Ageing is accompanied by a decline in muscle mass and function, termed sarcopenia( Reference Rosenberg 1 ). Sarcopenia increases the risk for falls and fractures, dependence, morbidity and mortality( Reference Janssen 2 ). The underlying cause of sarcopenia is multifactorial and complex in nature. Contributing factors include, but are not limited to, reduced physical activity levels, poor diet, chronic low-grade systemic inflammation, elevated levels of oxidative stress, mitochondrial dysfunction and hormonal changes( Reference Morley 3 – Reference Dickinson, Volpi and Rasmussen 5 ). Sarcopenia imposes significant burden on healthcare systems. In 2000, the estimated annual healthcare cost of sarcopenia in the USA reached $18·5 billion, representing 1·5 % of total healthcare expenditures for that year( Reference Morley, Anker and von Haehling 6 ). In order to treat or prevent sarcopenia, nutritional strategies must be developed to help increase or maintain skeletal muscle mass with advancing age.
Skeletal muscle mass is regulated by the balance between muscle protein synthesis and muscle protein breakdown( Reference Burd, Tang and Moore 7 ). Loss of muscle mass results from a negative net muscle protein balance, i.e. when muscle protein breakdown exceeds muscle protein synthesis over a given period of time. Muscle protein synthesis and muscle protein breakdown are concurrent and constant processes that are highly responsive to physical activity and protein intake( Reference Koopman and van Loon 8 ). Muscle protein synthesis is more responsive to both stimuli than muscle protein breakdown( Reference Biolo, Tipton and Klein 9 ). Thus, changes in muscle protein synthesis are primarily responsible for changes in muscle mass in response to exercise and nutrition, at least in healthy individuals( Reference Yang, Churchward-Venne and Burd 10 ). Dietary protein provides amino acids that can be used as precursors (i.e. building blocks) for muscle protein synthesis. Moreover, the essential amino acid leucine is not only a building block for muscle protein synthesis, it acts as a signalling molecule that can directly activate the muscle protein synthetic machinery. Several studies have compared the postprandial muscle protein synthetic response to protein intake between young and older individuals( Reference Brook, Wilkinson and Phillips 11 , Reference Shad, Thompson and Breen 12 ). Some studies observed lower postprandial muscle protein synthesis rates in older adults compared with the young, which has resulted in a concept termed anabolic resistance( Reference Burd, Gorissen and van Loon 13 ). Not all studies have been able to detect anabolic resistance, which might be related to the limited number of participants who are generally included in these financially expensive tracer studies( Reference Wall, Gorissen and Pennings 14 ). However, a more comprehensive evaluation of the muscle protein synthetic response to protein intake between young and older individuals was recently conducted by Wall et al. ( Reference Wall, Gorissen and Pennings 14 ), whereby data were pooled from multiple studies conducted within the same laboratory using an almost identical study design. Study findings revealed a markedly reduced muscle protein synthetic response to the ingestion of a single meal-like 20 g bolus of casein in older compared with young adults( Reference Wall, Gorissen and Pennings 14 ). These comprehensive data support the existence of anabolic resistance with ageing.
The aetiology of anabolic resistance with ageing is not entirely understood, but is proposed to be mediated by impairments in several physiological processes( Reference Burd, Gorissen and van Loon 13 ). A reduced rate of dietary protein digestion and amino acid absorption and/or a greater splanchnic amino acid retention may limit the postprandial availability of amino acids for muscle protein synthesis( Reference Boirie, Gachon and Beaufrere 15 , Reference Volpi, Mittendorfer and Wolf 16 ). In addition, a decline in insulin-mediated capillary recruitment, muscle tissue perfusion and the abundance or functionality of amino acid transporters may limit the delivery of amino acids to the muscle and the uptake of amino acids by the muscle( Reference Dickinson, Drummond and Coben 17 – Reference Rasmussen, Fujita and Wolfe 19 ). At the molecular level, an impaired activation of mechanistic target of rapamycin complex 1 and downstream signalling (e.g. p70S6 kinase, 4E-BP1) that regulates muscle protein synthesis also may contribute to anabolic resistance with ageing( Reference Cuthbertson, Smith and Babraj 20 , Reference Guillet, Prod'homme and Balage 21 ). Understanding the relative contribution of these processes to anabolic esistance with ageing is of critical importance for the design of effective nutritional strategies for combatting sarcopenia. Several factors are known to influence the muscle protein synthetic response to protein ingestion, most notably the quantity of protein consumed on a meal-by-meal basis( Reference Churchward-Venne, Holwerda and Phillips 22 ). In addition, the quality (i.e. source) of ingested protein has been shown to modulate the postprandial muscle protein synthetic response( Reference Gorissen, Remond and van Loon 23 ). Accordingly, the primary focus of this review is to compare the muscle anabolic capacity of animal-derived (dairy and meat-based) proteins with various plant-based proteins in older adults.
