Introduction
Tomato is one of the most important specialty crops worldwide and Florida is the leading producer of fresh-market tomatoes in the USA (USDA-NASS, 2018). Since the phase-out of the broad-spectrum fumigant methyl bromide, alternative chemical soil fumigants (CSF) are widely used in tomato production prior to transplanting to help suppress soil-borne pests and pathogens (Rosskopf et al., Reference Rosskopf, Chellemi, Kokalis-Burelle and Church2005). However, these alternative chemical fumigants often lack adequate efficacy for pest suppression and fumigant application and management regulations are becoming more stringent due to environmental and human health concerns (Duniway, Reference Duniway2002; Rosskopf et al., Reference Rosskopf, Chellemi, Kokalis-Burelle and Church2005). Considering the goal of long-term sustainability for vegetable production systems, there is an urgent need to explore and implement non-chemical alternatives to synthetic chemical fumigants (Rosskopf et al., Reference Rosskopf, Chellemi, Kokalis-Burelle and Church2005; Di Gioia et al., Reference Di Gioia, Ozores-Hampton, Hong, Kokalis-Burelle, Albano, Zhao, Black, Gao, Wilson, Thomas, Moore, Swisher, Guo and Rosskopf2016), while taking into consideration economic viability and social acceptability (Martin, Reference Martin2003).
Non-synthetic alternative tools for managing soil-borne pathogen and pests in vegetable cropping systems include crop rotation, soil solarization, biofumigation, biosolarization, anaerobic soil disinfestation (ASD) and the use of various organic soil amendments (Rosskopf et al., Reference Rosskopf, Chellemi, Kokalis-Burelle and Church2005; Sydorovych et al., Reference Sydorovych, Safley, Welker, Ferguson, Monks, Jennings, Driver and Louws2008). ASD, also known as ‘biological soil disinfestation’ (Goud et al., Reference Goud, Termorshuizen, Blok and van Bruggen2004; Messiha et al., Reference Messiha, van Diepeningen, Wenneker, van Beuningen, Janse, Coenen, Termorshuizen, van Bruggen and Blok2007) or ‘reductive soil disinfestation’ (Katase et al., Reference Katase, Kubo, Ushio, Ootsuka, Takeuchi and Mizukubo2009), is a relatively new technique and it is gaining interest among researchers and producers as a cultural and biological alternative to chemical soil fumigation (Butler et al., Reference Butler, Kokalis-Burelle, Muramoto, Shennan, McCollum and Rosskopf2012a, Reference Butler, Rosskopf, Kokalis-Burelle, Albano, Muramoto and Shennan2012b; Rosskopf et al., Reference Rosskopf, Serrano-Pérez, Hong, Shrestha, del Carmen Rodríguez-Molina, Martin, Kokalis-Burelle, Shennan, Muramoto, Butler, Meghavansi and Varma2015). The ASD method used in vegetable production consists of adding easily decomposable carbon sources (e.g., rice bran, molasses (M), or cover crop residues), followed by irrigation to saturation and tarping with totally impermeable film (TIF) for a duration of approximately 3 weeks (Butler et al., Reference Butler, Kokalis-Burelle, Muramoto, Shennan, McCollum and Rosskopf2012a; Rosskopf et al., Reference Rosskopf, Serrano-Pérez, Hong, Shrestha, del Carmen Rodríguez-Molina, Martin, Kokalis-Burelle, Shennan, Muramoto, Butler, Meghavansi and Varma2015). Growth of soil anaerobic microorganisms rises as a direct response to a readily available carbon source and anaerobic condition (van Agtmaal et al., Reference van Agtmaal, van Os, Hol, Hundscheid, Runia, Hordijk and de Boer2015). Organic acids, including butyric, acetic and propionic acids are produced as a result of anaerobic decomposition (Messiha et al., Reference Messiha, van Diepeningen, Wenneker, van Beuningen, Janse, Coenen, Termorshuizen, van Bruggen and Blok2007). These toxins are often to be lethal to soil-borne plant pathogens and plant-parasitic nematodes (Momma et al., Reference Momma, Yamamoto, Simandi and Shishido2006; Momma, Reference Momma2008; Butler et al., Reference Butler, Kokalis-Burelle, Muramoto, Shennan, McCollum and Rosskopf2012a, Reference Butler, Rosskopf, Kokalis-Burelle, Albano, Muramoto and Shennan2012b). Moreover, changes in resident soil microbial communities, fostered by resource availability in soil and the rhizosphere may actively contribute to disease suppression (Mazzola and Freilich, Reference Mazzola and Freilich2017; Shennan et al., Reference Shennan, Muramoto, Koike, Baird, Fennimore, Samtani, Bolda, Dara, Daugovish, Lazarovits, Butler, Rosskopf, Kokalis-Burelle, Klonsky and Mazzola2017).
Recent studies by Di Gioia et al. (Reference Di Gioia, Ozores-Hampton, Hong, Kokalis-Burelle, Albano, Zhao, Black, Gao, Wilson, Thomas, Moore, Swisher, Guo and Rosskopf2016) and Guo et al. (Reference Guo, Di Gioia, Zhao, Ozores-Hampton, Swisher, Hong, Kokalis-Burelle, DeLong and Rosskopf2017) compared ASD treatments using M and composted poultry litter (CPL) with conventional soil fumigation for tomato production in Florida sandy soils and reported positive impacts on nematode suppression while improving tomato yield. However, concerns associated with cost-effectiveness of using high M rates, the resulting increase in phosphorous application with the use of CPL (Di Gioia et al., Reference Di Gioia, Ozores-Hampton, Zhao, Thomas, Wilson, Li, Hong, Albano, Swisher and Rosskopf2017) and the potential food safety and cross-contamination risks posed by improperly CPL (Islam et al., Reference Islam, Doyle, Phatak, Millner and Jiang2005) motivate the search for alternative inputs for ASD treatment and the evaluation of other potential biological soil management strategies.
Composted yard waste (CYW) and other types of food waste as organic soil amendments have been utilized for improving soil fertility and quality in vegetable production (Ozores-Hampton and Peach, Reference Ozores-Hampton and Peach2002; Ozores-Hampton et al., Reference Ozores-Hampton, Stansly and Salame2011, Reference Ozores-Hampton, McSorley and Stansly2012). As a locally available, relatively inexpensive renewable resource, CYW usually contains lower levels of nutrients than animal manure-based composts. Given that CYW has been increasingly used by tomato growers in Florida, its use as a potential component of ASD to replace CPL deserves further evaluation.
