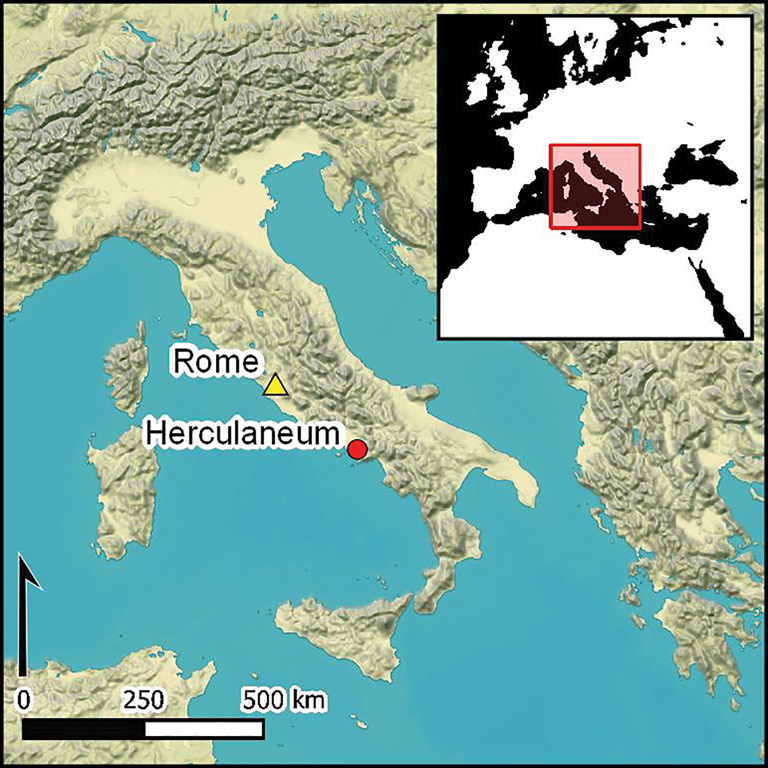
Introduction
The eruption of Mount Vesuvius on 24 August, AD 79 is one of the most iconic and archaeologically well-attested ancient natural disasters. The exceptional preservation and quantity of archaeological material recovered from sites such as Pompeii, Herculaneum, Oplontis and Stabiae present a detailed picture of Roman life before the eruption. The skeletal assemblage uncovered on the former seafront of Herculaneum, and from within 10 of the 12 fornici—a series of vaulted stone chambers adjacent to the beach (Figure 1)—offers a unique snapshot of the lives and deaths of the town's inhabitants. Recent developments in bioarchaeological analysis have catalysed debate over the nature and manner of the inhabitants’ deaths (Mastrolorenzo et al. Reference Mastrolorenzo, Petrone, Pagano, Incoronato, Baxter, Canzanella and Fattore2001, Reference Mastrolorenzo, Petrone, Pappalardo and Guarino2010; Petrone Reference Petrone2011; Fattore et al. Reference Fattore, Bondioli, Garnsey, Rossi and Sperduti2012; Schmidt et al. Reference Schmidt, Oakley, D'Anastasio, Brower, Remy, Viciano, Schmidt and Symes2015). The remains also provide a unique opportunity to examine the lethal effects of pyroclastic surges from what is still an active volcano in a densely inhabited contemporary landscape (Mastrolorenzo et al. Reference Mastrolorenzo, Petrone, Pappalardo and Guarino2010; Petrone et al. Reference Petrone2018).

Figure 1. The fornici of Herculaneum (figure by the authors).
Establishing the intensity of thermal pyroclastic exposure to which the Herculaneum victims were subjected fundamentally influences our understanding of both the cause of death and the effects of such heat exposure on the nature of bone diagenesis and collagen degradation. This last point is significant, as these changes may influence the range of subsequent bioarchaeological analyses, particularly biomolecular (Smith et al. Reference Smith, Chamberlain, Riley, Cooper, Stringer and Collins2001; Collins et al. Reference Collins, Nielsen-Marsh, Hiller, Smith and Roberts2002).