Anabolic properties of animal-derived protein sources
Several studies have demonstrated that animal-derived protein sources such as dairy (e.g. milk and eggs) and meat (e.g. beef) elicit a robust stimulation of muscle protein synthesis in older adults( Reference Gorissen, Remond and van Loon 23 ). However, not all animal-based protein sources are comparable in terms of anabolic properties that determine the amplitude and duration of the postprandial muscle protein synthetic response. For example, whey protein is characterised as a fast protein based on its rapid protein digestion and amino acid absorption kinetics, whereas casein clots in the stomach and is slowly digested and absorbed( Reference Boirie, Dangin and Gachon 24 , Reference Dangin, Boirie and Garcia-Rodenas 25 ). The ingestion of 20 g fast digestible whey protein, that is particularly high in leucine content, has been shown to stimulate muscle protein synthesis to a greater extent compared with a matched dose of slowly digestible micellar casein in older men( Reference Burd, Yang and Moore 26 ). These findings are consistent with similar studies in young adults( Reference Tang, Moore and Kujbida 27 ) and highlight the importance of a rapid rise in blood leucine concentrations for stimulating a robust increase in muscle protein synthesis( Reference West, Burd and Coffey 28 ).
Although whey protein has consistently been shown to elicit a robust stimulation of muscle protein synthesis, whey protein represents a fraction of milk and is commonly co-ingested with casein( Reference Mitchell, McGregor and D'Souza 29 ). Accordingly, a recent study assessed the postprandial muscle protein synthetic response to ingesting 20 g whey protein compared with a milk protein concentrate composed of both whey protein and casein( Reference Mitchell, McGregor and D'Souza 29 ). Study findings revealed a more rapid appearance of circulating amino acids after whey protein ingestion; however there was no difference in the postprandial muscle protein synthetic response between whey protein and milk protein concentrate in middle-aged men. In terms of comparing the anabolic potential of animal-derived protein-rich foods, we recently assessed postprandial protein handling and the subsequent muscle protein synthetic response to the ingestion of 350 ml fluid skimmed milk compared with 160 g cooked lean minced beef (both providing 30 g protein) during recovery from resistance exercise in young men( Reference Burd, Gorissen and van Vliet 30 ). Beef was more rapidly digested and absorbed, which resulted in a greater rise in plasma amino acid availability and higher peak plasma leucine concentrations. Skimmed milk ingestion resulted in a moderate but rapid rise in circulating plasma leucine and stimulated muscle protein synthesis to a greater extent during the early 0–2 h recovery period than beef. Taken together, these data suggest that milk is equally effective as whey protein and superior to beef with regard to stimulating muscle protein synthesis.
Food matrix and texture may represent another important factor that modulates the muscle protein synthetic response to protein ingestion in older adults( Reference Gorissen, Remond and van Loon 23 ). A recent study demonstrated that minced beef is more rapidly digested and absorbed than beef steak, resulting in a greater availability of protein-derived amino acids in the circulation and a more positive whole-body net protein balance after the ingestion of minced beef compared with beef steak( Reference Pennings, Groen and van Dijk 31 ). The 135 g portion of beef administered in this study provided about 20 g protein, which was unable to stimulate muscle protein synthesis. Consistent with this observation, a previous dose–response study demonstrated that 113 g minced beef (24 g protein) was not sufficient to stimulate muscle protein synthesis under both rested and post-exercise conditions in middle-aged men( Reference Robinson, Burd and Breen 32 ). Instead, 170 g beef, providing 36 g protein, was required to stimulate muscle protein synthesis. Taken together, these data suggest that minced beef may be more effective in stimulating muscle protein synthesis compared with beef steak at higher doses of protein intake.
Global differences in food sources constituting daily protein intake
To date, most studies in older adults that have assessed the postprandial muscle protein synthetic response to protein intake have administered an animal-derived protein source( Reference Burd, Yang and Moore 26 , Reference Pennings, Groen and van Dijk 31 , Reference Koopman, Crombach and Gijsen 33 – Reference Yang, Breen and Burd 37 ). Relatively few studies have characterised the muscle protein synthetic response to ingestion of a plant-based protein source( Reference Yang, Churchward-Venne and Burd 10 , Reference Gorissen, Horstman and Franssen 38 , Reference Phillips 39 ). This gap in knowledge may be considered surprising given that a greater variety of plant-based protein-rich foods are readily available compared with animal-derived protein foods and most dietary protein consumed worldwide is in fact derived from plant (60 %) rather than animal (40 %) sources (Table 1)( 40 ). An estimated 4 billion people live primarily on a plant-based diet, while an estimated 2 billion people worldwide live primarily on a meat-based diet( Reference Pimentel and Pimentel 41 ). Of the plant-based food sources, cereals provide the greatest contribution, responsible for approximately 65 % of plant protein intake and 40 % of total protein intake. Pulses, nuts, seeds and vegetables provide a moderate contribution (2·2–10·4 g/d) to daily protein intake, whereas lower amounts of protein are provided by potatoes and fruit (about 3 g/d). Importantly, the contribution of plant and animal-based protein sources to total daily protein intake is specific to the continent or country of interest. In Africa and Asia, plant-based foods provide 77 and 66 % of total protein intake, respectively, whereas the contribution of animal-derived food sources to total dietary protein intake is greater in the USA (56 %), Europe (57 %) and Oceania (65 %). Across the USA, Europe and Oceania, meat and dairy sources provide the greatest contribution (about 80 %) to daily animal protein intake, whereas as little as 7 g meat and 4 g milk is consumed per capita per day in Africa. It may be argued that future research designed to assess the muscle protein synthetic response to an ingested protein source should focus on the most commonly consumed protein source in any given country.