The native soil microbial community plays a key role in determining the effectiveness of ASD, as it is manipulated during the ASD process for enhancing the temporary growth of facultative and obligate anaerobes that are responsible for releasing organic acids and volatile organic compounds with potential toxicity against certain soil-borne pests and pathogens (Momma et al., Reference Momma, Yamamoto, Simandi and Shishido2006; Mowlick et al., Reference Mowlick, Yasukawa, Inoue, Takehara, Kaku, Ueki and Ueki2013; Rosskopf et al., Reference Rosskopf, Serrano-Pérez, Hong, Shrestha, del Carmen Rodríguez-Molina, Martin, Kokalis-Burelle, Shennan, Muramoto, Butler, Meghavansi and Varma2015). As research continues to reveal the importance of the soil microbiome and its direct role in building suppressive soils, as well as in promoting plant growth and plant responses to stress conditions, an alternative strategy that is gaining interest among vegetable growers is the application of amendments containing selected microbes with specific beneficial properties (Nielsen and Sørensen, Reference Nielsen and Sørensen1997; Pérez-García et al., Reference Pérez-García, Romero and De Vicente2011; Tan et al., Reference Tan, Jiang, Song, Huang, Ling, Xu and Shen2013). An example of such a strategy, recently proposed to vegetable growers in Florida, is the commercial formulation Soil Symphony Amendment (SSA) implemented by applying formulated feedstock to the soil with a biological mix containing chitosan, agricultural carbon source (M) and a solution of microorganisms including: Bacillus subtilis, B. laterosporus, B. liceniformis, B. megaterium, B. pumilus and Paenibacillus polymyxa, which are known as plant-growth-promoting rhizobacteria (PGPR) (Nielsen and Sørensen, Reference Nielsen and Sørensen1997; Kumar et al., Reference Kumar, Prakash, Johri and Maheshwari2011). Although ample literature exists on the beneficial impacts of PGPR in vegetable crops (e.g., Kokalis-Burelle et al., Reference Kokalis-Burelle, Vavrina, Rosskopf and Shelby2002; Beneduzi et al., Reference Beneduzi, Ambrosini and Passaglia2012; Ali et al., Reference Ali, Charles and Glick2014), very little is known about the efficacy of the more recently developed commercial formulations applied at field scale. Moreover, it is interesting to compare the ASD method, relying primarily on the native soil microbial community, with the application of soil biological amendments (SBS) containing selected non-native (laboratory-grown) potentially beneficial microbes.
Therefore, a field study was carried out on fresh-market tomato in two different locations in Florida, to compare the effects of ASD approach (Di Gioia et al., Reference Di Gioia, Ozores-Hampton, Hong, Kokalis-Burelle, Albano, Zhao, Black, Gao, Wilson, Thomas, Moore, Swisher, Guo and Rosskopf2016; Guo et al., Reference Guo, Di Gioia, Zhao, Ozores-Hampton, Swisher, Hong, Kokalis-Burelle, DeLong and Rosskopf2017) implemented using M as a C source and CPL as a supplemental amendment with (i) an untreated control, (ii) the standard CSF, (iii) ASD implemented using different application rates of CYW as an alternative to CPL, (iv) soil amended with CYW only and (v) SSA applied by itself or in combination with CYW, in terms of root-knot nematode and weed control, and tomato fruit yield and quality.
Materials and methods
Soil management treatments and field experiments
Two field experiments were conducted on fresh-market tomato during July 18 to November 9, 2016 at the University of Florida Plant Science Research and Education Unit at Citra, FL (29°43′32″N and 82°25′8″W) and from August 22 to December 22, 2016 at the University of Florida, South West Florida Research and Education Center at Immokalee, FL (26°27′38″N and 81°26′9″W).
The soil type at Citra is Gainesville loamy sand (hyperthermic, coated typic quartzipsamment). The mean, minimum and maximum soil temperatures at 5 cm depth during the 3-week soil treatment were 31.5, 20.7 and 73.8 °C, respectively. The Immokalee site soil is characterized as Immokalee fine sand (sandy, siliceous, hyperthermic Arenic Haplaquods). The mean, minimum and maximum soil temperatures during the 3-week soil treatment were 30.4, 23.7 and 69.8 °C, respectively.
A randomized complete block design with four replications was used in both experiments. The soil management treatments included untreated control, CSF, ASD0.5 (Guo et al., Reference Guo, Di Gioia, Zhao, Ozores-Hampton, Swisher, Hong, Kokalis-Burelle, DeLong and Rosskopf2017), CYW1 + M, CYW0.5 + M, SSA, CYW1 and CYW1 + SSA (Table 1). The CPL is reported to contain 2.6% N, 1.4% P and 2.5% K (Di Gioia et al., Reference Di Gioia, Ozores-Hampton, Hong, Kokalis-Burelle, Albano, Zhao, Black, Gao, Wilson, Thomas, Moore, Swisher, Guo and Rosskopf2016). The CYW contains 1.05% N, 0.08% P and 0.19% K. Pre-plant components of all the soil treatments were applied on July 18, 2016 in Citra and on August 22, 2016 in Immokalee.
Table 1. Experimental treatment details

a A solid product with a formulated composition of chitin, glucose, wheat middlings, corn gluten, citrus pulp, urea, bone meal, cotton seed meal, alfalfa and diatomaceous earth was applied at 816 kg ha−1 to the initial beds, followed by application of a liquid mixture containing Nutri-TAC (chitosan), M and soil biological amendment (SBS) (a solution containing a mixture of B. subtilis, B. laterosporus, B. liceniformis, B. megaterium, B. pumilus and P. polymyxa). The liquid mixture was prepared using 18.7 L Nutri-TAC, 37.4 L M and 0.05 L SBS in 935.4 L of water for applying to 1 ha of tomato beds. For the SSA and CYW1 + SSA treatments, M was applied through fertigation at the rate of 37.4 L ha−1 once per week starting 1 week after field transplanting. In addition, the full liquid mix containing M, chitosan and SBS prepared as described above, was injected by fertigation every 30 days until harvest.
At both experimental sites, soil was rototilled and a pre-plant fertilizer (bottom mix) 10 N-10 P2O5-10 K2O was broadcasted over the field before initial bed preparation at the rate of 56 kg ha−1 of N, 24.4 kg ha−1 P and 46.5 kg ha−1 K. M (1:1 volume dilution with water) and CPL for the ASD0.5 and other soil treatments were applied to the bed top of the initial beds made for soil treatment implementation. In the SSA treatment, a solid product with a formulated composition of chitin, glucose, wheat middlings, corn gluten, citrus pulp, urea, bone meal, cotton seed meal, alfalfa and diatomaceous earth was applied at 816 kg ha−1 to the initial beds, followed by application of a liquid mixture containing Nutri-TAC (chitosan), M and SBS (a solution containing a mixture of B. subtilis, B. laterosporus, B. liceniformis, B. megaterium, B. pumilus and P. polymyxa). The liquid mixture was prepared using 18.7 L Nutri-TAC, 37.4 L M and 0.05 L SBS in 935.4 L of water for applying to 1 ha of tomato beds. For the SSA and CYW1 + SSA treatments, M was applied through fertigation at the rate of 37.4 L ha−1 once per week starting 1 week after field transplanting. In addition, the full liquid mix containing M, chitosan and SBS prepared as described above was injected by fertigation every 30 days until harvest.
