Book contents
- Neurorehabilitation Therapy and Therapeutics
- Neurorehabilitation Therapy and Therapeutics
- Copyright page
- Dedication
- Contents
- Contributors
- Chapter 1 An Introduction to Neurological Rehabilitation
- Chapter 2 Management of Disorders of Cognition in Neurorehabilitation
- Chapter 3 Management of Mood and Behaviour in Neurorehabilitation
- Chapter 4 Management of Disorders of Consciousness in Neurorehabilitation
- Chapter 5 Management of Communication Disorders in Neurorehabilitation
- Chapter 6 Management of Disorders of Eating, Drinking, and Swallowing in Neurorehabilitation
- Chapter 7 Management of Salivary Disorders in Neurorehabilitation
- Chapter 8 Management of Upper Limb Impairment in Neurorehabilitation
- Chapter 9 Management of Vestibular Disorders in Neurorehabilitation
- Chapter 10 Management of Walking Disorders in Neurorehabilitation
- Chapter 11 Management of Spasticity in Neurorehabilitation
- Chapter 12 Neurorehabilitation in Parkinson’s Disease and Parkinsonism
- Chapter 13 Neuropathic Pain
- Chapter 14 Management of Phantom Limb in Neurorehabilitation
- Chapter 15 Management of Neuro-Ophthalmologic Disorders in Neurorehabilitation
- Chapter 16 Management of Pressure Ulcers in Neurological Rehabilitation
- Chapter 17 Management of Disorders of Blood Pressure Control in Neurorehabilitation
- Chapter 18 Management of Neurogenic Lower Urinary Tract Dysfunction
- Chapter 19 Management of Neurogenic Bowel Dysfunction
- Chapter 20 Management of Neurogenic Sexual Dysfunction
- Index
- References
Chapter 8 - Management of Upper Limb Impairment in Neurorehabilitation
Published online by Cambridge University Press: 13 October 2018
- Neurorehabilitation Therapy and Therapeutics
- Neurorehabilitation Therapy and Therapeutics
- Copyright page
- Dedication
- Contents
- Contributors
- Chapter 1 An Introduction to Neurological Rehabilitation
- Chapter 2 Management of Disorders of Cognition in Neurorehabilitation
- Chapter 3 Management of Mood and Behaviour in Neurorehabilitation
- Chapter 4 Management of Disorders of Consciousness in Neurorehabilitation
- Chapter 5 Management of Communication Disorders in Neurorehabilitation
- Chapter 6 Management of Disorders of Eating, Drinking, and Swallowing in Neurorehabilitation
- Chapter 7 Management of Salivary Disorders in Neurorehabilitation
- Chapter 8 Management of Upper Limb Impairment in Neurorehabilitation
- Chapter 9 Management of Vestibular Disorders in Neurorehabilitation
- Chapter 10 Management of Walking Disorders in Neurorehabilitation
- Chapter 11 Management of Spasticity in Neurorehabilitation
- Chapter 12 Neurorehabilitation in Parkinson’s Disease and Parkinsonism
- Chapter 13 Neuropathic Pain
- Chapter 14 Management of Phantom Limb in Neurorehabilitation
- Chapter 15 Management of Neuro-Ophthalmologic Disorders in Neurorehabilitation
- Chapter 16 Management of Pressure Ulcers in Neurological Rehabilitation
- Chapter 17 Management of Disorders of Blood Pressure Control in Neurorehabilitation
- Chapter 18 Management of Neurogenic Lower Urinary Tract Dysfunction
- Chapter 19 Management of Neurogenic Bowel Dysfunction
- Chapter 20 Management of Neurogenic Sexual Dysfunction
- Index
- References
Summary
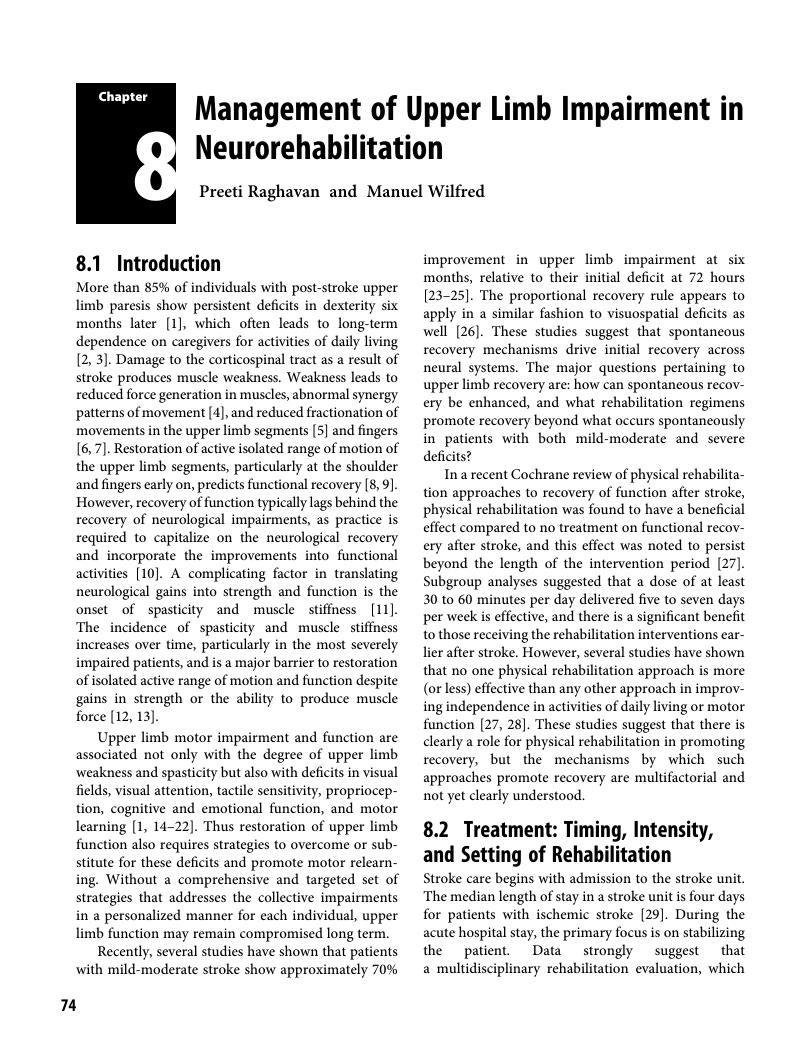
- Type
- Chapter
- Information
- Neurorehabilitation Therapy and Therapeutics , pp. 74 - 89Publisher: Cambridge University PressPrint publication year: 2018