Book contents
- Frontmatter
- Dedication
- Contents
- Preface
- Chapter 1 Geochemical models
- Chapter 2 Modeling tools
- Chapter 3 Rate equations
- Chapter 4 Chemical reactors
- Chapter 5 Molecular kinetics
- Chapter 6 Surface kinetics
- Chapter 7 Diffusion and advection
- Chapter 8 Quasi-kinetics
- Chapter 9 Accretion and transformation kinetics
- Chapter 10 Pattern formation
- References
- Index
- References
References
Published online by Cambridge University Press: 05 June 2014
- Frontmatter
- Dedication
- Contents
- Preface
- Chapter 1 Geochemical models
- Chapter 2 Modeling tools
- Chapter 3 Rate equations
- Chapter 4 Chemical reactors
- Chapter 5 Molecular kinetics
- Chapter 6 Surface kinetics
- Chapter 7 Diffusion and advection
- Chapter 8 Quasi-kinetics
- Chapter 9 Accretion and transformation kinetics
- Chapter 10 Pattern formation
- References
- Index
- References
Summary
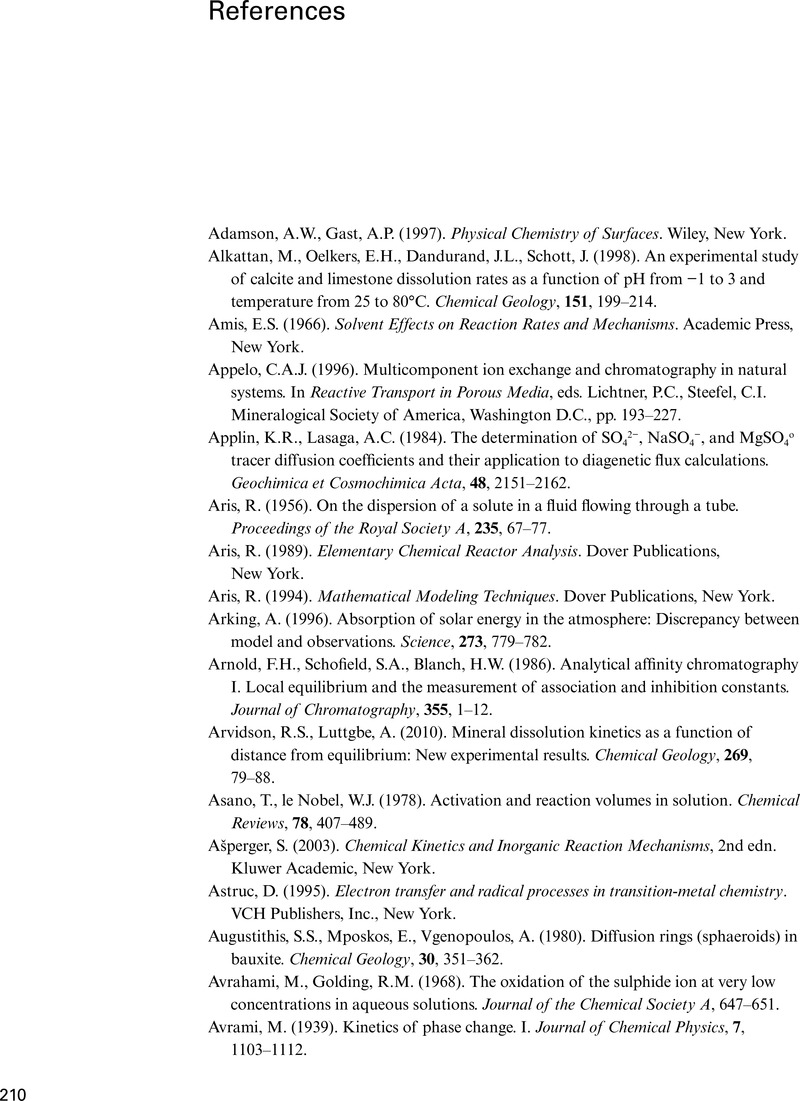
- Type
- Chapter
- Information
- Geochemical Rate ModelsAn Introduction to Geochemical Kinetics, pp. 210 - 227Publisher: Cambridge University PressPrint publication year: 2013