The final raised beds were formed after amendments were applied by tilling to a depth of 15 cm with a rotary cultivator. Before mulch application, a pre-emergent herbicide Reflex (a.i. fomesafen) (Syngenta Crop Protection, Greensboro, NC, USA) at the rate of 1.2 L ha−1 was sprayed over all beds. The beds were then covered with 0.03 mm black/white VaporSafe® TIF (Raven Industries Inc., Sioux Falls, SD, USA). Each bed was 18.3 m long and 0.9 m wide with 1.8 m between centers of two adjacent beds. Two drip irrigation lines (Jain Irrigation Inc., Haines City, FL, USA; 20 cm emitter spacing, 0.98 L h−1 emitter rate) were placed approximately 2.5 cm below the soil surface in each bed. Except for the untreated control, CSF and SSA, all other treatments received 5 cm initial irrigation to the top 10 cm (10 psi water pressure for 4 h) of covered beds to fill soil pore space and enhance the development of anaerobic conditions (Butler et al., Reference Butler, Kokalis-Burelle, Muramoto, Shennan, McCollum and Rosskopf2012a).
Three weeks after soil treatment, tomato seedlings at the four-leaf-stage were transplanted into each bed in a single row with plant spacing of 0.45 m on August 8, 2016 in Citra and on September 12, 2016 in Immokalee. Determinate tomato cultivar Tribute (Sakata, Morgan Hill, CA, USA) was used in both locations. Potassium nitrate (13 N-0 P2O5-46 K2O) and ammonium nitrate (34 N-0 P2O5-0 K2O in Citra and 27 N-0 P2O5-0 K2O in Immokalee) solutions were injected via drip irrigation once a week starting 1 week after transplanting (WAT) until harvest in both locations. Calcium nitrate (15.5 N-0 P2O5-0 K2O, 19% Ca) was applied in place of ammonium nitrate starting at 7 WAT in Immokalee. The post-transplanting application rates of total N and K in Citra were 181.2 and 159.8 kg ha−1, respectively, and in Immokalee the rates were at 215.5 and 218 kg ha−1 with 143.2 kg ha−1 of Ca.
Soil anaerobic condition monitoring
Soil redox potential (Eh), temperature and water content were monitored for 3 weeks after soil treatment application. Two oxidation–reduction potential probes (Pt combination electrodes with Ag/AgCl reference system; Sensorex, Garden Grove, CA, USA) were set in each bed at 15 cm depth. The raw values were used to correlate to the redox potential of a standard hydrogen electrode (Fiedler et al., Reference Fiedler, Vepraskas and Richardson2007). The electrodes were monitored using an automatic data logger (CR 1000, AM 16/32 multiplexes, Campbell Scientific, Logan, UT, USA). The redox potential values were recorded to obtain hourly average data.
Soil anaerobic conditions were determined by the cumulative values of hourly average redox potential values below a critical redox potential (CEh) calculated as: CEh = 595 mV ‒ (60 mV × soil pH) (NTCHS, 2015; Rabenhorst and Castenson, Reference Rabenhorst and Castenson2005; USDA-NRCS, Reference Vasilas, Hurt and Noble2010).
Weed population assessment, soil nematode analysis and tomato plant measurements
A mix of yellow nutsedge (Cyperus esculentus L.) and purple nutsedge (C. rotundus L.) composed the predominant weed population at both research sites. In Citra, due to the dense nutsedge and grass populations, the herbicide Sandea (Gowan Company, Yuma, AZ, USA) was applied over the bed top at the rate of 73.1 mL ha−1 to kill nutsedge at 10 days after transplanting (DAT) and SelectMax herbicide (Valent LLC, Walnut Creek, CA, USA) was applied at the rate of 1.17 L ha−1 to kill the grass weeds at 32 DAT. At both sites, weeds were counted in each plot and presented as an average number of weeds per plot for each treatment. Weed counts were conducted at 7 and 78 DAT at Citra and at 44, 67 and 101 DAT in Immokalee. The number of nutsedge emerging through TIF and in the planting holes were counted together in Citra, while the nutsedge along with other weeds emerging through TIF and in planting holes were counted separately in Immokalee. Weed coverage percentage was also determined by visual estimation in Immokalee.
For soil nematode analysis, soils were sampled from the top 15 cm at 0, 63 and 92 DAT from each plot in Citra and 0, 42 and 78 DAT in Immokalee. For plant root nematode infestation assessment, two plants were randomly selected from each plot at 92 DAT in Citra and 78 DAT in Immokalee. These plants were used to assess root galling and root nematodes as well as fresh root weight, stem diameter and root condition. Root galling was assessed on a scale of 0–10, with 0 indicating no galling and 10 indicating severe galling with dead roots (Zeck, Reference Zeck1971; Bridge and Page, Reference Bridge and Page1980). A rating scale of 0–5 was used to assess root condition, with 0 indicating healthy roots and 5 indicating diseased and necrotic roots (Butler et al., Reference Butler, Kokalis-Burelle, Muramoto, Shennan, McCollum and Rosskopf2012a). In addition, all ten plants in the harvest unit were used for root gall index ratings, root condition ratings, and stem diameter measurement in Citra after the final harvest (99 DAT).
Nematodes at the second juvenile stage (J2) were extracted from both root (from the two-plant samples) and soil samples utilizing the Baermann funnel technique (Walker and Wilson, Reference Walker and Wilson1960). Extracted nematodes were counted (nematodes per 100 cm3 of soil or per gram of fresh root) and identified as root-knot nematode (Meloidogyne spp.), stubby-root nematode (Nanidorus minor), spiral nematode (Helicotylenchus pseudorobustus) and non-parasitic free-living nematodes.
Tomato yield measurements and fruit quality assessment
Tomato yields were measured from a harvest unit consisting of ten consecutive plants in each plot. Tomato fruit at mature green or more advanced ripening stages were harvested at 78, 86 and 93 DAT in Citra, and at 81, 88 and 101 DAT in Immokalee. All fruits were graded as extra-large (>7.00 cm, XL), large (6.35–7.06 cm, L), medium (5.72–6.43 cm, M) and culls (small and defective fruit) following the USDA tomato grade standards (USDA, 1991) before weighing. The extra-large, large and medium fruit weights were summed to calculate marketable yield (MY). The total MY and cull weights were summed to determine the total season yield.
At the first harvest in each location, 20 representative mature-green tomatoes were collected from each plot and stored at 20 °C until table ripe stage for the assessment of fruit color, firmness, pH, total soluble solids (TSS), titratable acidity (TA) and dry matter (DM) content. The external fruit color was measured on four selected tomatoes per plot in the central part of the fruit (between blossom and distal end) using a CR-400 Chroma Meter (Konica Minolta Sensing Americas, Inc., NJ, USA), and a* values indicating redness–greenness were recorded. The higher value of a* shows better redness. Fruit firmness was measured at the blossom end of the same tomato fruit using a TA HD Plus Texture Analyzer (Texture Technologies Corp., NY, USA) equipped with a 5 kg load cell. The maximum force (N) required to compress the fruit by 2 mm was recorded. One-fourth of each of the four tomatoes were then homogenized using a blender and centrifuged at 4 °C for 20 min, and the resulting supernatant was filtered through four-layer cheesecloth for further analysis of TSS, pH and TA following the procedure of Djidonou et al. (Reference Djidonou, Simonne, Koch, Brecht and Zhao2016). About 150–200 g sliced fresh tomato of the same four fruit samples per plot were weighed and oven dried at 65 °C until constant weight to calculate fruit DM content.
