crop and Weed Biomass
Oats produced approximately 2,800 kg ha−1 in 2010 and 2011 (Table 3). Oat growth was poor in 2012, likely because of low precipitation during May and June 2012 (Table 1), producing on average only 1,900 kg ha−1. With the residue raked into the plot areas, biomass was increased to almost 2,800 kg ha−1. Weed biomass within the oat cover crop was variable and ranged from 108 to 1,084 kg ha−1. Higher weed biomass was observed in 2010, the year in which we did not apply glyphosate prior to cover crop planting. Lower weed biomass was observed in 2011, the year with higher than average rainfall, suggesting that oats are more successful in outcompeting weeds in years with adequate moisture (Ateh and Doll Reference Ateh and Doll1996). Dominant weed species within the cover crop growth period were shepherd’s purse [Capsella bursa-pastoris (L.) Medik.], mouse-ear cress [Arabidopsis thaliana (L.) Heynh.], and common chickweed [Stellaria media (L.) Vill.].
Tillage Effects on Emergence following Early Planting
In-Row (IR). At the early timing (planted 0 DAT), emergence of Powell amaranth was lower in the tilled IR zone of ST compared with FWT in two of three years—by 42 and 23% in 2010 and 2012, respectively (Table 4; Figure 2A). IR emergence did not differ between tillage types in 2011. We did not observe any interactions between tillage and the moisture subplots in this zone (Table 4), so this suppression does not appear to be related to differences in soil moisture. Other factors that may have influenced Powell amaranth emergence include physical differences in the seedbed, temperature, or impacts on nitrogen or fungal pathogens. Others have demonstrated lower soil temperatures (Mochizuki et al. Reference Mochizuki, Rangarajan, Bellinder, Bjorkman and van Es2007) and lower availability of nitrogen (Haramoto and Brainard Reference Haramoto and Brainard2012) in the IR zone of ST, both of which could reduce germination and emergence of Powell amaranth (Brainard et al. Reference Brainard, DiTommaso and Mohler2006).
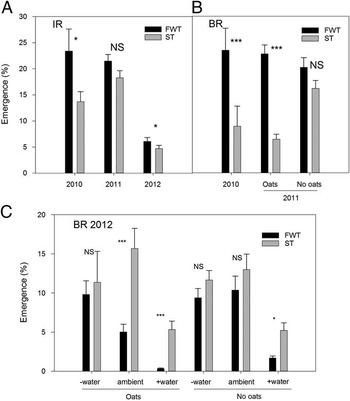
Figure 2 Effects of tillage on emergence of Powell amaranth planted early (sown 0 d after tillage) in-row (IR; A) and between-row (BR; B), and a three-way interaction on BR emergence in 2012 (C). Error bars represent ±1 SE. Within each year or interaction, significance levels for the difference between full-width tillage (FWT) and strip till (ST) are shown. NS, difference is not significant; *, P<0.05; **, P<0.01; ***, P<0.001.
Table 4 Results of a three-way ANOVA for early in-row (IR) and between-row (BR) emergence of Powell amaranth beginning 0 d after tillage.

a Main plot factors were tillage and cover crop, with moisture treatment (ambient, +water, −water) as the subplot factor.
Between-Row (BR). Compared with FWT, emergence of Powell amaranth at this first planting time was reduced in ST-BR by 62% in 2010 and by 72% in 2011 where oat residue was present (Figure 2B). The effects of oats and ST on emergence in 2011 did not depend on moisture (no significant interactions; Table 4), so this suggests that emergence was suppressed in this zone due to another mechanism—perhaps due to physical impedance from the surface oats residue.
In 2012 tillage had very different effects on emergence: ST often increased BR emergence relative to FWT, but these effects depended on both moisture treatments and oats residue. In particular, emergence was greater in ST-BR relative to FWT only in +water subplots and ambient moisture subplots with oats (Figure 2C). This result was contrary to the hypothesis that higher emergence would occur in low-moisture treatments in the ST-BR zone in dry years like 2012 (Table 2; Figure 1C) because of the moisture-conserving effects of ST.