The aim of this study is to apply newly developed analytical methods to bone recovered from the fornici in order to describe the context of death. While these methods have contributed to a more nuanced understanding of other cremation contexts, they have not to date been applied. The results offer an unparalleled insight into the impact of this natural disaster on the bodies of ancient Roman individuals.
Background
The human skeletal sample recovered from excavations at Herculaneum represents a portion of the ‘living’ population who died trying to escape from the eruption of Vesuvius in AD 79. In total, 340 individuals have been excavated from the beach and from the adjacent fornici, in which they sought shelter.
The human remains from the seafront were recovered during three separate phases. The first, between 1980 and 1985, revealed approximately 150 skeletons from fornici 3, 4 and (partially) 5, the area surrounding the central stairs that link the city to the beach, and the beach itself. A second group of around 75 skeletons was recovered between 1997 and 1999 from fornici 5, 10 and 12. The third phase took place from 2008–2012, and recovered approximately 104 skeletons from fornici 7, 8, 9 and 11, and from within a niche of fornice 10.
Demographic analysis of the whole sample demonstrates a slightly unbalanced sex ratio (males/females = 1:2.2) and age distribution, with fewer than expected infants and children for an ancient population (Fattore et al. Reference Fattore, Bondioli, Garnsey, Rossi and Sperduti2012). While this underrepresentation could reflect the way in which the population sought to escape the eruption, we should also consider the peculiar demographic dynamics of the city as attested by the analysis of the Album, an inscription in marble carrying a list of names of Herculaneum's inhabitants (de Ligt & Garnsey Reference de Ligt and Garnsey2012).
The most notable aspect of the sample's demographic profile concerns the sex and age composition of the individuals found on the beach in comparison with those found in the boat chambers (Figure 2). Adult and young adult males died primarily, although not exclusively, on the exposed beach, while the demographic within the fornici comprised mainly adult females and juveniles/infants, most of them possibly related (Fattore et al. Reference Fattore, Bondioli, Garnsey, Rossi and Sperduti2012; Figures 3–4). This evidence offers insight into how the Herculaneum residents reacted to the catastrophe and organised their escape.

Figure 2. Distribution by sex (for adults) and by age (infants 0–10 years old; juveniles 10–15 years old) of the whole sample recovered from the beach and from inside the fornici (figure by the authors).

Figure 3. The in situ human remains preserved inside one of the stone chambers (figure by the authors).

Figure 4. Excavation plan and 3D high-resolution laser scan record of the fornice 8 excavation; note the overlaying of the bodies and the commingled context (figure by the authors).
The boat chambers and the beach provided very different environmental conditions when the pyroclastic flow hit. As the individuals within the fornici were far less exposed than those on the beach, their manner of death may reflect these differences.
Death by volcanic activity can leave no trace on the body (Makoye Ng'Walali et al. Reference Makoye Ng'Walali, Koreeda, Kibayashi and Tsunenari1999). Forensic and histological investigations of the Herculaneum victims from both contexts, however, have shown the cause of death to be instantaneous, thermally induced fulminant shock (Mastrolorenzo et al. Reference Mastrolorenzo, Petrone, Pagano, Incoronato, Baxter, Canzanella and Fattore2001, Reference Mastrolorenzo, Petrone, Pappalardo and Guarino2010; Petrone Reference Petrone2011; Petrone et al. Reference Petrone2018). Histological analysis of the skeletons from the beach at Herculaneum and of individuals from Oplontis have revealed: bone discolouration varying from black to grey-white; the presence of both linear and polygonal microcracking; dental enamel cracks; cranial fractures as a result of thermally induced intracranial pressure; and incipient to high crystallisation of bone hydroxyapatite (Mastrolorenzo et al. Reference Mastrolorenzo, Petrone, Pagano, Incoronato, Baxter, Canzanella and Fattore2001, Reference Mastrolorenzo, Petrone, Pappalardo and Guarino2010). The lack of detectable DNA from victims found on the beach (Mastrolorenzo et al. Reference Mastrolorenzo, Petrone, Pappalardo and Guarino2010) and of proteins in those from the chambers (Petrone et al. Reference Petrone2018) also supports the hypothesis of exposure to high pyroclastic temperatures. Furthermore, it is suggested that the lack of the boxer position, or pugilistic attitude, in many of the victims at Herculaneum also denotes instant vaporisation of the soft tissues upon impact. Absorption of thermal energy by these soft tissues is believed to have subsequently caused a rapid decrease in aerospheric temperature within the fornici (Mastrolorenzo et al. Reference Mastrolorenzo, Petrone, Pagano, Incoronato, Baxter, Canzanella and Fattore2001, Reference Mastrolorenzo, Petrone, Pappalardo and Guarino2010; Petrone et al. Reference Petrone2018).