Statistical analyses
Data analyses were conducted separately for the two locations using the GLIMMIX procedure in SAS Version 9.3 software (SAS Institute, Cary, NC, USA) following a randomized complete block design where treatment was fixed effect and block was random effect. Multiple comparisons of different measurements among treatments were performed using Fisher's least significant difference (LSD) test at P ≤ 0.05.
Results
Soil anaerobic conditions
The ASD0.5 treatment resulted in significantly greater accumulation of anaerobic conditions compared with all other treatments at both research sites. The cumulative values of redox potential reached 17,219 and 19,445 mVhr in Citra and Immokalee, respectively (Fig. 1). In Immokalee, CYW1 + M exhibited a higher level of anaerobicity than control, CSF, SSA, CYW1 and CYW + SSA. Significant differences were not detected between cumulative redox potential values of CYW1 + M and CYW0.5 + M at P ≤ 0.05 at either location.

Fig. 1. The cumulative soil redox potential as affected by soil amendment treatments (in mVhr × 1000) during the initial 3-week period in Citra and Immokalee, FL. aControl: untreated control; CSF: chemical soil fumigation with Pic-Clor60 containing chloropicrin (59.6%) and 1,3-dichloropropene (39.0%) at a rate of 224 kg ha−1; ASD0.5: ASD with 6.93 m3 ha−1 of molasses and 11 Mg ha−1 of composted poultry litter; SSA: Soil Symphony Amendments 450 at 816 kg ha−1; CYW1: composted yard waste at 26.9 Mg ha−1; CYW1 + M: ASD with CYW at 26.9 Mg ha−1 plus 6.93 m3 ha−1 of molasses; CYW0.5 + M: ASD with CYW at 13.45 Mg ha−1 plus 6.93 m3 ha−1 of molasses; and CYW1 + SSA: CYW at 26.9 Mg ha−1 plus SSA. Bars followed by same letter within each location were not significantly different by Fisher's LSD test at P ≤ 0.05.
Nematode assessment, root conditions and stem diameter
Soil densities of root-knot nematodes as well as non-parasitic nematodes did not differ significantly among soil treatments at any sampling time in both sites (data not shown). The average density of root-knot nematodes was 182.8 and 6.6 counts 100−1 cm3 of soil in Citra and Immokalee, respectively. Similarly, the average density of non-parasitic nematodes was 347.7 and 326.8 counts 100−1 cm3 of soil in Citra and Immokalee, respectively.
In Citra, the number of root-knot nematodes isolated from roots was significantly greater in the untreated control compared with CSF, ASD0.5 and SSA; however, it was not significantly different from the treatments containing CYW (Table 2). Interestingly, untreated control, CYW1 + M and CYW1 + SSA showed significantly greater counts of non-parasitic nematodes in roots in contrast to ASD0.5 and CYW1, which were statistically similar to CSF, SSA and CYW0.5 + M. Moreover, CSF, SSA and CYW0.5 + M were not significantly different from ASD0.5 and CYW1 in numbers of non-parasitic nematodes isolated from roots (Table 2). The root densities of spiral and stubby root nematodes and root weight did not differ among soil treatments. The root condition rating index, root gall index and stem diameter were not significantly different among treatments at 92 DAT. However, when all ten plants in the harvest unit were evaluated at 99 DAT, significant differences among the treatments were found in root gall index and root condition rating index (Fig. 2). With respect to root condition, no difference was observed between SSA, CYW1 + M, CSF and ASD0.5; however, CYW1, CYW0.5 + M and CYW + SSA resulted in significantly greater (more diseased) ratings than those from CSF and ASD0.5, but lower (less diseased) ratings relative to the control (Fig. 2). CSF, ASD0.5, CYW1 + M and CYW0.5 + M significantly reduced the root gall index compared with control, whereas SSA, CYW1 and CYW1 + SSA did not differ significantly from control. Similar root gall ratings were observed among ASD0.5, SSA, CYW1, CYW1 + M, CYW0.5 + M and CYW1 + SSA, which were significantly higher than CSF, except for CYM1 + M. Plant stem diameter was significantly greater in ASD0.5 and CYW1 + M compared with control, CSF and CYW1. Almost all biological soil management treatments showed significantly greater stem diameter in comparison to control except CYW1 and CYW1 + SSA (Fig. 2). The relatively low diameter measured at 99 DAT as compared with the measurements at 92 DAT (Table 2) was probably due to the final harvest, and plant condition might have worsened after the final harvest event.

Fig. 2. Root gall index ratings and root condition ratings (I), and stem diameter (II) as affected by treatments after the final harvest (99 DAT) in Citra, FL. aControl: untreated control; CSF: chemical soil fumigation with Pic-Clor60 containing chloropicrin (59.6%) and 1,3-dichloropropene (39.0%) at a rate of 224 kg ha−1; ASD0.5: ASD with 6.93 m3 ha−1 of molasses and 11 Mg ha−1 of composted poultry litter; SSA: Soil Symphony Amendments 450 at 816 kg ha−1; CYW1: composted yard waste at 26.9 Mg ha−1; CYW1 + M: ASD with CYW at 26.9 Mg ha−1 plus 6.93 m3 ha−1 of molasses; CYW0.5 + M: ASD with CYW at 13.45 Mg ha−1 plus 6.93 m3 ha−1 of molasses, and CYW1 + SSA: CYW at 26.9 Mg ha−1 plus SSA. Bars followed by same letter within each category were not significantly different by Fisher's LSD test at P ≤ 0.05. Root condition: 0 = clean, white roots, 5 = completely rotted and discolored roots. Gall index: 0 = no galling, 10 = complete galling.
Table 2. Root nematodes and plant biometrics at the end of the tomato growing season (92 DAT) in Citra, FL

a Control: untreated control; CSF: chemical soil fumigation with Pic-Clor60 containing chloropicrin (59.6%) and 1,3-dichloropropene (39.0%) at a rate of 224 kg ha−1; ASD0.5: anaerobic soil disinfestation (ASD) with 6.93 m3 ha−1 of molasses and 11 Mg ha−1 of composted poultry litter; SSA: Soil Symphony Amendment at 816 kg ha−1; CYW1: composted yard waste at 26.9 Mg ha−1; CYW1 + M: ASD with CYW at 26.9 Mg ha−1 plus 6.93 m3 ha−1 of molasses; CYW0.5 + M: ASD with CYW at 13.45 Mg ha−1 plus 6.93 m3 ha−1 of molasses; and CYW1 + SSA: CYW at 26.9 Mg ha−1 plus SSA.
b Root condition: 0 = clean, white roots, 5 = completely rotted and discolored roots.
c Nematode gall index: 0 = no galling, 10 = complete galling.
Means within a column followed by same letter were not significantly different by Fisher's LSD test at P ≤ 0.05.
Data are presented as mean ± standard deviation of four replications.
