Book contents
- Clays in the Minerals Processing Value Chain
- Clays in the Minerals Processing Value Chain
- Copyright page
- Contents
- Contributors
- Reviewers
- Foreword
- Preface
- 1 Clays and the Minerals Processing Value Chain (MPVC)
- 2 Fundamentals of Clays Surface and Colloid Science, and Rheology
- 3 Leaching and Solution Chemistry
- 4 Identification and Quantification of Clays
- 5 Clays by Deposit Type
- 6 Exploration and Discovery
- 7 Clay Minerals in Flotation and Comminution Operations
- 8 Solid–Liquid Separation of Clay Tailings
- 9 Effects of Clay in Tailings Handling and Storage
- 10 Knowledge and Practice Gaps and Research Priorities for Clay-Related Phenomena in the Minerals Processing Value Chain
- Glossary
- Index
- References
7 - Clay Minerals in Flotation and Comminution Operations
Published online by Cambridge University Press: 30 August 2017
- Clays in the Minerals Processing Value Chain
- Clays in the Minerals Processing Value Chain
- Copyright page
- Contents
- Contributors
- Reviewers
- Foreword
- Preface
- 1 Clays and the Minerals Processing Value Chain (MPVC)
- 2 Fundamentals of Clays Surface and Colloid Science, and Rheology
- 3 Leaching and Solution Chemistry
- 4 Identification and Quantification of Clays
- 5 Clays by Deposit Type
- 6 Exploration and Discovery
- 7 Clay Minerals in Flotation and Comminution Operations
- 8 Solid–Liquid Separation of Clay Tailings
- 9 Effects of Clay in Tailings Handling and Storage
- 10 Knowledge and Practice Gaps and Research Priorities for Clay-Related Phenomena in the Minerals Processing Value Chain
- Glossary
- Index
- References
Summary
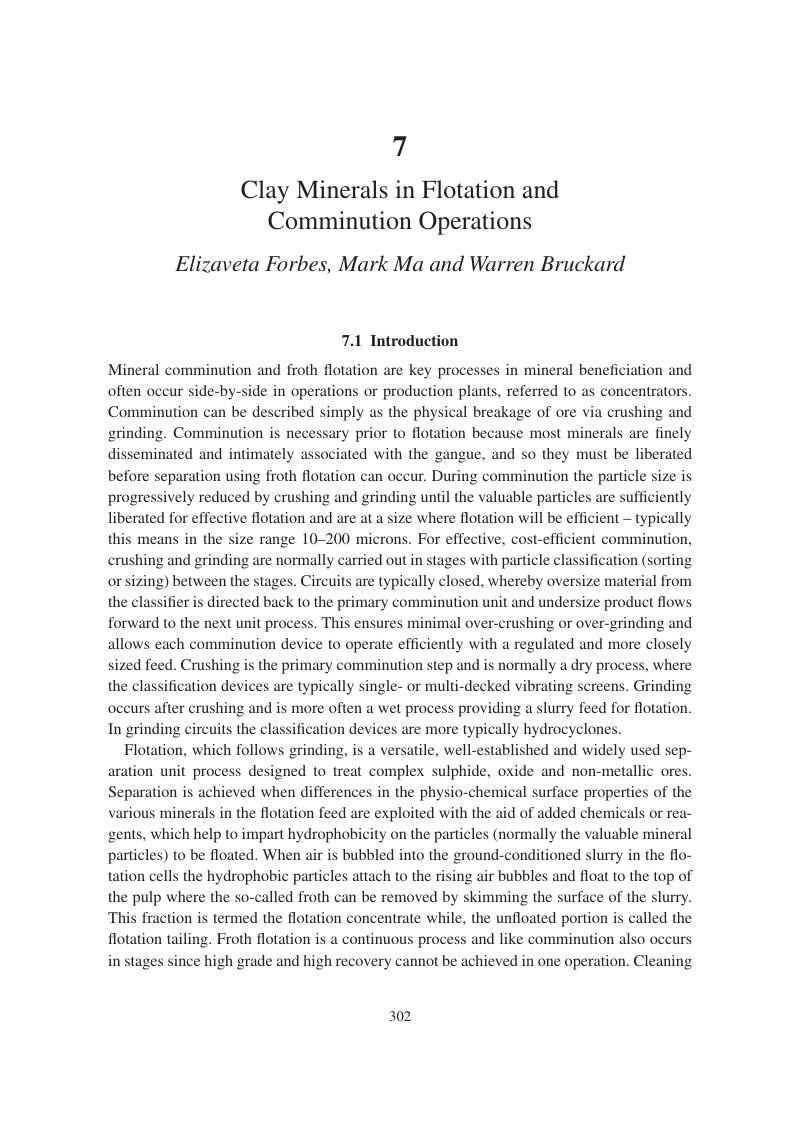
- Type
- Chapter
- Information
- Clays in the Minerals Processing Value Chain , pp. 302 - 326Publisher: Cambridge University PressPrint publication year: 2017
References
- 1
- Cited by