Others, however, suggest a lower pyroclastic surge temperature of around 350–400°C, and a manner of death concordant with the gradual dehydration and contraction of the victims’ muscles and internal organs (e.g. Capasso Reference Capasso2000). Observations of brown rather than blackened bone and the apparent lack of significant cracking in the long bones and cranial elements suggest minimal exposure to direct heat. Recent reanalysis of skeletal morphology by Schmidt et al. (Reference Schmidt, Oakley, D'Anastasio, Brower, Remy, Viciano, Schmidt and Symes2015) supports this conclusion, explicitly attributing the lack of fractures and discolouration to the presence of soft tissue. Indeed, examples of cranial staining may demonstrate the pooling of soft tissue during decomposition. Cranial fractures are inferred to be the result of impact with debris brought into the fornici by the pyroclastic flow, and the presence of the pugilistic attitude may be attributed to soft tissue contraction and shortening (Schmidt et al. Reference Schmidt, Oakley, D'Anastasio, Brower, Remy, Viciano, Schmidt and Symes2015). The former explanation, however, should be regarded with caution, as there is little evidence to suggest that the fatal surge carried such debris along with it. Nonetheless, the inferred presence or absence of soft tissue plays a key role in the assessment of the manner of death. Evidence from other (non-skeletal) material at Herculaneum also argues for a lower overall temperature (e.g. Caricchi et al. Reference Caricchi, Vona, Corrado, Giordano and Romano2014).
Recent advances in the study of burned bone
Despite their magnitude and often widespread damage, research into the effects of volcanic eruptions on the human body is notably absent in the scholarly literature. Recent growing interest in the analysis of burned and cremated human remains (e.g. Thompson Reference Thompson and Thompson2015a), however, has focused on the development and refinement of new methodologies, particularly regarding the analysis of bone microstructure. By applying a range of analytical methods and imaging modalities, studies have demonstrated that subtle and complex changes occur within the bone structure as a result of thermal exposure (e.g. Boschin et al. Reference Boschin, Zanolli, Bernardini, Princivalle and Tuniz2015; Thompson Reference Thompson and Thompson2015a & Reference Thompson, Schmidt and Symesb; Ellingham et al. Reference Ellingham, Thompson and Islam2015, Reference Ellingham, Thompson and Islam2016; Cascant et al. Reference Cascant, Rubio, Gallello, Pastor, Garrigues and de la Guardia2017). Crucially, it has been argued that these changes can be correlated with specific burning conditions, and hence allow for the contextual interpretation of the event itself.
With this in mind, attempts have been made to offer refined interpretations of funerary behaviour based on cremated human remains (Gonçalves & Pires Reference Gonçalves and Pires2015; Thompson Reference Thompson and Thompson2015a). A recent study of Roman soldiers along the Northern Frontier, for example, demonstrates the potential for inferring aspects of individual identity through the combined analysis of microscopic heat-induced changes in cremated bone and pyre artefacts (Thompson et al. Reference Thompson, Szigeti, Gowland and Witcher2016). Squires (Reference Squires and Thompson2015: 168) undertook a similar approach to the study of Anglo-Saxon remains from the UK, noting that “it is equally likely that the time and resources expended on the cremation process were just as significant [as those invested in creating the grave good assemblages]”. Piga et al. (Reference Piga, Guirguis, Thompson, Isidro, Enzo and Malgosa2016) combine results of bone microstructure analysis and osteological assessment to identify the incomplete cremation of a pregnant female from a Phoenician-Punic site in Sardinia, Italy. Such analyses, performed in tandem with similar work (Keough et al. Reference Keough, L'Abbé, Steyn and Pretorius2015), allow for a more nuanced interpretation of the pre-burning condition (e.g. fleshed or defleshed) of human remains.