In 2012 Powell amaranth emergence in +water subplots was lower than emergence in ambient and –water subplots for all combinations of tillage and oats residue except ST oats (Figure 2C). Since higher soil moisture generally stimulates germination (Oryokot et al. Reference Oryokot, Murphy and Swanton1997), a possible explanation of this counterintuitive result is that soil moisture added to the +water subplots through irrigation increased postgermination mortality prior to seedling emergence. Additional water that we applied evaporated quickly in this extremely hot and dry year (Figure 1C). It is possible that moisture persisted long enough for seeds to imbibe and even to commence the germination process, but that moisture was insufficient for complete germination and emergence, especially in FWT treatments. Emergence of small Amaranthus seedlings is susceptible to soil crusting in loamy soils (Bavec and Mlakar Reference Bavec and Mlakar2002); we observed soil crusting to a greater extent in the +water subplots in FWT and ST-IR, which may have further inhibited successful emergence.
Overall, it is not surprising that we generally observed stronger effects of ST relative to FWT in the untilled BR zone compared with tilled IR zone (Figure 2) and that there were more interactions with the cover crop in the BR zone, as this remains as a surface mulch layer in this zone in ST (Table 4). However, our rainfall subplot treatments provided little support for the hypothesis that these tillage effects were mediated by soil moisture.
Oat Cover Crop Effects on Emergence following Early Planting
In-Row (IR). In 2011 oats stimulated emergence of Powell amaranth, but only in the −water treatment (Figure 3A). This is consistent with our original hypothesis that the incorporated oats residue may have increased emergence by relieving some of the moisture limitation in the dry conditions maintained under our precipitation exclosures. In 2012, in contrast, oats suppressed emergence of Powell amaranth, but only under ambient and +water treatments. Moisture additions in this very dry year may have contributed to postgermination, pre-emergence seedling mortality through soil crusting or pathogen stimulation.
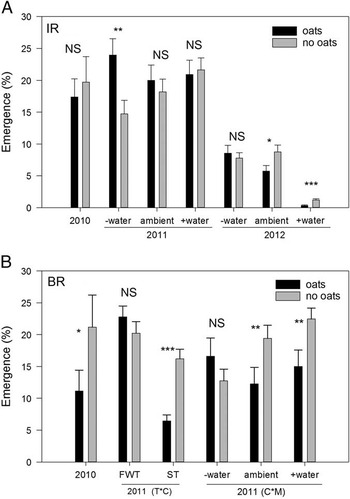
Figure 3 Effects of oat cover crop on emergence of Powell amaranth planted early (sown 0 d after tillage) in-row (IR; A) and between-row (BR; B). Error bars represent ±1 SE. Within each year or interaction, significance levels for the difference between oats and no oats are shown. NS, difference is not significant, FWT, full-width tillage, ST, strip tillage, T, tillage, C, cover crop, M, moisture; *, P<0.05; **, P<0.01; ***, P<0.001.
Between-Row (BR). In all years, emergence of early planted Powell amaranth was either reduced or unaffected by oats compared with no oats (Table 4). In 2010 oats reduced emergence of early planted BR Powell amaranth by 53% (Figure 3B). In 2011 oats reduced emergence of early planted BR Powell amaranth by 60% but only in ST-BR, where the oats residue remained on the soil surface (Figure 3B). Oats residue only reduced emergence in some of the FWT subplot treatments in 2012 (see Figure 2C). Specifically, oats reduced emergence in ambient subplots (effects slicing P=0.008; F(1, 55)=7.52) and in +water subplots (effects slicing P=0.040; F(1,55)=4.45).
The effects of oats residue on Powell amaranth emergence in the BR zone were also mediated by moisture subplots in both 2011 and 2012 (Table 4). In 2011, across both tillage types, oats suppressed emergence of Powell amaranth only in ambient and +water treatments (Figure 3B). One explanation for this result is that the combination of oats residue and high soil moisture stimulated fungal pathogens such as Pythium and Fusarium, which contribute to postgermination pre-emergence mortality (see Mohler et al. Reference Mohler, Dykeman, Nelson and DiTommaso2012). This hypothesis is also consistent with suppressive effects of oats on emergence in 2010, when initial soil moisture conditions were high (Figure 1A).
As in the IR zone, Powell amaranth emergence in 2011 without oats (Figure 3B) was lowest where water was withheld (effects slicing P=0.0003; F(2, 56)=9.43), but emergence with the oats residue was similar regardless of the moisture manipulation (effects slicing P=0.17; F(2, 56)=1.86). In 2012 oats also reduced emergence under ambient and +water treatments, but this effect was only observed under FWT (Figure 2C). Again, we suspect that increased postgermination mortality may have been due to soil crusting. While surface oat residue was expected to increase soil moisture, potentially stimulative moisture effects may have been masked by suppressive effects of these residues—blocking light, reducing soil temperature, and otherwise physically or biologically impeding seedling emergence.
