Book contents
- The Handbook of Medical Image Perception and Techniques, Second Edition
- Reviews
- The Handbook of Medical Image Perception and Techniques
- Copyright page
- Dedication
- Contents
- Contributors
- 1 Medical Image Perception
- Part I Historical Reflections and Theoretical Foundations
- 2 A Short History of Image Perception in Medical Radiology
- 3 Spatial Vision Research without Noise
- 4 Signal Detection Theory: A Brief History
- 5 Signal Detection in Radiology
- 6 Lessons from Dinners with the Giants of Modern Image Science*
- 7 Perception in Context
- Part II Science of Image Perception
- Part III Perception Metrology
- Part IV Clinical Performance Assessment
- Part V Computational Perception
- Part VI Applied Perception
- Index
- References
5 - Signal Detection in Radiology
from Part I - Historical Reflections and Theoretical Foundations
Published online by Cambridge University Press: 20 December 2018
- The Handbook of Medical Image Perception and Techniques, Second Edition
- Reviews
- The Handbook of Medical Image Perception and Techniques
- Copyright page
- Dedication
- Contents
- Contributors
- 1 Medical Image Perception
- Part I Historical Reflections and Theoretical Foundations
- 2 A Short History of Image Perception in Medical Radiology
- 3 Spatial Vision Research without Noise
- 4 Signal Detection Theory: A Brief History
- 5 Signal Detection in Radiology
- 6 Lessons from Dinners with the Giants of Modern Image Science*
- 7 Perception in Context
- Part II Science of Image Perception
- Part III Perception Metrology
- Part IV Clinical Performance Assessment
- Part V Computational Perception
- Part VI Applied Perception
- Index
- References
Summary
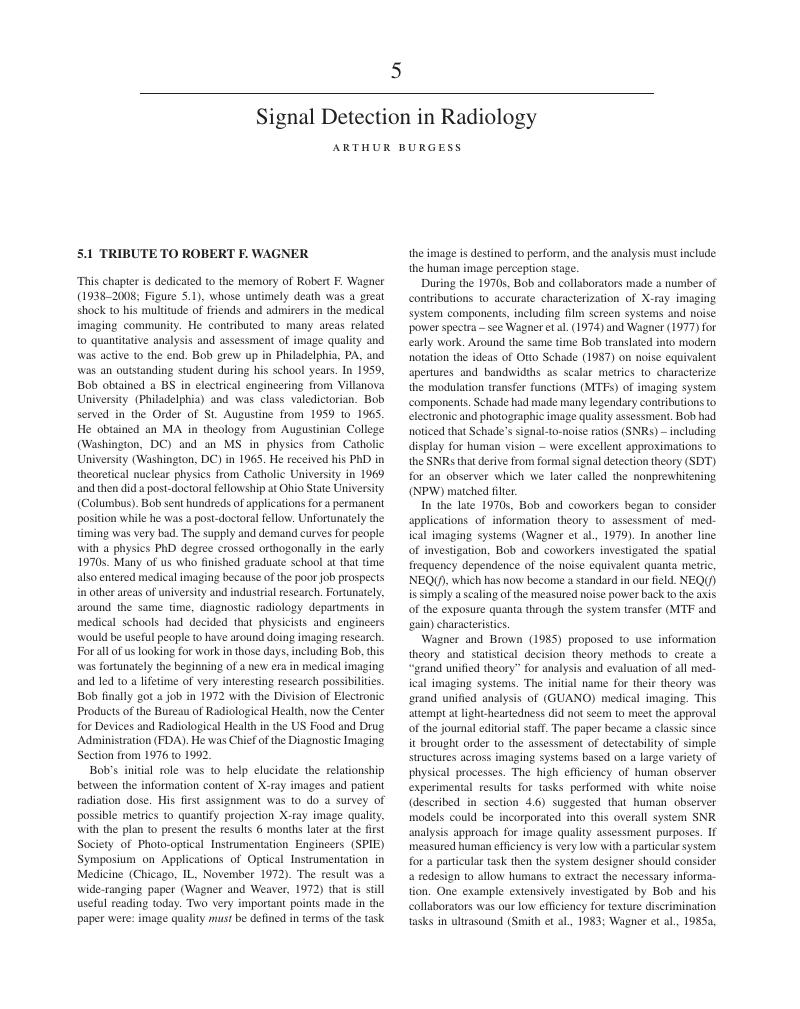
- Type
- Chapter
- Information
- The Handbook of Medical Image Perception and Techniques , pp. 49 - 75Publisher: Cambridge University PressPrint publication year: 2018
References
- 1
- Cited by