Thus, recent methodological and conceptual developments, which have been applied in archaeological contexts around the world (Thompson Reference Thompson, Schmidt and Symes2015b), suggest that it is now possible to examine the burned remains from Herculaneum from a new perspective.
Collagen quality and thermal diagenesis
In fresh, unaltered mammalian bone, collagen represents approximately 22 per cent of the total weight (van Klinken Reference van Klinken1999). Diagenetic pressures, such as heat, acidity, microbial attack and hydrology can cause collagen loss (Jans et al. Reference Jans, Nielson-Marsh, Smith, Collins and Kars2004; Tibbett & Carter Reference Tibbett and Carter2008; Milner et al. Reference Milner2011)—a pervading concern in bioarchaeological studies. Research suggests, however, that collagen is remarkably robust. The sequencing of type I collagen from specimens dating to both the Middle Pleistocene (c. 780–125 ka) and the Lower Pleistocene (c. 1.5 mya), for example, presents compelling evidence for its longevity and survival (Buckley & Collins Reference Buckley and Collins2011). It is also generally accepted that even in cases of low total abundance (yield), the structural and chemical integrity of collagen remains largely intact. Dobberstein et al. (Reference Dobberstein, Collins, Craig, Taylor, Penkman and Ritz-Timme2009) suggest that 99 per cent of total collagen is lost before amino acid, isotopic and elemental (carbon:nitrogen) disruption occurs. This one per cent threshold is used in isotopic studies as one of several key criteria by which collagen quality is determined (DeNiro Reference DeNiro1985; van Klinken Reference van Klinken1999).
Within the past few decades, the study of thermally induced collagen diagenesis—as with bioapatite—has become increasingly pervasive in archaeology. It is now widely accepted that the structural relationship between bioapatite and collagen plays a mutually advantageous role in resisting thermally induced degradation, although scholarly opinion differs with regard to which tissue (if either) is more responsible for maintaining overall integrity. Some argue that collagen protects bioapatite from thermal degradation by acting as a ‘thermal shield’, reducing porosity and thus the overall mineral surface area exposed to the environment (Person et al. Reference Person, Bocherens, Mariotti and Renard1996; Trueman et al. Reference Trueman, Privat and Field2008; Lebon et al. Reference Lebon2010; Thompson et al. Reference Thompson, Islam and Bonniere2013). Others propose that the mineral phase offers collagen protection from diagenetic alteration, acting as fracture centres that, when split by thermal energy, expose the collagen to diagenesis (Etok et al. Reference Etok2007). In the context of boiling, during which temperatures remain relatively low (≤100°C), Koon et al. (Reference Koon, Loreille, Covington, Christensen, Parsons and Collins2008) suggest that although the morphology of the collagen fibrils can be thermally altered, their association with hydroxyapatite has a stabilising effect until temperatures reach ≥500°C (at which point collagen gelatinises).
There probably exists a relatively symbiotic dependency between bone collagen and bioapatite (Dobberstein et al. Reference Dobberstein, Collins, Craig, Taylor, Penkman and Ritz-Timme2009), the nature of which is not of immediate concern to the current research, other than to note that it has been shown to occur at both the macro- and microscopic levels (Vassalo et al. Reference Vassalo, Mamede, Ferreira, Cunha and Gonçalves2019). Importantly, Koon et al. (Reference Koon, Loreille, Covington, Christensen, Parsons and Collins2008) propose that collagen exhibits an ‘all or nothing’ mechanism, and that, regardless of its association with hydroxyapatite, collagen in mammalian bone will not undergo extensive thermally induced diagenetic alteration until exposed to sufficiently high temperatures (300–500°C; Holden et al. Reference Holden, Phakey and Clement1995; Etok et al. Reference Etok2007; Munro et al. Reference Munro, Longstaffe and White2007; Koon et al. Reference Koon, Loreille, Covington, Christensen, Parsons and Collins2008).