Reductions in BR emergence in ST with oats, observed in 2011, are consistent with multiple studies that demonstrate lower emergence under cover crop residue mulches (Bernstein et al. Reference Bernstein, Stoltenberg, Posner and Hedtcke2014; Campiglia et al. Reference Campiglia, Radicetti and Mancinelli2012; De Bruin et al. Reference De Bruin, Porter and Jordan2005; Nord et al. Reference Nord, Curran, Mortensen, Mirsky and Jones2011; Radicetti et al. Reference Radicetti, Mancinelli and Campiglia2013; Smith et al. Reference Smith, Reberg-Horton, Place, Meijer, Arellano and Mueller2011). However, several studies have noted that this effect can be inconsistent, especially with low cover crop biomass production (e.g., <4,000 kg ha−1; De Bruin et al. Reference De Bruin, Porter and Jordan2005) and later in the season (Mirsky et al. Reference Mirsky, Curran, Mortensen, Ryan and Shumway2011). Oats biomass in our study was less than 3,000 kg ha−1 in all years (Table 3), which could explain why we did not consistently observe lower emergence in the BR zone of ST with oats.
Tillage Effects on Emergence following Late Planting
In 2010 ST increased emergence of Powell amaranth by 72% IR and by 81% BR relative to FWT but only with oats (Figure 4). In contrast, in 2011 ST resulted in lower Powell amaranth emergence relative to FWT in both zones (Figure 4). Manipulating moisture additions did not affect this tillage response in 2011 (Table 5; tillage by moisture interaction NS), suggesting that factors other than moisture were responsible for the suppression of emergence in ST in 2011. In 2010 moisture subplots were not studied, but ambient conditions during late emergence were dry (Figure 1A), so it is possible that observed stimulative effects of ST that year were due to greater moisture retention. However, this hypothesis was not supported by results in 2012, which had comparably dry ambient moisture conditions (Figure 1C).
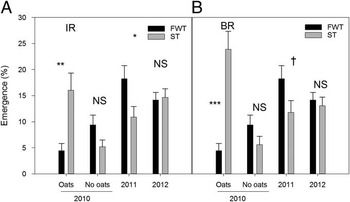
Figure 4 Effect of tillage on emergence of Powell amaranth planted later (sown 7–13 d after tillage) in-row (IR; A) and between-row (BR; B). Error bars ±1 SE. Within each year or interaction, significance levels for the difference between full-width tillage (FWT) and strip tillage (ST) are shown. NS, difference is not significant; †, P<0.10; *, P<0.05; **, P<0.01; ***, P<0.001.
Table 5 Results of a three-way ANOVA for late in-row (IR) and between-row (BR) emergence of Powell amaranth beginning 7 to 13 d after tillage.

a Main plot factors were tillage and cover crop, with moisture treatment (ambient, +water, −water) as the subplot factor.
Oat Cover Crop Effects on Late Emergence
In-Row (IR). In 2010 oats increased emergence of IR Powell amaranth but only in ST (Figure 5A). There was no effect of oats residue in 2011 (Table 5). In 2012 oats reduced emergence of the late-planted IR seeds by approximately one-third in ambient moisture and +water subplots (Figure 5A). This is similar to the effect observed at the early timing, when oats also reduced emergence in these subplots (Figure 3A).
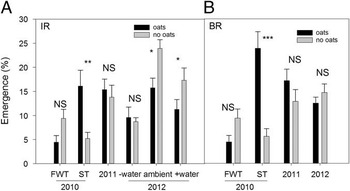
Figure 5 Effect of oat cover crop on emergence of Powell amaranth planted later (sown 7 to 13 d after tillage) in-row (IR; A) and between-row (BR; B). Error bars represent ±1 SE. Within each year or interaction, significance levels for the difference between oats and no oats. NS, difference is not significant; *, P<0.05; **, P<0.01; ***, P<0.001.
Between-Row (BR). Oats residue increased emergence of late-planted Powell amaranth in ST-BR almost threefold relative to no oats in 2010, but no differences were observed in 2011 or 2012 (Table 5; Figure 5B). This increased emergence with oats at the late timing contrasts sharply with the inhibitory effect of oats observed at the early timing (Figure 3B). Interestingly, soil moisture conditions also contrasted sharply between early and late timings in 2010, with wet conditions prevailing for the first week at the early timing, and dry conditions occurring during the period of peak emergence at the late timing (Figure 1A). While these results from 2010 are consistent with the hypothesis that oats suppressive effects are most pronounced under moist conditions, they were not observed consistently.
