Book contents
- Magnetic Nanoparticles in Biosensing and Medicine
- Magnetic Nanoparticles in Biosensing and Medicine
- Copyright page
- Contents
- Contributors
- Preface
- Abbreviations
- 1 Magnetism, Magnetic Materials, and Nanoparticles
- 2 Preparation of Magnetic Nanoparticles for Applications in Biomedicine
- 3 Magnetic Nanoparticle Functionalization
- 4 Manipulation
- 5 Modeling the In-Flow Capture of Magnetic Nanoparticles
- 6 Sensing Magnetic Nanoparticles
- 7 Magnetic Nanoparticles for Magnetic Resonance Imaging Contrast Agents
- 8 Magnetotactic Bacteria and Magnetosomes
- Appendix: Units of Magnetic Properties
- Index
- References
8 - Magnetotactic Bacteria and Magnetosomes
Published online by Cambridge University Press: 10 February 2019
- Magnetic Nanoparticles in Biosensing and Medicine
- Magnetic Nanoparticles in Biosensing and Medicine
- Copyright page
- Contents
- Contributors
- Preface
- Abbreviations
- 1 Magnetism, Magnetic Materials, and Nanoparticles
- 2 Preparation of Magnetic Nanoparticles for Applications in Biomedicine
- 3 Magnetic Nanoparticle Functionalization
- 4 Manipulation
- 5 Modeling the In-Flow Capture of Magnetic Nanoparticles
- 6 Sensing Magnetic Nanoparticles
- 7 Magnetic Nanoparticles for Magnetic Resonance Imaging Contrast Agents
- 8 Magnetotactic Bacteria and Magnetosomes
- Appendix: Units of Magnetic Properties
- Index
- References
Summary
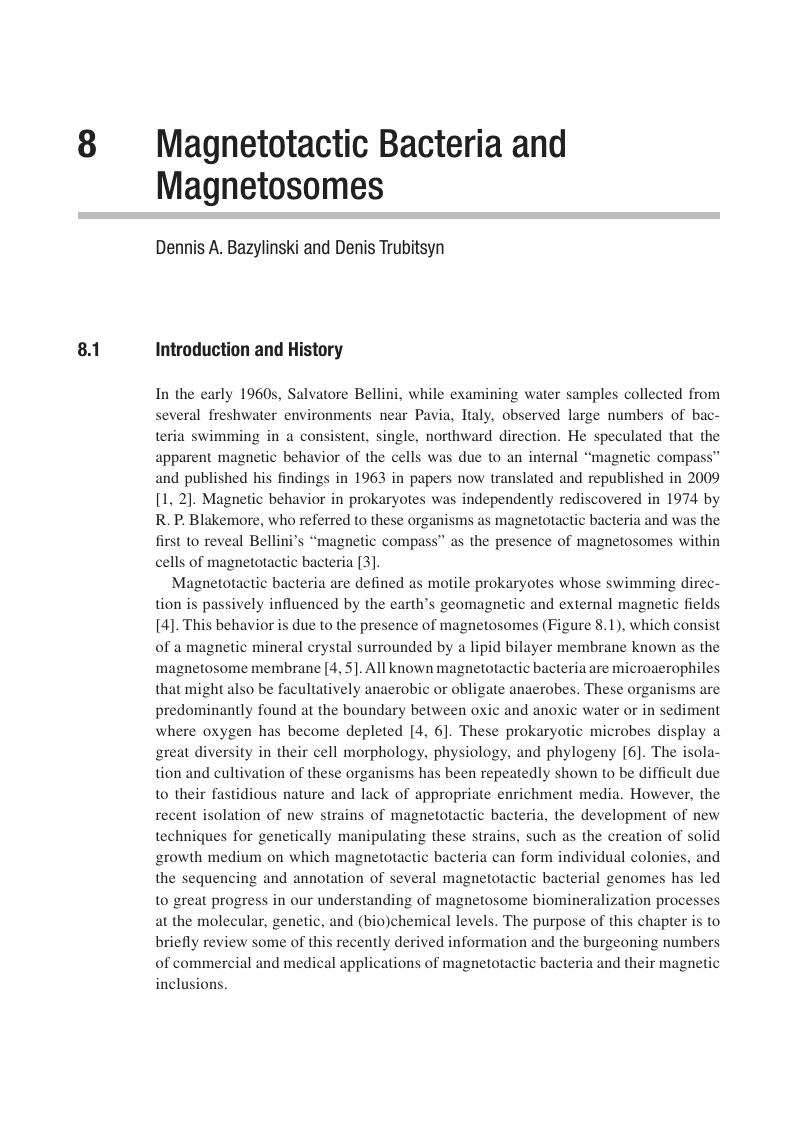
- Type
- Chapter
- Information
- Magnetic Nanoparticles in Biosensing and Medicine , pp. 251 - 284Publisher: Cambridge University PressPrint publication year: 2019
References
- 4
- Cited by