Table 1. Sources of dietary protein intake

Data are expressed as g protein per capita per d. Food sources shown in table provide at least 96 % of daily protein intake. Data are derived from Statistics Division of FAO of the UN, Food Balance Sheets 2013( 40 ).
Sustainability of commonly consumed dietary protein sources
A more advanced understanding of the anabolic potential of various plant-based protein sources also may be considered critical given concerns regarding the global sustainability of animal-based protein diets. A sustainable diet may be defined as ‘a diet with low environmental impact that contributes to food and nutrition security and to healthy life for present and future generations’( Reference Macdiarmid 42 ). The food supply chain accounts for about 20 % of all annual greenhouse gas emissions attributed to the UK( Reference Berners-Lee, Hoolohan and Cammack 43 ). In the UK, the consumption of animal-derived protein foods is increasing at a rate 2-fold greater than plant-based protein foods( Reference Millward and Garnett 44 ). Diets that primarily contain animal-derived food sources (e.g. meat and dairy products) are associated with high greenhouse gas emissions (>4 kg carbon dioxide equivalents (CO2e)/kg edible weight; Fig. 1)( Reference Macdiarmid 42 ). According to recent UK estimates, the production and consumption of beef (about 70 kg CO2e/kg) and pork (8 kg CO2e/kg edible weight) contributes most of all food sources to greenhouse gas emissions( Reference Scarborough, Appleby and Mizdrak 45 ). Interestingly, unlike most other dairy products (e.g. cheese and eggs), milk is associated with only moderate greenhouse gas emissions. The production and consumption of most plant-based protein foods, including wheat, oat and potato are associated with low greenhouse gas emissions (<1 kg CO2e/kg edible weight)( Reference Macdiarmid 42 ). Notable exceptions include rice (4 kg CO2e/kg edible weight) and to a lesser extent soya (2 kg CO2e/kg edible weight)( Reference Scarborough, Appleby and Mizdrak 45 ). Therefore, on a gram-for-gram basis, commonly consumed plant-based protein-rich foods are, for the most part, considered to be more environmentally sustainable compared with animal-derived proteins, especially from meat sources( Reference Pimentel and Pimentel 41 ). However, as a note of caution, it is widely appreciated that recommendations for improving protein-based food choices to reduce greenhouse gas emissions must be balanced against protein recommendations for improving health( Reference Macdiarmid 42 , Reference Millward and Garnett 44 ), of which the maintenance of muscle mass becomes increasingly important with advancing age( Reference Wolfe 46 ). It follows that a critically important topic in the field of protein nutrition for healthy musculoskeletal ageing includes comparing the anabolic capacity of plant and animal-based proteins for combatting sarcopenia.

Fig. 1. Estimated greenhouse gas (GHG) emissions (kg CO2e/kg edible weight) for the most common animal-based (grey bars) and plant-based (white bars) dietary protein sources. Low GHG emissions, <1·0 kg CO2e/kg edible weight; Medium GHG emissions, 1·0–4·0 kg CO2e/kg edible weight; High GHG emissions, >4·0 kg CO2e/kg edible weight; CO2e, carbon dioxide equivalent. Estimated values are based on UK data derived from Scarborough et al. ( Reference Scarborough, Appleby and Mizdrak 45 ).
Anabolic properties of plant-based protein sources
Soya protein is one of the few plant-based proteins that has been studied for its muscle anabolic potential in human subjects. Wilkinson et al. ( Reference Wilkinson, Tarnopolsky and Macdonald 47 ) compared the anabolic response to consuming soya and milk within a mixed macronutrient beverage containing 18 g protein. The consumption of milk after a single bout of resistance exercise increased postprandial muscle protein synthesis rates to a greater extent compared with the ingestion of soya in healthy young men( Reference Wilkinson, Tarnopolsky and Macdonald 47 ). The authors speculated that due to the more rapid digestion of soya protein and therefore faster and greater delivery of amino acids from the gut to the liver, more of the soya protein-derived amino acids were directed towards urea production and serum protein synthesis( Reference Bos, Metges and Gaudichon 48 ), rather than muscle protein synthesis( Reference Wilkinson, Tarnopolsky and Macdonald 47 ). Milk ingestion resulted in a moderate but sustained rise in plasma amino acid concentrations and subsequently a more prolonged positive net protein balance across the leg( Reference Wilkinson, Tarnopolsky and Macdonald 47 ). Consistent with this observation, the chronic consumption of milk immediately and 1 h after each exercise session (providing 35 g protein) during 12 weeks resistance training resulted in greater gains in lean body mass when compared with the consumption of isonitrogenous amounts of soya( Reference Hartman, Tang and Wilkinson 49 ). Thus, milk has been shown to be more effective in stimulating muscle protein synthesis compared with a soya-based protein beverage in resistance trained young men.