Materials and methods
Rib sections from 152 of the Herculaneum individuals were selected from six of the 12 fornici, and were prepared for collagen extraction using a modified Longin method (see Craig et al. Reference Craig2009). For comparative analysis, ribs were obtained from four individuals from the second-century AD Roman necropolis at Velia, a site at which previous analysis of collagen has been conducted (Craig et al. Reference Craig2009). The Velia samples were from formally buried, fully articulated individuals exhibiting no evidence of exposure to heat. Several animals, including dogs and ovicaprids, were discovered within the Herculaneum fornici and were analysed for further comparison.
In brief, samples were cleaned using a sterile scalpel and crushed into fragments of approximately 2–5mm in length. Sixty milligrams of each sample was weighed into a vial and demineralised in 0.6M HCl, before being gelatinised and lyophilised. Extracted collagen was weighed, and percentage weight calculated for each sample. Collagen yield is commonly expressed as a percentage of the bone's overall mass (for full details of the method, see Martyn et al. (Reference Martyn, Garnsey, Fattore, Petrone, Sperduti, Bondioli and Craig2018)). Following cleaning, the remaining bone samples were crushed into a granular powder using a pestle and mortar, prior to analysis by Fourier transform infrared spectroscopy-attenuated total reflectance (FTIR-ATR; for a detailed protocol, see Thompson et al. Reference Thompson, Islam and Bonniere2013, Reference Thompson, Szigeti, Gowland and Witcher2016). The scans were performed using a Nicolet 5700 FTIR spectrometer controlled by OMNIC 7.3 software. Before each bone sample was analysed, the diamond stage was cleaned with propanol and background spectra were collected. Three replicate measurements were taken for each sample and averages were used in subsequent analysis. The spectra were then recorded between 2000cm−1 and 400cm−1, at a resolution of 4cm−1. The following crystallinity measures proposed by Thompson et al. (Reference Thompson, Islam and Bonniere2013) were used in this study:
Crystallinity index (CI) = (565cm−1 + 605cm−1) / 595cm−1
Carbon / phosphate ratio (C/P) = 1415cm−1 / 1035cm−1
Carbonate / phosphate ratio (CO/P) = 1650cm−1 / 1035cm−1
Carbonate / carbonate ratio (CO/CO3) = 1650cm−1 / 1415cm−1
Carbonate / phosphate ratio (CO3/P) = 900cm−1 / 1035cm−1
Phosphate high temperature (PHT) = 625cm−1 / 610cm−1
Line width = the full width at half maximum of the phosphate peak at 1035cm−1
The numbers in these measures relate to positions on the FTIR spectra, and the ratios themselves examine different aspects of heat-induced change as influenced by burning conditions (Thompson et al. Reference Thompson, Islam and Bonniere2013, Reference Thompson, Szigeti, Gowland and Witcher2016). Therefore, the use of all seven indices allows for the analysis of a range of possible burning intensities: C/P, CO/CO3, CO3/P, CO/P and line-width best describe low-temperature burning. CI and line-width work best for describing middle intensity, while PHT and C/P best describe high-intensity burning events.
Results
Collagen yield
Of the 152 individuals analysed, only 12 (8 per cent) exhibit percentage collagen yields that fall below the 1 per cent threshold indicative of structural and chemical deterioration (DeNiro Reference DeNiro1985; van Klinken Reference van Klinken1999; Dobberstein et al. Reference Dobberstein, Collins, Craig, Taylor, Penkman and Ritz-Timme2009). Of these 12 individuals, seven are identified as infant or juvenile. Ten of the 12 were deposited in fornice 9 and two in fornice 8. Table 1 presents the average collagen yields for the individuals from the six fornici.
Table 1. Summary of collagen yields.

