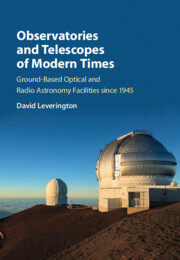
Book contents
- Frontmatter
- Contents
- Preface
- Part 1 Optical Observatories
- 1 Palomar Mountain Observatory
- 2 The United States Optical Observatory
- 3 From the Next Generation Telescope to Gemini and SOAR
- 4 Competing Primary Mirror Designs
- 5 Active Optics, Adaptive Optics and Other Technical Innovations
- 6 European Northern Observatory and Calar Alto
- 7 European Southern Observatory
- 8 Mauna Kea Observatory
- 9 Australian Optical Observatories
- 10 Mount Hopkins' Whipple Observatory and the MMT
- 11 Apache Point Observatory
- 12 Carnegie Southern Observatory (Las Campanas)
- 13 Mount Graham International Optical Observatory
- 14 Modern Optical Interferometers
- 15 Solar Observatories
- Part 2 Radio Observatories
- Name Index
- Optical/ Infrared Observatory and Telescope Index
- Radio Observatory and Telescope Index
- General Index
- References
14 - Modern Optical Interferometers
from Part 1 - Optical Observatories
Published online by Cambridge University Press: 15 December 2016
- Frontmatter
- Contents
- Preface
- Part 1 Optical Observatories
- 1 Palomar Mountain Observatory
- 2 The United States Optical Observatory
- 3 From the Next Generation Telescope to Gemini and SOAR
- 4 Competing Primary Mirror Designs
- 5 Active Optics, Adaptive Optics and Other Technical Innovations
- 6 European Northern Observatory and Calar Alto
- 7 European Southern Observatory
- 8 Mauna Kea Observatory
- 9 Australian Optical Observatories
- 10 Mount Hopkins' Whipple Observatory and the MMT
- 11 Apache Point Observatory
- 12 Carnegie Southern Observatory (Las Campanas)
- 13 Mount Graham International Optical Observatory
- 14 Modern Optical Interferometers
- 15 Solar Observatories
- Part 2 Radio Observatories
- Name Index
- Optical/ Infrared Observatory and Telescope Index
- Radio Observatory and Telescope Index
- General Index
- References
Summary
Mount Wilson
Michelson had designed a stellar interferometer for the Mount Wilson Observatory in 1920 that consisted of a 6 m (20 ft) long steel girder and two 15 cm (6 inch) movable mirrors which he mounted across the open end of the 2.5 m (100 inch) telescope.(1) This enabled Michelson and Pease to measure the diameter of a number of red giants. However, little more interferometric work was done on Mount Wilson until 1979 when Michael Shao and David Staelin of the Massachusetts Institute of Technology designed a two telescope, white light, 1.5 m (5 ft) baseline interferometer. It was the first interferometer to compensate for atmospheric turbulence by making continuous phase and amplitude measurements with a 4 ms integration time. The Mark I was succeeded by the 3.1 m Mark II interferometer which was used as a technology testbed for astrometric measurements from 1982 to 1984. It incorporated a high speed, ultra high accuracy, laser controlled optical delay line, and it was also used to develop an operational procedure for rapid switching between stars. The Mark II was, in turn, followed by the Mark III(2) from 1986 to 1993 which had a maximum baseline of 32 m and operated in two spectral bands from about 400 to 600 nm and from about 600 to 900 nm. Like the Mark II, the Mark III was designed to test out new techniques and technologies. Its aim was to be reliable and easy to operate, whilst being capable of extremely accurate astronomical measurements of stellar positions and diameters. Lessons learnt in operating the Mark III were applied to the design of the US Naval Observatory's NPOI (Navy Prototype Optical Interferometer, see Section 14.6) located in the mountains near Flagstaff, Arizona which became operational in 1996.
Berkeley Infrared Spatial Interferometer (ISI)
Instead of using the Michelson homodyne or direct detection system of the above instruments, Charles Townes, Mike Johnson and Al Betz of the University of California (UC) at Berkeley(3) used a heterodyne system in 1974 to produce the first mid-infrared interferometer based on techniques used in radio astronomy.
- Type
- Chapter
- Information
- Observatories and Telescopes of Modern TimesGround-Based Optical and Radio Astronomy Facilities since 1945, pp. 244 - 253Publisher: Cambridge University PressPrint publication year: 2016