The postprandial muscle protein synthetic response to the ingestion of isolated soya protein also has been compared with the constituent milk proteins, whey and casein, in young men under resting conditions( Reference Tang, Moore and Kujbida 27 ). The ingestion of soya protein stimulated muscle protein synthesis to a greater extent when compared with casein, but the postprandial muscle protein synthetic response to soya protein tended to be lower when compared with whey protein. This divergent muscle protein synthetic response was likely attributed to differences in protein digestion kinetics and leucine content. Whey protein is rapidly digested and exhibits a high leucine content (2·3 g), whereas soya protein is rapidly digested but exhibits a lower leucine content (1·8 g) and casein is slowly digested and exhibits a lower leucine content (1·8 g). As such, the ingestion of whey protein, soya protein and casein results in a high, medium and low rise in plasma leucine concentrations, respectively, which is reflected by the magnitude of the postprandial increase in muscle protein synthesis rates( Reference Tang, Moore and Kujbida 27 ). Studies in older individuals have shown that postprandial muscle protein synthesis rates after the ingestion of 24 g soya protein are lower when compared with an equal amount of protein provided by beef( Reference Phillips 39 ). Consistent with this observation, the muscle protein synthetic response to graded intakes of soya protein also was shown to be lower when compared with whey protein in older adults( Reference Yang, Churchward-Venne and Burd 10 ). In fact, ingesting up to 40 g soya protein failed to substantially elevate muscle protein synthesis rates from basal, fasting rates( Reference Yang, Churchward-Venne and Burd 10 ). Thus, the ingestion of soya protein may stimulate muscle protein synthesis in young individuals, albeit to a lesser extent compared with some animal-derived proteins (e.g. whey). However, based on current evidence, soya protein intake is unable to stimulate muscle protein synthesis in older adults, at least at the doses (up to 40 g) investigated to date.
Wheat protein is the most abundant plant-based dietary protein source worldwide comprising approximately 20 % of total protein intake (Table 1)( 40 ). We recently assessed the anabolic properties of wheat protein when compared with casein and whey protein in healthy older men( Reference Gorissen, Horstman and Franssen 38 ). We provided 35 g whey, casein, or wheat protein containing 4·4, 3·2 or 2·5 g leucine, respectively, which is suggested to be sufficient to activate the muscle protein synthetic machinery( Reference Bauer, Biolo and Cederholm 50 ). The ingestion of whey protein increased postprandial plasma leucine concentrations to a greater extent compared with casein and wheat protein. The ingestion of casein and wheat protein resulted in a similar peak in plasma leucine concentrations, with a more sustained elevation of plasma leucine concentrations after casein ingestion. Interestingly, only the ingestion of casein stimulated muscle protein synthesis and the postprandial muscle protein synthetic response to the ingestion of wheat protein was significantly lower when compared with casein (Fig. 2). Ingesting a greater amount of wheat protein (i.e. 60 g), matched for the leucine content of whey protein, prolonged the postprandial increase in plasma leucine concentrations and increased muscle protein synthesis rates to a similar extent as casein( Reference Gorissen, Horstman and Franssen 38 ). These data demonstrate that a larger amount of wheat protein compared with casein is required to stimulate muscle protein synthesis in older men.