A two-sided independent Kruskall-Wallis test was applied to all 152 samples to identify statistical significance between percentage collagen yield and each fornice. Results showed that the distribution in collagen yields is significantly different between the chambers (H = 56.157, P = 0.000; Table 1). The highest average percentage yield is exhibited by the individuals from fornice 10 (average yield = 13.3 per cent, mean rank = 103.11), which also sheltered the greatest number of victims (43), and had the highest estimated total body mass based on the numbers of infants, juveniles, men and women present (Figure 1). The lowest average percentage yield is exhibited in fornice 7 (average yield = 3.8 per cent; mean rank = 40.68), which correlates with the lowest number of victims and the lowest total body mass. This trend is visible across the fornici populations, demonstrating a decrease in average percentage yield as the total population within each fornice also decreases (Table 1; Figure 5). An exception to this trend is presented in fornice 9, which exhibits the second largest population (37), but fifth lowest average (average yield = 4.1 per cent; mean rank = 39.58).

Figure 5. Notched box plots showing the influence of body mass in each fornici (packing density) on collagen yield. The notches indicates the confidence interval around the median (shown), if the notches do not overlap there is ‘strong evidence’ (95 per cent confidence) that their medians differ. Total body mass is estimated from the number, age and sex of individuals in each fornici using the corresponding mean body mass for modern Europeans (source: Food and Agriculture Organization of the United Nations: http://www.fao.org/home/en) (figure by the authors).
In addition, δ13C and δ15N isotope data obtained for a subset of 81 of the 152 samples were checked for C:N ratios, which fall within the acceptable ranges indicative of diagenetically unaltered collagen. These ranges are 2.9–3.6 and 3.1–3.5, as proposed by DeNiro (Reference DeNiro1985) and van Klinken (Reference van Klinken1999), respectively. All 81 samples fall within these ranges, suggesting that their structure and chemical quality are not compromised by diagenetic interference.
Crystalline structure
Figure 6 presents the combined CI and C/P data, an effective method of highlighting burning intensity. Comparative baseline C/P and CI values for bones exposed to low-, medium- and high-intensity burning are presented, collected in accordance with the same methodology (for method and summary data, see Thompson et al. Reference Thompson, Szigeti, Gowland and Witcher2016).

Figure 6. Comparison of crystallinity measurements from the Herculaneum fornici (grey) with controlled experiment burnings (coloured); bone samples from Velia are also shown (n = 4, black) (figure by the authors).
CI values ranged between 2.30 and 4.33, which corresponds with low-intensity burning events. This range has been verified through calibration with controlled experimental studies, but also other work on archaeological remains (Figure 6). The other crystallinity measures also support this temperature range, with the C/P and CI values coinciding with those exhibited by the low-intensity experimental samples (see Table S1 in the online supplementary data (OSM)).
Discussion
Overall, good collagen preservation and crystallinity values falling within the range indicative of no to low-intensity burning events suggest that the Herculaneum victims within the fornici were not exposed to significantly high temperatures at the time of death. This means either that previous estimates for the flow temperature are too high, or that other mechanisms buffered the victims’ skeletons from exposure to the full thermal energy of the pyroclastic surge. Several palaeomagnetic analyses undertaken at Herculaneum and contemporaneous sites estimate the temperature of the pyroclastic event to have been approximately 350–400°C (Kent et al. Reference Kent, Ninkovich, Pescatore and Sparks1981; Capasso Reference Capasso2000; Zanella et al. Reference Zanella2000; Cioni et al. Reference Cioni, Gurioli, Lanza and Zanella2004), although Mastrolorenzo et al. (Reference Mastrolorenzo, Petrone, Pagano, Incoronato, Baxter, Canzanella and Fattore2001) calculated 480°C immediately outside the chambers. Others have investigated the exposure of mortars, plasters and wood to the pyroclastic temperatures at different parts of the site (e.g. Caricchi et al. Reference Caricchi, Vona, Corrado, Giordano and Romano2014; Leone et al. Reference Leone, Vita, Magnani and Rossi2016). Their estimates (240–370°C) may suggest an even lower exposure temperature, assuming that it could indeed apply to the beach and fornici areas, as well as within the town itself. Furthermore, recent work by Giordano et al. (Reference Giordano2018) examined the influence of building structure and material on the temperatures of the pyroclastic flows, concluding that internal thermal disequilibrium was apparent. This means that temperatures could be 100°C lower when walls or building material were mixed up in the flow, when compared to locations where there was no building material present. Regardless, in either situation, the highest temperature noted was 440°C (Giordana et al. Reference Giordano2018).