Overall, the results from Immokalee followed a similar trend to those collected at the Citra location. Root densities of root-knot, spiral and stubby root nematodes were not significantly different among the control and treatments at 78 DAT (Table 3). Additional sampling of ten plants to assess root condition and root galling was not conducted due to low population density of plant-parasitic nematodes at this location. Stem diameters were significantly smaller in control and CYW1 + SSA compared with ASD0.5 and CSF, while ASD0.5 showed a similar stem diameter to those treated with CSF, CYW1, CYW1 + M and CYW0.5 + M. ASD0.5 significantly increased root weight in comparison to CSF, control and CYW1. Meanwhile, ASD0.5 exhibited improved root conditions as reflected by the significantly lower ratings than the control and other treatments, but was similar to those from CYW1 + M and CYW0.5 + M (Table 3).
Table 3. Root nematodes and plant biometrics at the end of the tomato growing season (78 DAT) in Immokalee, FL
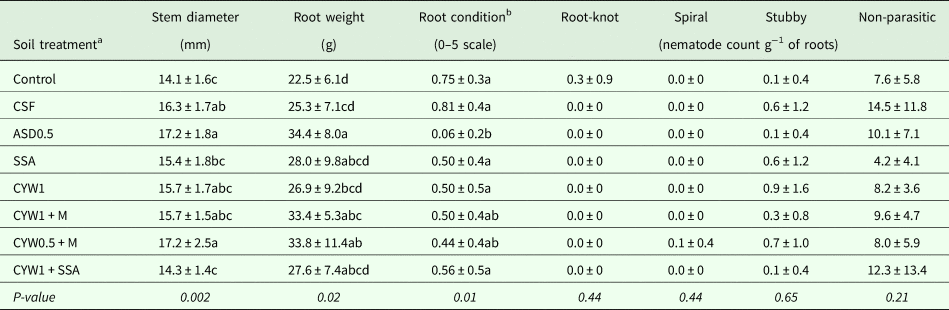
a Control: untreated control; CSF: chemical soil fumigation with Pic-Clor60 containing chloropicrin (59.6%) and 1,3-dichloropropene (39.0%) at a rate of 224 kg ha−1; ASD0.5: anaerobic soil disinfestation (ASD) with 6.93 m3 ha−1 of molasses and 11 Mg ha−1 of composted poultry litter; SSA: Soil Symphony Amendment at 816 kg ha−1; CYW1: composted yard waste at 26.9 Mg ha−1; CYW1 + M: ASD with CYW at 26.9 Mg ha−1 plus 6.93 m3 ha−1 of molasses; CYW0.5 + M: ASD with CYW at 13.45 Mg ha−1 plus 6.93 m3 ha−1 of molasses; and CYW1 + SSA: CYW at 26.9 Mg ha−1 plus SSA.
b Root condition: 0 = clean, white roots, 5 = completely rotted and discolored roots.
Means within a column followed by same letter were not significantly different by Fisher's LSD test at P ≤ 0.05.
Data are presented as mean ± standard deviation of four replications.
Weed populations
In Citra, the nutsedge count, including planting holes and those emerging through the plastic mulch, at 7 DAT was significantly lower in CSF than control and other soil treatments, while it was significantly higher in SSA and CYW1 + M compared with CSF and the untreated control (Table 4). There was a substantial reduction of nutsedge population in all the plots following the herbicide spray with Sandea and SelectMax, while the nutsedge count remained lowest in CSF at 78 DAT. The grass and broadleaf weeds were negligible in Citra and thus were not recorded.
Table 4. Effects of soil treatments on nutsedge weed counts in Citra, FL

a Control: untreated control; CSF: chemical soil fumigation with Pic-Clor60 containing chloropicrin (59.6%) and 1,3-dichloropropene (39.0%) at a rate of 224 kg ha−1; ASD0.5: anaerobic soil disinfestation (ASD) with 6.93 m3 ha−1 of molasses and 11 Mg ha−1 of composted poultry litter; SSA: Soil Symphony Amendment at 816 kg ha−1; CYW1: composted yard waste at 26.9 Mg ha−1; CYW1 + M: ASD with CYW at 26.9 Mg ha−1 plus 6.93 m3 ha−1 of molasses; CYW0.5 + M: ASD with CYW at 13.45 Mg ha−1 plus 6.93 m3 ha−1 of molasses; and CYW1 + SSA: CYW at 26.9 Mg ha−1 plus SSA.
Means within a column followed by same letter were not significantly different by Fisher's LSD test at P ≤ 0.05.
DAT: days after transplanting.
Bed area per plot: 18.3 m × 0.9 m = 16.47 m2.
Data are presented as mean ± standard deviation of four replications.
Weed pressure was lower in the Immokalee trial than that of Citra. The nutsedge counts per bed and per planting hole were not significantly different among treatments during each assessment period (Table 5). The broadleaf weed count in planting holes was lowest in CSF, which was statistically similar to CYW1 and control, but significantly lower compared with other treatments at 44 and 101 DAT. The same trend was observed in total weed count per planting hole at 44 and 101 DAT. At 67 DAT, the broadleaf and total weed counts in planting holes were significantly lower in CSF in contrast to control and all other treatments (Table 5). Grass and broadleaf weeds in the bed and grass weeds in planting holes were negligible in Immokalee. The weed coverage percentage was significantly lower in CSF compared with control and CYW1 + M, but was similar to all other treatments at 44 DAT (Table 5). At 67 DAT, the weed coverage percentage was significantly lower in CSF compared with control, CYW1 + M, SSA and ASD0.5, but was similar to CYW1, CYW0.5 + M and CYW + SSA. No significant differences in weed coverage were observed among treatments at 101 DAT (Table 7).
Table 5. Weed count and coverage as affected by soil treatments in Immokalee, FL

a Control: untreated control; CSF: chemical soil fumigation with Pic-Clor60 containing chloropicrin (59.6%) and 1,3-dichloropropene (39.0%) at a rate of 224 kg ha−1; ASD0.5: anaerobic soil disinfestation (ASD) with 6.93 m3 ha−1 of molasses and 11 Mg ha−1 of composted poultry litter; SSA: Soil Symphony Amendment at 816 kg ha−1; CYW1: composted yard waste at 26.9 Mg ha−1; CYW1 + M: ASD with CYW at 26.9 Mg ha−1 plus 6.93 m3 ha−1 of molasses; CYW0.5 + M: ASD with CYW at 13.45 Mg ha−1 plus 6.93 m3 ha−1 of molasses; and CYW1 + SSA: CYW at 26.9 Mg ha−1 plus SSA.
Means within a column followed by same letter were not significantly different by Fisher's LSD test at P ≤ 0.05.
DAT, days after transplanting.
Bed area per plot: 18.3 m × 0.9 m = 16.47 m2.
Data are presented as mean ± standard deviation of 4 replications.
Tomato yield
During the first and second harvests in Citra, no significant differences were observed among treatments with respect to marketable and total yields as well as the yields of extra-large, large and medium-sized tomatoes (Table 6). The whole season marketable and total yields were significantly greater in CSF, ASD0.5 and CYW1 + M than control, CYW1 and SSA, which exhibited the lowest yield (Table 6). The total MY of ASD0.5 was 57.1% more than that of control, while CYW1 + M and CSF showed an increase of 42 and 49.1%, respectively. Among all the biological soil management treatments, SSA, CYW1 and CYW1 + SSA led to significantly lower total yields than ASD0.5, while ASD0.5, CYW1 + M and CYW0.5 + M did not differ significantly. The greater yield resulting from CSF, ASD0.5 and CYW1 + M also stood out in the total medium fruit yield. In addition, ASD0.5, CYW1 + M and CYW0.5 + M showed significantly higher cull fruit yield than untreated control. The biological soil treatments including ASD0.5, CYW1 + M, CYW0.5 + M and CYW1 + SSA produced statistically similar yields as CSF for the season total. Furthermore, CYW0.5 + M and CYW1 + SSA did not differ significantly from CSF, ASD0.5 or control in marketable and total yields.