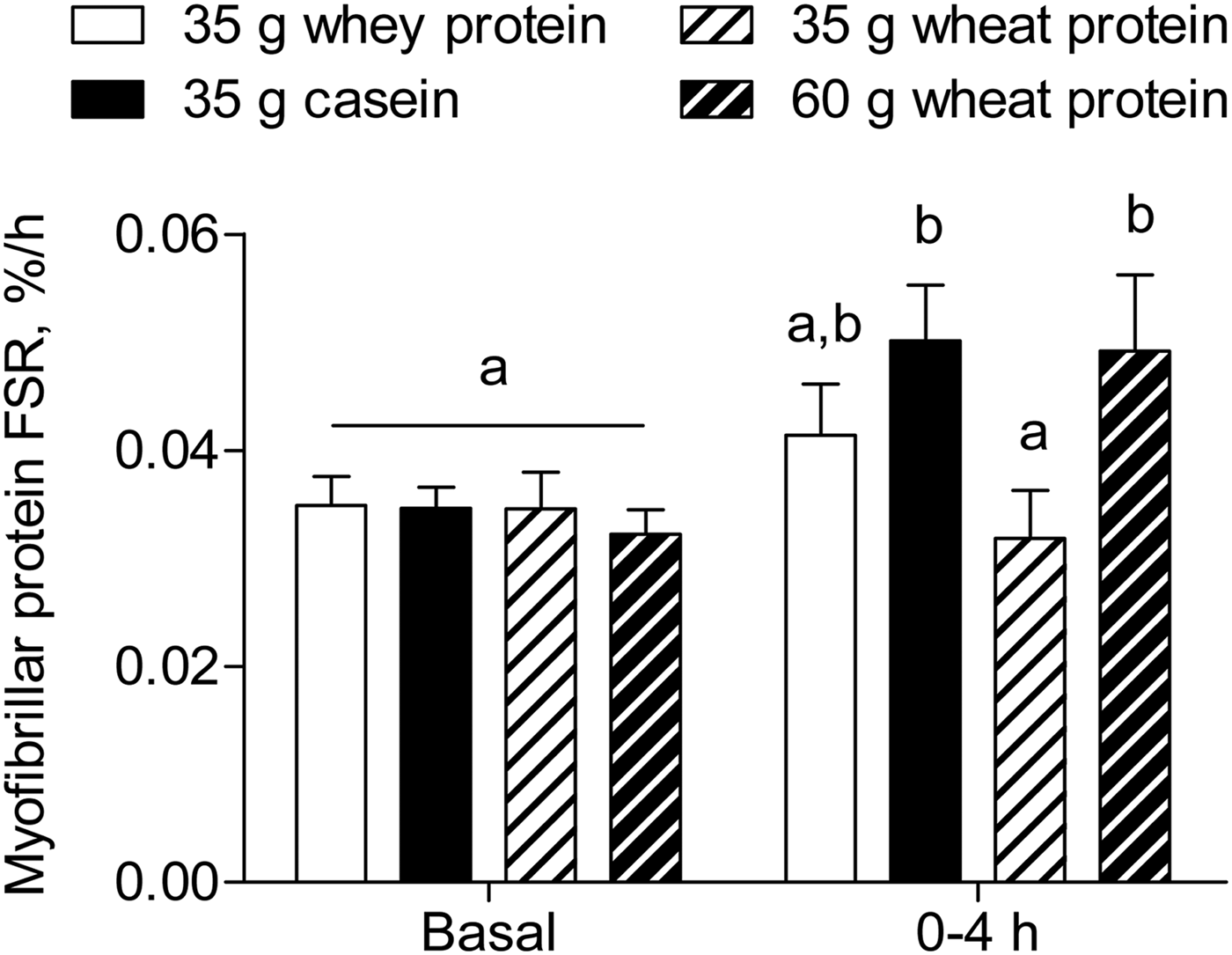
Fig. 2. Myofibrillar protein fractional synthetic rate (FSR, %/h) during the fasting state (Basal) and over the 4 h postprandial period after the ingestion of 35 g whey protein, 35 g casein, 35 g wheat protein, or 60 g wheat protein in healthy older men. Values are means ± sem, n 12/group. Labelled bars without a common letter differ, P < 0·05. Data are derived from Gorissen et al. ( Reference Gorissen, Horstman and Franssen 38 ).
The assumption that plant-based proteins exhibit inferior muscle anabolic potential compared with animal proteins is essentially based on data obtained from acute metabolic studies that assessed the postprandial muscle protein synthetic response to ingested soya and wheat protein( Reference Yang, Churchward-Venne and Burd 10 , Reference Tang, Moore and Kujbida 27 , Reference Gorissen, Horstman and Franssen 38 , Reference Phillips 39 , Reference Wilkinson, Tarnopolsky and Macdonald 47 , Reference Hartman, Tang and Wilkinson 49 ). An explanation for the reduced postprandial muscle protein synthetic response after the ingestion of soya or wheat may relate, at least in part, to the lower digestibility of these plant proteins compared with animal proteins. Animal-based protein sources such as dairy, meat and fish are highly digestible with digestibility scores exceeding 90 %. In contrast, plant-based protein sources such as rice, wheat, soya and potato exhibit lower digestibility scores ranging from 45 to 80 %( 51 ). As such, less of the dietary protein contained in a plant source is absorbed by the small intestine, resulting in a lower availability of dietary protein-derived amino acids for muscle protein synthesis. However, after removal of anti-nutritional factors that interfere with protein digestion and absorption, purified plant-based proteins are likely to exhibit digestibility scores similar to animal-derived proteins. Moreover, the essential amino acid composition of plant-based proteins may be suboptimal for the stimulation of muscle protein synthesis compared with animal-derived proteins. If an (essential) amino acid is limiting, protein synthesis is compromised and all other amino acids will be oxidised rather than utilised for protein synthesis( 52 ). Plant-based proteins generally have an inadequate lysine and/or methionine content (Table 2)( Reference van Vliet, Burd and van Loon 53 ). For example, wheat protein contains low amounts of lysine and methionine, both below the amino acid requirement as defined by the WHO/FAO/UNU( 52 ). Maize, rice and oat protein are low in lysine, whereas soyabean and pea protein are low in methionine. However, potato and quinoa protein contain sufficient amounts of all essential amino acids. As such, the assertion that all plant-based protein sources exhibit inferior muscle anabolic potential may be considered somewhat premature. Future studies are warranted to assess whether potato or quinoa protein have the capacity to stimulate muscle protein synthesis in older individuals.