An alternative hypothesis is therefore required to explain why the victims at Herculaneum were exposed to temperatures significantly lower than the pyroclastic movement. Drawing upon various lines of evidence, one suggestion is the presence of soft tissue at the time of, and following, impact. The data show a correlation between greater collagen and apatite preservation, and the number of individuals within each fornice. This suggests that soft tissue density played an important role in the preservation of these substrates. In accordance with the Flory-Rehner theory, van der Sman (Reference van der Sman2007) proposes that a higher density of soft tissue holds the potential for greater thermal protection of bone. When tissue is heated, a temperature gradient forms due to a delay in the transfer of energy from the exposed outer surface to the inner portions. Indeed, if the period of exposure is sufficiently short, such internal temperatures may not be experienced at all. At Herculaneum, the heating of the cadavers through pyroclastic exposure probably caused the outer tissues (dermis, muscles and tendons) to swell. The movement of water into the central portion of the cadaver (i.e. the thoracic area, interfaces (joints) with long bones in the extremities, and the pelvic region) may have served to bake, rather than burn, the skeleton.
Such a theory is partially supported by the occasional presence of the pugilistic attitude in some individuals—a position caused by dehydration and contraction of the muscles and other soft tissues of the body (Bohnert et al. Reference Bohnert, Rost and Pollack1998; Keough et al. Reference Keough, L'Abbé, Steyn and Pretorius2015; Ubelaker Reference Ubelaker and Thompson2015)—which suggests that not all victims were vaporised. These results are particularly interesting when compared to similar research on bones from other small, enclosed chambers. Salesse et al.’s (Reference Salesse, Dufour, Lebon, Wurster, Castex, Bruzek and Zazzo2014) work in the catacombs of Marcellinus and Peter in Rome, for example, shows considerable variation in collagen levels and high levels of crystallisation, and thus these seem to be greatly influenced by local environmental conditions. It has been argued that once burned, bones display limited subsequent diagenetic change (Snoeck et al. Reference Snoeck, Lee-Thorp and Schulting2014). Correlation between collagen yield/CI and fornice population sizes/total body mass at Herculaneum may demonstrate this relationship between density and tissue thermal buffering. Controlled cremation studies demonstrate that it takes between 40 minutes and two hours at temperatures in excess of 650°C (averaging 800–1000°C) to destroy cadaveric soft tissue fully (Bohnert et al. Reference Bohnert, Rost and Pollack1998; Gerling et al. Reference Gerling, Meissner, Reiter and Oehmichen2001; Schwark et al. Reference Schwark, Heinrich, Preuße-Prange and Wurmb-Schwark2011). Even when subjected to temperatures in excess of 1000°C, human tissue will not simply vaporise, as proposed by Mastrolorenzo et al. (Reference Mastrolorenzo, Petrone, Pagano, Incoronato, Baxter, Canzanella and Fattore2001, Reference Mastrolorenzo, Petrone, Pappalardo and Guarino2010), Petrone (Reference Petrone2011) and Petrone et al. (Reference Petrone2018). Evidence suggests that it is often the case with victims of fire that the side or portion of the body upon which they lie during burning remains relatively less degraded than areas undergoing direct exposure (Gerling et al. Reference Gerling, Meissner, Reiter and Oehmichen2001). In contrast with the majority of experimental investigations that burn single cadavers at a time, the Herculaneum victims died in close proximity, with bodies sometimes overlapping.