Table 6. Tomato yield components as affected by soil treatments in Citra, FL

XL, extra-large fruit (diameter > 7.00 cm); L, large fruit (6.35 < diameter < 7.00 cm); M, medium fruit (5.72 < diameter < 6.43 cm); MY, marketable yield; TY, total yield.
a Control: untreated control; CSF: chemical soil fumigation with Pic-Clor60 containing chloropicrin (59.6%) and 1,3-dichloropropene (39.0%) at a rate of 224 kg ha−1; ASD0.5: anaerobic soil disinfestation (ASD) with 6.93 m3 ha−1 of molasses and 11 Mg ha−1 of composted poultry litter; SSA: Soil Symphony Amendment at 816 kg ha−1; CYW1: composted yard waste at 26.9 Mg ha−1; CYW1 + M: ASD with CYW at 26.9 Mg ha−1 plus 6.93 m3 ha−1 of molasses; CYW0.5 + M: ASD with CYW at 13.45 Mg ha−1 plus 6.93 m3 ha−1 of molasses; and CYW1 + SSA: CYW at 26.9 Mg ha−1 plus SSA.
Means within a column followed by same letter were not significantly different by Fisher's LSD test at P ≤ 0.05.
Data are presented as mean ± standard deviation of four replications.
Tomato yields were higher and the differences among treatments were more pronounced in Immokalee than in Citra. At the first harvest, CSF, CYW1 + M, CYW0.5 + M and CYW1 + SSA resulted in significantly higher yields of extra-large fruit than were harvested from control. Moreover, CSF, ASD0.5, CYW1 + M and CYW1 + SSA resulted in significantly greater yields of large fruit as well as improved marketable and total yields compared with control (Table 7). After the first and second harvests, CSF and all of the biological soil treatments except CYW1 exhibited enhanced marketable and total fruit yields along with higher yields of extra-large fruit in comparison with yields in control. With respect to the whole season yield components and compared with control, CSF and biological soil treatments except for CYW1 exhibited higher yields of extra-large fruit, while CSF, ASD0.5, CYW1 + M and CYW0.5 + M improved large fruit yields (Table 7). CSF and all of the biological soil treatments resulted in significantly greater marketable and total yields than control. The three ASD treatments ASD0.5, CYW1 + M and CYW0.5 + M did not differ significantly from CSF in marketable or total fruit yield. The total MY of ASD0.5 was 59.2% higher than the yield of the control, while CYW1 + M showed an increase of 53.6% relative to the control. Compared with other biological soil treatments, SSA and CYW1 showed significantly lower marketable and total yields.
Table 7. Cumulative tomato yield components as affected by soil treatments in Immokalee, FL

XL, extra-large fruit (diameter > 7.00 cm); L, large fruit (6.35 < diameter < 7.00 cm); M, medium fruit (5.72 < diameter < 6.43 cm); MY, marketable yield; TY, total yield.
a Control: untreated control; CSF: chemical soil fumigation with Pic-Clor60 containing chloropicrin (59.6%) and 1,3-dichloropropene (39.0%) at a rate of 224 kg ha−1; ASD0.5: anaerobic soil disinfestation (ASD) with 6.93 m3 ha−1 of molasses and 11 Mg ha−1 of composted poultry litter; SSA: Soil Symphony Amendment at 816 kg ha−1; CYW1: composted yard waste at 26.9 Mg ha−1; CYW1 + M: ASD with CYW at 26.9 Mg ha−1 plus 6.93 m3 ha−1 of molasses; CYW0.5 + M: ASD with CYW at 13.45 Mg ha−1 plus 6.93 m3 ha−1 of molasses; and CYW1 + SSA: CYW at 26.9 Mg ha−1 plus SSA.
Means within a column followed by same letter were not significantly different by Fisher's LSD test at P ≤ 0.05.
Data are presented as mean ± standard deviation of four replications.
Fruit quality
Soil treatments did not result in significantly different effects on fruit quality attributes including fruit firmness, pH, TSS and DM content in either location (Table 8). The fruit color and TA were not impacted by treatments at Citra, but showed some differences among treatments at Immokalee. The fruit color, as measured by Chroma Meter and indicated by redness–greenness index a*, was significantly lower in CYW1 + SSA, CYW1 and ASD0.5 compared with control but was similar to CSF. The TA was significantly lower in CSF and SSA compared with ASD0.5, CYW1 + M and CYW0.5 + M, but was similar to control, CYW1 and CYW1 + SSA.
Table 8. Effects of soil treatment on tomato fruit quality attributes in Citra and Immokalee, FL

a Control: untreated control; CSF: chemical soil fumigation with Pic-Clor60 containing chloropicrin (59.6%) and 1,3-dichloropropene (39.0%) at a rate of 224 kg ha−1; ASD0.5: anaerobic soil disinfestation (ASD) with 6.93 m3 ha−1 of molasses and 11 Mg ha−1 of composted poultry litter; SSA: Soil Symphony Amendment at 816 kg ha−1; CYW1: composted yard waste at 26.9 Mg ha−1; CYW1 + M: ASD with CYW at 26.9 Mg ha−1 plus 6.93 m3 ha−1 of molasses; CYW0.5 + M: ASD with CYW at 13.45 Mg ha−1 plus 6.93 m3 ha−1 of molasses; and CYW1 + SSA: CYW at 26.9 Mg ha−1 plus SSA.
Means within a column followed by same letter were not significantly different by Fisher's LSD test at P ≤ 0.05.
Data are presented as mean ± standard deviation of four replications.
Discussion
A variety of biological soil amendment-based tactics for soil-borne pathogen control have been examined in previous research as potential alternatives to CSF. In Florida, ASD conducted using a combination of liquid M with CPL has provided excellent control of soil-borne pathogens and plant-parasitic nematodes in raised-bed vegetable production systems including tomato, eggplant and pepper (Butler et al., Reference Butler, Kokalis-Burelle, Muramoto, Shennan, McCollum and Rosskopf2012a, Reference Butler, Rosskopf, Kokalis-Burelle, Albano, Muramoto and Shennan2012b; Di Gioia et al., Reference Di Gioia, Ozores-Hampton, Hong, Kokalis-Burelle, Albano, Zhao, Black, Gao, Wilson, Thomas, Moore, Swisher, Guo and Rosskopf2016; Guo et al., Reference Guo, Di Gioia, Zhao, Ozores-Hampton, Swisher, Hong, Kokalis-Burelle, DeLong and Rosskopf2017). However, the food safety concern as well as environmental issues pertaining to high P content in animal manure-based products led to the search for alternatives to CPL for ASD application in Florida (Islam et al., Reference Islam, Doyle, Phatak, Millner and Jiang2005).
