Book contents
- Frontmatter
- Contents
- Acknowledgments
- Introduction: Biology and physics
- 1 The cell: fundamental unit of developmental systems
- 2 Cleavage and blastula formation
- 3 Cell states: stability, oscillation, differentiation
- 4 Cell adhesion, compartmentalization, and lumen formation
- 5 Epithelial morphogenesis: gastrulation and neurulation
- 6 Mesenchymal morphogenesis
- 7 Pattern formation: segmentation, axes, and asymmetry
- 8 Organogenesis
- 9 Fertilization: generating one living dynamical system from two
- 10 Evolution of developmental mechanisms
- Glossary
- References
- Index
- References
References
Published online by Cambridge University Press: 24 May 2010
- Frontmatter
- Contents
- Acknowledgments
- Introduction: Biology and physics
- 1 The cell: fundamental unit of developmental systems
- 2 Cleavage and blastula formation
- 3 Cell states: stability, oscillation, differentiation
- 4 Cell adhesion, compartmentalization, and lumen formation
- 5 Epithelial morphogenesis: gastrulation and neurulation
- 6 Mesenchymal morphogenesis
- 7 Pattern formation: segmentation, axes, and asymmetry
- 8 Organogenesis
- 9 Fertilization: generating one living dynamical system from two
- 10 Evolution of developmental mechanisms
- Glossary
- References
- Index
- References
Summary
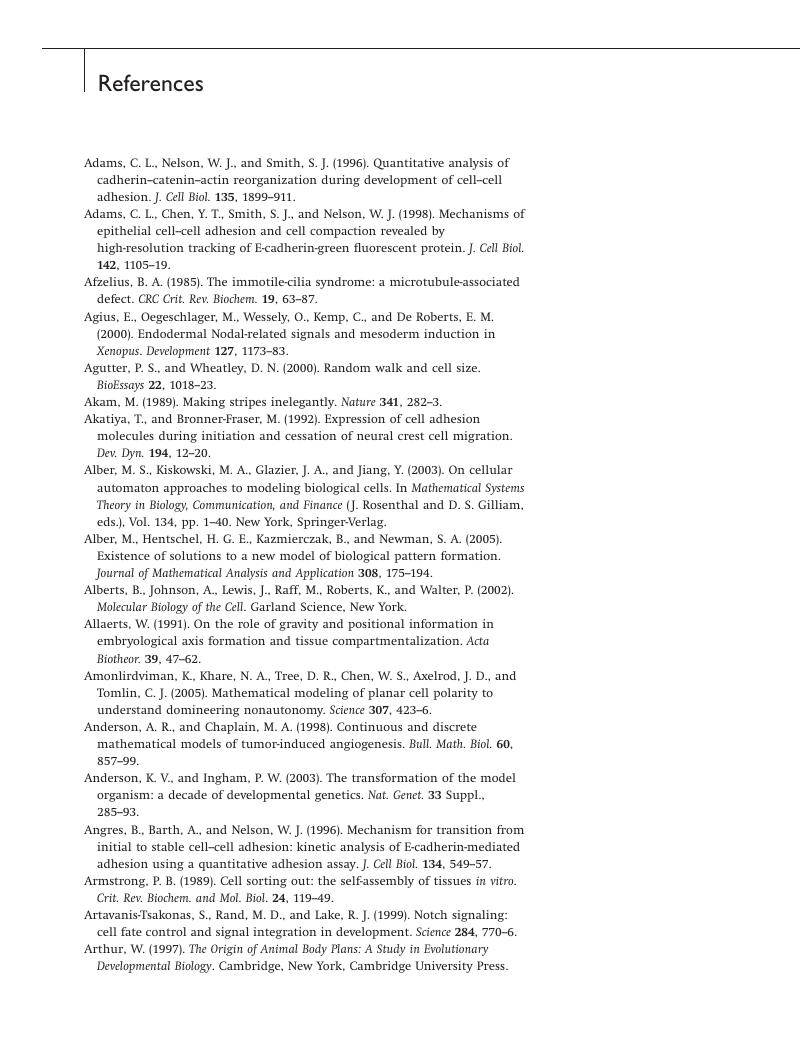
- Type
- Chapter
- Information
- Biological Physics of the Developing Embryo , pp. 291 - 326Publisher: Cambridge University PressPrint publication year: 2005