Table 2. Amino acid composition of various plant-based and animal-derived proteins

Overcoming the perceived inferior anabolic properties of plant-based proteins
Dose of protein
Protein dose–response studies have been conducted to assess how much protein is required to maximally stimulate muscle protein synthesis. We recently assessed the postprandial muscle protein synthetic response to whey protein intakes ranging from 0 to 40 g in young men( Reference Witard, Jackman and Breen 54 ). The results indicated that a maximal stimulation of muscle protein synthesis was achieved after ingesting 20 g whey protein. A similar study in older men showed a dose–response relationship up to 40 g whey protein( Reference Yang, Breen and Burd 37 ), indicating that older individuals require a higher protein dose to maximally stimulate muscle protein synthesis. To date, only one study has assessed the postprandial muscle protein synthetic response to graded intakes of plant-based protein( Reference Yang, Churchward-Venne and Burd 10 ). The ingestion of up to 40 g soya protein (3·2 g leucine) was unable to induce a measurable increase in muscle protein synthesis rates under resting conditions in older individuals( Reference Yang, Churchward-Venne and Burd 10 ), suggesting that even greater amounts of plant-based protein are required to stimulate muscle protein synthesis in older adults. With a view to overcoming the inferior anabolic capacity of wheat protein, we recently provided older men with a 60 g bolus of wheat protein constituting 4·4 g leucine( Reference Gorissen, Horstman and Franssen 38 ). The ingestion of this high dose of wheat protein effectively stimulated muscle protein synthesis to a similar extent as dairy protein. Interestingly, ingesting the higher dose of wheat protein did not further increase the amplitude of peak plasma leucine concentrations compared with the lower 35 g dose of wheat protein, but prolonged the postprandial elevation in plasma leucine (and total essential amino acid) concentrations( Reference Gorissen, Horstman and Franssen 38 ). These data suggest that the kinetics of amino acid appearance in the blood rather than the absolute increase in leucine or essential amino acid concentrations determine the postprandial muscle protein synthetic response. Moreover, essential amino acids should be made available for muscle protein synthesis in older adults during the late postprandial period. Although effective, the ingestion of (very) high doses of plant-based protein may not represent a practical strategy for stimulating muscle protein synthesis in older adults with low appetite. In addition, it could be questioned whether plant-based proteins are still more environmentally sustainable when accounting for the greater dose of ingested protein necessary to maximally stimulate muscle protein synthesis. Therefore, more practical, cost-effective and sustainable strategies are discussed later.
Protein blends
The use of protein blends recently has received considerable attention as a possible strategy to overcome the inferior anabolic properties of plant-based proteins( Reference van Vliet, Burd and van Loon 53 ). Combining two or more plant-based protein sources may potentially overcome any deficiencies in a single essential amino acid that may be prevalent if a single plant protein is consumed. Plant-based proteins are generally low in lysine and/or methionine content when compared with animal-based proteins, which may compromise the postprandial muscle protein synthetic response( Reference van Vliet, Burd and van Loon 53 ). As described earlier, many plant-based proteins are low in either lysine or methionine, but contain ample amounts of the other essential amino acids (Table 2). Theoretically, combining a plant-based protein, which is low in lysine but high in methionine with a plant-based protein, which is low in methionine but high in lysine will result in a protein blend that contains sufficient amounts of all essential amino acids required for stimulating muscle protein synthesis. For example, maize, rice and oat protein are low in lysine but high in methionine, whereas soyabean and pea protein are low in methionine but high in lysine. Accordingly, we designed three protein blends that combine two plant-based proteins in a 50/50 ratio: maize/soyabean, rice/soyabean and rice/pea. The lysine and methionine contents of all the three blends exceed the amino acid requirement as defined by the WHO/FAO/UNU (4·5 and 1·6 %, respectively( 52 ); Fig. 3). Multiple other protein blends can be formulated that combine two or more complementary plant-based proteins at different ratios in order to provide sufficient amounts of all nine essential amino acids. However, it remains to be determined whether these protein blends have the capacity to stimulate muscle protein synthesis to a similar extent as dairy or meat-based proteins.
Alternatively, combining plant-based with animal-derived proteins may result in a protein blend that will capitalise on the unique digestive properties of each type of protein, allowing for an optimal blood availability of amino acids to increase the amplitude and duration of the postprandial muscle protein synthetic response. A series of studies have been conducted using a plant/animal protein blend composed of 50 % caseinate, 25 % whey protein and 25 % soya protein( Reference Borack, Reidy and Husaini 55 – Reference Reidy, Walker and Dickinson 58 ). In young adults, ingesting 19 g of this protein blend after a single bout of resistance exercise increased muscle protein synthesis rates and resulted in a more sustained elevation of muscle protein synthesis when compared with the ingestion of a leucine-matched amount of whey protein( Reference Reidy, Walker and Dickinson 58 ). In terms of translating these acute data in young adults to a chronic setting, dietary supplementation with this protein blend during 12 weeks resistance training tended to enhance gains in lean body mass compared with placebo in young men (2·9 v. 2·0 kg, respectively), whereas no further increase in lean body mass was observed after training with whey protein supplementation (2·3 kg)( Reference Reidy, Borack and Markofski 56 ). In older adults, ingesting a higher 30 g dose of the protein blend during recovery from resistance exercise failed to stimulate muscle protein synthesis above basal resting values, whereas whey protein ingestion did stimulate muscle protein synthesis during the early and entire post-exercise recovery period( Reference Borack, Reidy and Husaini 55 ). The absence of a measureable increase in muscle protein synthesis after ingesting the protein blend is surprising since it has consistently been shown that prior exercise sensitises skeletal muscle to the anabolic properties of dietary protein( Reference Burd, West and Moore 59 , Reference Pennings, Koopman and Beelen 60 ). It seems likely that the higher basal resting muscle protein synthesis rates in the protein blend condition precluded the ability to detect a significant stimulation of muscle protein synthesis. Nonetheless, absolute values of postprandial muscle protein synthesis rates after the ingestion of the protein blend were similar when compared with whey protein. These data suggest that a plant/animal protein blend can stimulate muscle protein synthesis to a similar extent as whey protein. However, the addition of a small amount of soya protein to milk protein does not substantially reduce dairy protein intake. Moreover, this protein blend does not seem to offer benefits regarding sustainability as milk is relatively environmentally sustainable compared with other animal-based protein sources. Future studies should identify other plant/animal protein blends with a higher plant-based protein content that could serve as a more sustainable dietary protein source to preserve muscle mass in the ageing population.