According to Gerling et al. (Reference Gerling, Meissner, Reiter and Oehmichen2001), the Herculaneum individuals within the fornici would have been even less susceptible to soft-tissue damage than those observed in controlled experiments. Indeed, the very nature of the thermal energy differs between the two scenarios. Organic matter acts as fuel when exposed to fire. This is further exacerbated by clothing, which can cause a ‘wick’ effect (DeHaan & Nurbakhsh Reference DeHaan and Nurbakhash2001; Christensen Reference Christensen2002) that often accelerates the burning process. Pyroclastic surges create radiant heat that does not utilise fuel. It is generally accepted that bodies exposed to radiant heat will exhibit significantly slower soft tissue consumption compared with bodies subjected to fire (Bohnert et al. Reference Bohnert, Rost and Pollack1998; Christensen Reference Christensen2002). This would have been compounded by the intact walls of the fornici, which, as Giordana et al.’s (Reference Giordano2018) work shows, would have slowed the process of thermal equilibrium with the external environment. Experimental work by Carroll and Smith (Reference Carroll and Smith2018) also suggests that bodies burning within enclosed spaces exhibit less significant heat-induced changes compared to those from pyre contexts. Further, analysis by Ellingham et al. (Reference Ellingham, Thompson and Islam2016) explicitly highlights the significant ‘buffering’ effect that soft tissue can have on heat-induced changes to bone crystallinity at temperatures below 300°C, and a ‘fuelling’ effect (i.e. accelerating combustion) above 800°C.
When interpreting crystallinity values, it is important to note that the relationship between the CI and burning intensity is not linear. The increase in CI, for example, is slow until around 500°C is reached, at which point it increases rapidly. In some cases, it has been argued that increases in CI due to low-intensity burning are the result of normal diagenesis and decomposition (Thompson Reference Thompson and Thompson2015a & b; Thompson et al. Reference Thompson, Islam and Bonniere2013, Reference Thompson, Szigeti, Gowland and Witcher2016). Notably, the nearby site of Velia, which suffered no such thermal incident but contains contemporaneous interred individuals, exhibits comparable CI values resulting from natural diagenesis (Figure 6). Due to the detailed contextual information we have for the eruption, the known history of Herculaneum and the presence of other heat-induced changes identified through osteological analysis, it is apparent that, in this instance, the observed changes can be confidently attributed to low-intensity heat exposure, rather than natural diagenesis. As noted above, subsequent diagenetic change to these heat-altered bones is unlikely.
Conclusions
The new data presented here, combined with a synthesis of results from studies that have examined Herculaneum's building and artefactual material, suggest that instantaneous soft tissue vaporisation and displacement with ash (Mastrolorenzo et al. Reference Mastrolorenzo, Petrone, Pagano, Incoronato, Baxter, Canzanella and Fattore2001, Reference Mastrolorenzo, Petrone, Pappalardo and Guarino2010; Petrone Reference Petrone2011; Petrone et al. Reference Petrone2018) would probably not have been possible under the conditions within the fornici. Based on the data presented and the macro- and microscopic osteological observations by Schmidt et al. (Reference Schmidt, Oakley, D'Anastasio, Brower, Remy, Viciano, Schmidt and Symes2015), we propose that the population density of each fornice affected collagen preservation directly through variability in soft-tissue ‘buffering’. The manner in which the victims of Vesuvius died at Herculaneum is tragic when approached from any perspective, but we conclude here that instant soft tissue vaporisation within the fornici is not concordant with the observed biochemical and osteological evidence presently available. Although the victims recovered from the beach were not part of our study sample, we suggest that instant vaporisation did not occur there either, due to some of the same reasons set out above.
The results presented here underline the importance of conducting specific FTIR and diagenetic analyses on material from contexts that have experienced events involving significant heat. Archaeological sites need to be assessed on an individual basis, taking into account the unique characteristics of the heating event and considering the importance of building integrity and enclosed spaces on the temperature experienced by the victims (Carroll & Smith Reference Carroll and Smith2018; Giordana et al. Reference Giordano2018). Other research at Herculaneum has demonstrated that good preservation of non-collagen organic material is also possible (Rowan Reference Rowan2017), thereby emphasising that organic residues can survive extremely challenging environments, and that analysis of these residues can allow for reinterpretation of past events.
Supplementary material
To view supplementary material for this article, please visit https://doi.org/10.15184/aqy.2019.215