In conclusion, in the majority of cases examined, ST and oats residue either had no effect or suppressed emergence of Powell amaranth seeds placed near the soil surface immediately after tillage (Figures 2 and 3). These effects were often large—e.g., up to 72% reduction in emergence in ST-BR with oats compared with FWT with oats in one year (Figure 2B)—though variable. Assuming that the density of nondormant Powell amaranth seeds in the germination zone is similar in the two tillage types, our results suggest that growers using ST with cover crops would see lower emergence immediately after tillage relative to a field in FWT. However, when seeds were sown 7 to 13 d after tillage, emergence responses to ST and oats were generally smaller and more variable, with increases in emergence noted in several cases. This finding highlights that growers are not likely to see season-long weed suppression from ST with a spring-planted oat cover crop and that late-season weed emergence may sometimes be greater in ST compared with FWT.
Overall, our results demonstrated large variability in Powell amaranth emergence between years and zones in response to tillage and cover cropping, even when controlling for seed burial depth and dormancy status. For example, on a field scale and summed across both zones, the emergence of sown Powell amaranth seeds in 2010 was 50% lower in ST compared with FWT, regardless of whether a cover crop was used. In the next year, however, Powell amaranth emergence was 44% lower in ST compared with FWT with oat cover crop residue, but only 17% lower without that residue. In a very dry year like 2012, the results were more complicated: in ambient moisture conditions emergence was similar in ST and FWT without a cover crop, while ST resulted in an 84% increase in emergence relative to FWT if an oat cover crop was used.
The relationship between Powell amaranth emergence observed in our study and that which would actually be experienced by a grower adopting CA practices depends on several important factors not evaluated in our study, including tillage-induced impacts on the vertical distribution of seeds in the soil, seed dormancy status, seed predation, and seed rain. Our method of placing seeds with the same dormancy status near the soil surface after tillage facilitates better understanding of the impact of edaphic conditions on emergence (independent of seed depth and dormancy status) but limits our ability to predict the overall weed emergence response governed by these multiple factors. Among these factors, tillage effects on vertical distribution of seeds in the soil is particularly important: since reduced tillage practices, including ST, generally result in a shallower distribution of seeds near the soil surface over time (e.g., Cardina et al. Reference Cardina, Regnier and Harrison1991), the suppressive effects of ST that we observed might be offset by greater seed density in the germination zone. Clearly, improved predictions of weed emergence in response to tillage and cover cropping depends on integration of emergence responses like those examined in this study with population dynamic models that account for other important factors influencing weed emergence.
Our hypothesis that variation in emergence response to tillage and cover cropping could be explained in part by rainfall conditions was not consistently supported by our results. In some cases, emergence of Powell amaranth in response to tillage and cover crops was unaffected by simulated rainfall manipulations, suggesting that other mechanisms such as changes in soil physical conditions (e.g., surface crusting), fungal pathogens, allelopathy, or temperature effects were more important. However, in several cases, particularly for oat-induced effects on emergence, rainfall/irrigation appeared to play an important role. In particular, oats residue increased emergence most often in dry conditions, while emergence was suppressed most commonly in wetter conditions.
While some generalizations about the emergence response can be made, the observed variability in our study highlights two key points: (1) more complex CA systems are likely to result in more variable and complex responses by the weed community then FWT practices, and (2) further research is needed to elucidate mechanisms responsible for this variability. Because our results demonstrated strong spatial and temporal variability in emergence responses, development of management practices targeting distinct zones and timings (e.g., zonal cover cropping, IR cultivation tools, or slow-release banded fertilization) will likely be particularly helpful for overcoming weed management constraints in these systems.
Acknowledgments
This project was supported by funding from a University Distinguished Fellowship award from Michigan State University (MSU); a C. S. Mott Pre-Doctoral Fellowship from the Center for Regional Food Systems at MSU; and grants from MSU Project GREEEN (GR10-118) and the USDA-NCR-SARE program (LNC11-330). Support for weather data collection at the experimental site was also provided by the NSF Long-Term Ecological Research Program at the Kellogg Biological Station and by Michigan State University AgBioResearch. We thank Zachary Hayden, Ben Henshaw, Frank Horton, Carolyn Lowry, Corey Noyes, Nate Robinson, Joe Simmons, and many undergraduate research assistants for their technical assistance. We also thank Alan Taylor, Christy Sprague, Sieglinde Snapp, and Scott Swinton and two anonymous reviewers and an Associate Editor whose thoughtful comments greatly strengthened this manuscript.