Leucine co-ingestion
Accumulating evidence suggests that the strongest independent predictor of muscle anabolic potential is the leucine content of the ingested protein source( Reference Phillips 61 ). In addition to providing substrate for the synthesis of new muscle protein, leucine acts as a key signal for activating the muscle protein synthetic machinery. The leucine content of animal proteins is typically 10 % or greater, whereas the leucine content of most plant proteins ranges from 6 to 8 %. An exception to this rule is maize protein, which constitutes approximately 12 % leucine. The leucine content can be modified simply by adding free leucine to the protein source. Two studies have demonstrated that adding free leucine (2·5 g) to a meal-like bolus of casein further increases the postprandial stimulation of muscle protein synthesis at rest( Reference Rieu, Balage and Sornet 62 , Reference Wall, Hamer and de Lange 63 ). In addition, 2 weeks of leucine supplementation has been shown to increase both post-absorptive and postprandial muscle protein synthesis rates( Reference Casperson, Sheffield-Moore and Hewlings 64 ). In contrast, the addition of leucine to leucine-rich whey protein failed to further increase the postprandial muscle protein synthetic response during post-exercise recovery( Reference Koopman, Wagenmakers and Manders 65 ). Moreover, long-term leucine supplementation did not improve muscle mass or strength in healthy older adults who consumed an adequate amount of protein within their diet (about 1·0 g/kg per d)( Reference Verhoeven, Vanschoonbeek and Verdijk 66 ). To our knowledge, no study to date has determined whether co-ingesting leucine with a plant-based, leucine-poor, protein source potentiates the postprandial muscle protein synthetic response at rest or following exercise in older adults. In addition, no study has examined the impact of supplementing a vegetarian diet with leucine on chronic changes in muscle mass in older adults. While limited data currently exist in human subjects( Reference Murphy, Saddler and Devries 67 ), a study in rodents demonstrated that fortification of wheat with leucine to match the leucine content of a whey protein meal resulted in a similar postprandial muscle protein synthetic response( Reference Norton, Wilson and Layman 68 ). Hence, it is intuitive that enriching lower leucine-containing plant-based proteins will enhance postprandial muscle protein synthesis rates in older adults.
Enhancing the muscle anabolic sensitivity to ingested protein
As an alternative approach to enhancing the anabolic capacity of plant-based proteins, increasing the sensitivity of skeletal muscle to anabolic stimuli may potentiate the postprandial muscle protein synthetic response after the ingestion of plant-based protein. The most potent approach to enhance the sensitivity of skeletal muscle is physical activity. Both resistance( Reference Pennings, Koopman and Beelen 60 ) and aerobic( Reference Timmerman, Dhanani and Glynn 69 ) exercise performed before protein intake have been shown to enhance the utilisation of protein-derived amino acids for de novo muscle protein synthesis in older adults. More recent attention has focused on the role of fish oil-derived n-3 PUFA for increasing the anabolic sensitivity of skeletal muscle to protein intake, with more encouraging results in older( Reference Smith, Atherton and Reeds 70 ) compared with young adults( Reference McGlory, Wardle and Macnaughton 71 ). In a proof-of-concept study, 8 weeks supplementation with a daily dose of 1·9 g EPA and 1·5 g DHA was shown to potentiate muscle protein synthesis rates in response to simulated feeding (i.e. a hyperaminoacidemic-hyperinsulinemic clamp) in middle-aged and older adults( Reference Smith, Atherton and Reeds 70 , Reference Smith, Atherton and Reeds 72 ). The enhanced muscle protein synthetic response to amino acid provision with n-3 PUFA supplementation was associated with an increased incorporation of n-3 PUFA into the muscle phospholipid membrane( Reference Smith, Atherton and Reeds 70 , Reference Smith, Atherton and Reeds 72 ) and an increased expression and phosphorylation of anabolic signalling proteins such as mechanistic target of rapamycin complex 1, protein kinase B and focal adhesion kinase( Reference Smith, Atherton and Reeds 70 , Reference Smith, Atherton and Reeds 72 , Reference McGlory, Galloway and Hamilton 73 ). Moreover, findings from a recent cell culture experiment suggests that EPA rather than DHA is the anabolically active ingredient of fish oil, both in terms of upregulating muscle protein synthesis and suppressing muscle protein breakdown( Reference Kamolrat and Gray 74 ). A next step for this research field is to investigate the role of n-3 PUFA supplementation in sensitising skeletal muscle to the ingestion of a plant-based protein source in older adults.
Does a plant-based diet support muscle mass maintenance?