A cumulative anaerobic condition of 50,000 mVhr has been suggested previously as a potential threshold for achieving adequate control of Verticillium in CA strawberry production (Shennan et al., Reference Shennan, Muramoto, Koike, Bolda, Daugovish, Mochizuki, Klonsky, Rosskopf, Kokalis-Burelle and Butler2011). In our previous study, cumulative anaerobic conditions of 73,315 and 87,894 mVhr, resulted from the ASD0.5 treatment at Citra and Immokalee, respectively (Guo et al., Reference Guo, Di Gioia, Zhao, Ozores-Hampton, Swisher, Hong, Kokalis-Burelle, DeLong and Rosskopf2017). In another previous study, ASD treatment with 22 Mg ha−1 CPL and 13.9 m3 ha−1 M led to cumulative soil anaerobiosis nearing 50,000 mVhr which resulted in significantly lower root gall index values and similar yields to those achieved using CSF (Di Gioia et al., Reference Di Gioia, Ozores-Hampton, Hong, Kokalis-Burelle, Albano, Zhao, Black, Gao, Wilson, Thomas, Moore, Swisher, Guo and Rosskopf2016). In the current study, considerably lower cumulative anaerobic soil conditions of 17,219 (Citra site) and 19,445 (Immokalee site) mVhr were achieved with the ASD0.5 application, followed by CYW1 + M 5125 and 7636 mVhr in Citra and Immokalee, respectively, and CYW0.5 + M 2017 and 4779 mVhr in Citra and Immokalee, respectively. Very low or no anaerobic condition was observed in SSA, CYW1 and CYW1 + SSA as expected, as these treatments are not considered to be ASD, but rather amendment with compost and commercial microbial products. Environmental conditions, including rainfall and soil temperature affect soil anaerobic conditions (Butler et al., Reference Butler, Shrestha, Dee, Inwood, McCarty, Ownley and Rosskopf2014b). The mean, minimum and maximum air temperatures in Immokalee were slightly higher in this study relative to the previous trials (Di Gioia et al., Reference Di Gioia, Ozores-Hampton, Hong, Kokalis-Burelle, Albano, Zhao, Black, Gao, Wilson, Thomas, Moore, Swisher, Guo and Rosskopf2016; Guo et al., Reference Guo, Di Gioia, Zhao, Ozores-Hampton, Swisher, Hong, Kokalis-Burelle, DeLong and Rosskopf2017), while the mean soil temperatures were also higher at both locations compared with our previous experiments (Di Gioia et al., Reference Di Gioia, Ozores-Hampton, Zhao, Thomas, Wilson, Li, Hong, Albano, Swisher and Rosskopf2017). Regarding the increased fruit yield, cumulative soil anaerobic condition may not be the only contributor to the efficacy of the ASD process. Despite the low-to-intermediate level of root-knot nematode infestation in Citra soils, other soil-borne pathogens were not assessed in this study. The approaches to ASD, i.e., ASD0.5, CYW1 + M and CYW0.5 + M reduced root galling and produced higher marketable and total yields than untreated control, with equivalent yield to conventional CSF, without generating levels of cumulative anaerobicity assumed to be necessary for broad-spectrum pest control. Application rate, type of carbon sources and corresponding changes in the soil physical, chemical and biological properties may be more important for positive plant responses (Liu et al., Reference Liu, Kong, Cui, Zhang, Wang, Cai and Huang2016; Mazzola et al., Reference Mazzola, Muramoto and Shennan2018).
Literature suggests that soil microbial communities play a pivotal role in managing soil-borne pathogens including root-knot nematodes, with both beneficial and pathogenic soil microorganisms being highly sensitive to changes in management practices (Mazzola, Reference Mazzola2004; Raaijmakers et al., Reference Raaijmakers, Paulitz, Steinberg, Alabouvette and Moënne-Loccoz2009; Paudel et al., Reference Paudel, Carpenter-Boggs and Higgins2016). Positive impacts of ASD on beneficial microorganisms including Trichoderma, actinomycetes and Bacillus have been reported (Shrestha et al., Reference Shrestha, Auge and Butler2016). The microbial community composition may also be associated with nutrient availability in the soil as affected by organic amendments (Guo et al., Reference Guo, Zhao, Rosskopf, Di Gioia, Hong and McNear2018). In the present study, both root-knot nematodes and non-parasitic nematodes were monitored to help better characterize the effects of soil amendments on the tomato production system. The soil nematode density increased as the growing season progressed at Citra, with little change in Immokalee. Interestingly, soil populations of both root-knot nematodes and non-parasitic nematodes at Citra increased during the late season as compared with their populations at mid-season and prior to transplanting immediately after the soil treatments were completed. Similar results were reported by Di Gioia et al. (Reference Di Gioia, Ozores-Hampton, Hong, Kokalis-Burelle, Albano, Zhao, Black, Gao, Wilson, Thomas, Moore, Swisher, Guo and Rosskopf2016). Differences in root-knot nematode infestation level in roots, root gall index and root condition rating among treatments were more evident than the soil nematode population estimates, and demonstrated the effectiveness of ASD in suppressing root-knot nematodes and maintaining root health. Using approaches of ASD including CPL and CYW, the plant stem diameters and root condition ratings reflected an overall plant growth and health improvement by ASD.
There is a lack of consistent weed suppression by ASD (Di Gioia et al., Reference Di Gioia, Ozores-Hampton, Hong, Kokalis-Burelle, Albano, Zhao, Black, Gao, Wilson, Thomas, Moore, Swisher, Guo and Rosskopf2016; Shrestha et al., Reference Shrestha, Auge and Butler2016; Guo et al., Reference Guo, Di Gioia, Zhao, Ozores-Hampton, Swisher, Hong, Kokalis-Burelle, DeLong and Rosskopf2017), particularly relative to nutsedge species. The levels of cumulative anaerobicity achieved in this study did not result in effective weed control, although CYW1 tended to suppress broadleaf weeds in the Immokalee trial. The level of cumulative anaerobicity may not be the most significant driver relative to weed control, particularly of nutsedge, which may be more highly correlated to organic acid accumulation, carbon source and C:N ratio (Shennan et al., Reference Shennan, Muramoto, Koike, Baird, Fennimore, Samtani, Bolda, Dara, Daugovish, Lazarovits, Butler, Rosskopf, Kokalis-Burelle, Klonsky and Mazzola2017; Shrestha et al., Reference Shrestha, Rosskopf and Butler2018). However, with the application of herbicide to all treatments in the current study, treatment effects for weed control could not be clearly compared. Use of fomesafen for tomato production in Florida has been reported to result in variable nutsedge control (Boyd, Reference Boyd2015), which is reflected here in this study, with poor control at the Citra site and more effective nutsedge control in Immokalee. Previous studies conducted using ASD and halosulfuron-methyl resulted in significantly better nutsedge control than using ASD without an herbicide (Guo et al., Reference Guo, Di Gioia, Zhao, Ozores-Hampton, Swisher, Hong, Kokalis-Burelle, DeLong and Rosskopf2017) and this may be a better herbicide partner for ASD in tomato production, although the effects of the two herbicides have not yet been studied simultaneously.