Acute metabolic studies utilising stable isotope tracer methodology offer a powerful approach to qualitatively compare the anabolic response to the ingestion of an isolated dairy, meat, or plant-based protein source (primarily by measuring postprandial muscle protein synthesis rates)( Reference Wolfe, Chinkes and Wolfe 75 ). However, dietary protein is generally consumed as part of a meal providing proteins from various sources. As an alternative approach to acute tracer studies, several studies have utilised dual-energy X-ray absorptiometry, computed tomography or MRI techniques to assess chronic changes (over weeks or months) in skeletal muscle mass following consumption of meat-based v. plant-based diets( Reference Heymsfield, Adamek and Gonzalez 76 ). For example, Campbell et al. ( Reference Campbell, Barton and Cyr-Campbell 77 ) compared a mixed omnivorous diet (about 50 % protein from beef, pork, poultry and fish) with a lacto-ovo vegetarian diet and assessed changes in muscle mass over 12 weeks resistance training. Older men in the lacto-ovo vegetarian group did not gain fat free mass, whereas men in the omnivorous group gained 1·7 kg fat free mass on average over 12 weeks resistance training. However, the vegetarian diet provided less protein (0·8 g/kg per d) compared with the omnivorous diet (1·0 g/kg per d). When consuming 1·2 g protein/kg per d, resistance training-induced gains in muscle mass (midthigh cross-sectional area) did not differ between the vegetarian and the omnivorous diet in healthy older men( Reference Haub, Wells and Tarnopolsky 78 ). On a population level, cross-sectional studies showed that total protein and animal protein intake, but not plant protein intake, are positively associated with muscle mass index( Reference Aubertin-Leheudre and Adlercreutz 79 – Reference Maltais, Leblanc and Archambault-Therrien 81 ) and leg lean mass( Reference Sahni, Mangano and Hannan 82 ). In addition, longitudinal studies have shown that higher intakes of total protein and animal protein are associated with a reduced loss of lean mass over 3 years of follow-up( Reference Houston, Nicklas and Ding 83 , Reference Isanejad, Mursu and Sirola 84 ) and a reduced loss of grip strength over 6 years of follow-up( Reference McLean, Mangano and Hannan 85 ). The absence of a significant association between plant protein intake and changes in lean mass may be related to a smaller range of plant protein intakes compared with animal protein intakes and/or the inclusion of trunk lean mass measurements, which mainly includes organs rather than skeletal muscle. Interestingly, higher plant protein intake was significantly associated with a reduced loss of appendicular lean mass (including lean mass of arms and legs only) over 3 years of follow-up( Reference Isanejad, Mursu and Sirola 84 ). As an alternative approach to assessing the relationship between dietary protein source and muscle mass, Mangano et al. ( Reference Mangano, Sahni and Kiel 86 ) recently identified six food clusters each containing proteins from various sources but predominantly from (1) fast food, (2) red meat, (3) chicken, (4) fish, (5) milk or (6) legumes. Men and women in the legume group consumed high amounts of beans and peas, nuts and seeds, fruit and vegetables, and cereals, but not from red meat and relatively low amounts from dairy, chicken and fish. Participants in the legume food cluster had a lower appendicular lean mass compared with participants in the other food clusters. However, after adjusting for known confounding factors such as age, sex, BMI, physical activity level, smoking status and alcohol intake, no significant differences between food clusters were observed. Together, these data suggest that diets high in plant protein sources have the potential to support the maintenance of muscle mass with ageing provided that sufficient amounts of protein are consumed.
Conclusions
Total dietary protein intake plays a critical role in maintaining skeletal muscle mass with advancing age( Reference Witard, McGlory and Hamilton 87 ). The source of protein consumed may represent another factor that influences the preservation of muscle mass in older adults. Although a wide range of protein-rich foods are commonly consumed, scientific insight into the impact of chosen protein source on muscle protein synthesis is limited to the ingestion of milk proteins, beef and the plant-based proteins soya and wheat protein. To our knowledge, no study to date has characterised the postprandial muscle protein synthetic response to the consumption of egg, poultry, pork, fish, or plant-based proteins other than soya and wheat in older adults. Based on currently available evidence from studies in older adults, there is general consensus that on a gram-for-gram basis, the ingestion of animal-based protein sources such as dairy and meat are more potent in terms of stimulating muscle protein synthesis compared with plant-based proteins. This differential postprandial stimulation of muscle protein synthesis is likely attributed to differences in protein digestion and amino acid absorption kinetics, essential amino acid profile and leucine content between plant-based and animal-derived protein sources. However, this belief may be considered somewhat premature given that a comparison with plant-based protein sources is limited to soya and wheat proteins, while other plant-based proteins (e.g. maize or potato protein) may have a greater anabolic potential due to a favourable amino acid composition. From the standpoint of environmental sustainability and food security, plant-based protein-rich foods may be considered advantageous over animal-based protein-rich foods on a gram-for-gram basis, but not when taken into account the greater dose of protein that may be required to maximally stimulate muscle protein synthesis. Theoretical strategies to increase the anabolic potential of plant-based protein sources include fortifying plant-based proteins with leucine and consuming protein blends. Future work is warranted to develop and apply these strategies with a view to overcoming the inferior anabolic properties of plant-based proteins and help maintain muscle mass in older adults.
Acknowledgements
The authors would like to express their gratitude to Professor Kevin Tipton and Drs Chris McGlory and Daniel Traylor for their constructive feedback during the preparation of the manuscript.
Financial Support
None.
Conflicts of Interest
None.
Authorship
Both authors wrote and approved the manuscript.