In both locations, ASD applied using CPL (ASD0.5) and CYM (CYW1 + M) resulted in greater total and MY than the untreated control, and the yields were comparable to CSF. Similar results were also found in CYW0.5 + M and CYW1 + SSA in Immokalee. Interestingly, there appeared to be a benefit of combining CYW1 and SSA when compared with CYW1 or SSA applied alone. CYW as a soil amendment could help improve soil water and nutrient holding capacity to enhance nutrient availability especially under sandy soil conditions (Butler et al., Reference Butler, Rosskopf, Kokalis-Burelle, Albano, Muramoto and Shennan2012b; Ozores-Hampton et al., Reference Ozores-Hampton, Stansly and Salame2011; Guo et al., Reference Guo, Di Gioia, Zhao, Ozores-Hampton, Swisher, Hong, Kokalis-Burelle, DeLong and Rosskopf2017). It was likely that the effects of CYW on soil fertility and availability may have been more pronounced in Immokalee due to the greater percentage of sand in the Immokalee soil in contrast to the Citra location (Novak et al., Reference Novak, Busscher, Laird, Ahmedna, Watts and Niandou2009; Ozores-Hampton et al., Reference Ozores-Hampton, Stansly and Salame2011), while the combination of the CYW and SSA, which includes a weekly and monthly application of some of the SSA components, could contribute to enhanced nutrient availability.
Introducing non-native microbial amendments to crop production systems often results in inconsistency with respect to plant growth and yield promotion (Mazzola, Reference Mazzola2007). A positive impact on crop performance by disease suppression or by a growth-promoting microbes is the result of complex interactions between pathogen, host plant, soil biological parameters, as well as the environment. In many cases, the external biological control agent or beneficial bacterial inoculants fail to establish in the new physical environment (Handelsman and Stabb, Reference Handelsman and Stabb1996; Mazzola, Reference Mazzola2007). Despite the expected positive impact on crop health, SSA alone produced higher yields than the control at Immokalee, but did not differ from control yields at Citra. The benefit of combining SSA with CYW may have been a result of providing a substrate upon which the introduced bacterial communities were able to persist. Applications of organic amendments, such as composts and animal manures, increase soil organic matter that will lead to an array of positive impacts on soil physical, chemical and biological processes and properties, and contribute to soil-borne pathogen suppression (Tester, Reference Tester1990; Darby et al., Reference Darby, Stone and Dick2006). Although the ASD treatments (ASD0.5, CYW1 + M and CYW0.5 + M) in this study did not result in high anaerobicity, these treatments likely provided substrate and carbon source for stimulating microbial communities and activities. Hence, overall improvement of soil quality and nutrient availability may have contributed to greater yields in treatments with the ASD approach and CYW1 + SSA (Ozores-Hampton et al., Reference Ozores-Hampton, Stansly and Salame2011, Reference Ozores-Hampton, McSorley and Stansly2012; Butler et al., Reference Butler, Kokalis-Burelle, Albano, McCollum, Muramoto, Shennan and Rosskopf2014a; Di Gioia et al., Reference Di Gioia, Ozores-Hampton, Hong, Kokalis-Burelle, Albano, Zhao, Black, Gao, Wilson, Thomas, Moore, Swisher, Guo and Rosskopf2016, Reference Di Gioia, Ozores-Hampton, Zhao, Thomas, Wilson, Li, Hong, Albano, Swisher and Rosskopf2017).
In this study, little influence of ASD and other soil biological treatments was found on tomato fruit quality attributes including color, firmness, pH, TSS, TA and DM content as compared with CSF and control in the two sites, except for some differences in color and TA in Immokalee. The difference in color and TA observed in Immokalee were consistent with field observations at the first harvest. Plants were more vigorous and had more abundant foliage with delayed ripening in ASD and CSF which affected color and TA of fruit in the first harvest. The lack of significant effects of these biological soil treatments on fruit quality attributes was consistent with previous findings by Guo et al. (Reference Guo, Di Gioia, Zhao, Ozores-Hampton, Swisher, Hong, Kokalis-Burelle, DeLong and Rosskopf2017) and Di Gioia et al. (Reference Di Gioia, Ozores-Hampton, Hong, Kokalis-Burelle, Albano, Zhao, Black, Gao, Wilson, Thomas, Moore, Swisher, Guo and Rosskopf2016).
The results of the current study support the potential of employing CYW as an alternative to CPL in the application of ASD. Although yard waste composts are commonly used as soil amendments (Bonilla et al., Reference Bonilla, Vida, Martinez-Alonso, Landa, Gaju, Cazorla and de Vicente2015), employing CYW as an amendment for ASD treatment had not been explored previously. Despite the considerably lower anaerobicity achieved by the ASD treatment with CYW compared with that achieved with CPL, the addition of a labile carbon source, such as M, combined with soil saturation significantly improved the effect of CYW on tomato productivity. Additionally, the additive and/or synergistic effects of the application of CYW, M and SSA in maintaining plant health and improving crop yield demonstrated that this treatment deserves further examination. As CYW may be more readily available and possess no food safety concerns compared with CPL, the combination could be a promising substitute for CPL in implementing ASD. More studies are warranted to understand modified carbon input requirements, the soil and environmental factors contributing to the development of anaerobic conditions in ASD with CYW, and the ultimate effects on soil-borne pathogen suppression, soil fertility and plant health.
Conclusions
There is a critical need to seek sustainable practices for managing soil-borne pests and pathogens and improving crop health in tomato production which are environmentally sound, economically feasible and socially acceptable. As an alternative to CPL for ASD, results from the present study support the conclusion that CYW could be a potential replacement for CPL in maintaining the effect of ASD with M. Overall, the two application rates of CYW examined, i.e., 26.9 and 13.45 Mg ha−1, led to comparable crop performance, when used as an alternative to CPL in combination with M for ASD implementation. In general, these soil biological treatments did not cause losses in the fruit quality attributes assessed and resulted in yields that were comparable to those achieved with a commonly used CSF. Additional studies are warranted to further investigate the potential environmental conditions that impact development of soil anaerobicity, the underlying mechanisms associated with disease suppressiveness under both high and low cumulative anaerobicity, and the impacts of these parameters on plant health and crop productivity as a result of ASD applied with various soil amendments. More integrated research needs to be conducted for developing effective, consistent, economically viable approaches for synergistic use of different types of amendments and their impacts on soil microbial communities that support plant health.
Supplementary material
The supplementary material for this article can be found at https://doi.org/10.1017/S1742170518000571
Acknowledgements
Current research project was funded in part by the US Department of Agriculture, Agricultural Research Service, Areawide Project on Anaerobic Soil Disinfestation. We greatly appreciate and acknowledge the technical assistance provided by Alexander Tasi, Buck Nelson, Dustin Huff, Gilma Castillo, Melissa Edgerly, Michael Hensley, Peter D'Aiuto, Shawron Weingarten, Thaisa Cantele, Wesley Schonborn, Kaylene Sattanno and Zachary Black. Mention of commercial names in this publication is solely for the purpose of providing exact information and does not imply recommendation or endorsement by the US Department of Agriculture.