Arsenic is a naturally occurring element that is present in inorganic and organic forms and widely distributed throughout the environment. Due to its widespread presence in soil and rock, arsenic is a common contaminant of air, water and food(1). Exposure to arsenic, particularly inorganic arsenic, has been associated with several adverse health outcomes, including developmental, cancer and cardiovascular outcomes(Reference Abdul, Jayasinghe and Chandana2). Despite this evidence, there are currently no global consensus guidelines for exposure to arsenic in diet. In 1988, the WHO first established a provisional tolerable total arsenic level (from all sources) of 2·1 μg/kg-body weight (bw)/d. In 2011, this guideline value was withdrawn, as it was not considered protective of human health(3). Given its environmental ubiquity, associations with adverse health outcomes, and lack of a global consensus exposure guideline, arsenic exposure represents a major public health concern.
In the USA, low air concentrations(1) and established enforceable public drinking water standards(4) suggest low levels of exposure through these routes, leaving diet as the predominant source of arsenic exposure(Reference Xue, Zartarian and Wang5,Reference Kurzius-Spencer, Burgess and Harris6) . Diet presents the potential for exposure to organic (commonly found in shellfish) and inorganic (commonly found in fruits, vegetables and grains, such as rice) arsenic(1). Importantly, the magnitude of arsenic levels in diet is impacted by the global distribution of foods coupled with the variability in arsenic content in soil and water. Although there are few federal regulations pertaining to arsenic content in foods or guidance for tolerable levels of exposure to arsenic in diet, the US Environmental Protection Agency (EPA) uses a daily reference dose for inorganic arsenic of 0·3 μg/kg-bw/d(7). This reference dose encompasses arsenic exposure from all sources – including diet, drinking water and occupation – and represents a dose likely to be without an appreciable risk for non-cancer effects.
Even though diet is considered the primary source of arsenic exposure in the USA, little is known about how this route of exposure may influence the development of birth defects in humans. From limited national data, it is estimated that US women of reproductive age are exposed to approximately 0·16–0·23 μg/kg-bw/d of total arsenic and 0·03–0·08 μg/kg-bw/d of inorganic arsenic through diet(Reference Suhl, Conway and Rhoads8,Reference Jara and Winter9) . These diet-only estimates are below the withdrawn WHO tolerable total arsenic level and the current US EPA reference dose for tolerable inorganic arsenic level from all sources. Nonetheless, inorganic arsenic has been shown to cross the placenta, with animal models demonstrating accumulation of arsenic in fetal tissues(Reference Lindgren, Danielsson and Dencker10,Reference Devesa, Adair and Liu11) . Furthermore, some animal studies have reported birth defects in offspring following maternal high-dose inorganic arsenic exposure(1). Several human studies were identified that examined maternal arsenic exposure from a variety of sources and birth defects in offspring(Reference Brender, Suarez and Felkner12–Reference Jin, Zhang and Li22). However, only one study reported associations specific to arsenic exposure in diet, and this study was limited to associations with orofacial clefts (OFC)(Reference Suhl, Leonard and Weyer17).
Currently, the suggested, but limited, animal evidence and the paucity of human evidence poses challenges in determining how arsenic in diet in the USA translates to risk of birth defects in humans. As such, we estimated associations between maternal levels of total (all forms) and inorganic arsenic in diet and a spectrum of selected major structural birth defects using maternal food frequency reports collected in the multisite, population-based National Birth Defects Prevention Study (NBDPS).
Materials and methods
Study design
We used data collected from the NBDPS – a case–control study of environmental and genetic risk factors for over thirty major structural birth defects. The NBDPS methodology has been detailed previously(Reference Reefhuis, Gilboa and Anderka23). Case children with estimated dates of delivery (EDD) during October 1997–December 2011 were identified from ten US sites (Arkansas, California, Georgia, Iowa, Massachusetts, New Jersey, New York, North Carolina, Texas and Utah); delivery outcomes included live births (all sites), stillbirths (six sites) and elective terminations (five sites). Abstracted medical record data for case children were reviewed by clinical geneticists to confirm diagnosis, and those diagnosed with birth defects with a known cause (e.g. chromosomal disorders) were excluded(Reference Rasmussen, Olney and Holmes24). Eligible case children were classified as ‘isolated’ (no other additional major birth defects in a different organ system) or ‘multiple’ (one or more additional, major unrelated birth defects in a different organ system). Control children were a random sample of live births without birth defects, selected from hospital delivery logs or birth certificate files during the same time frame and geographic areas as case children. Approximately 100 control children were recruited per site per year, allowing a minimum of a 1:1 ratio between control children and children with an NBDPS-eligible birth defect.
Mothers of case and control children completed a telephone interview 6 weeks through 24 months following their EDD(Reference Reefhuis, Gilboa and Anderka23). The interview asked mothers to report sociodemographic, medical and prenatal care, family history, and environmental and lifestyle information, including diet. A fifty-eight-item FFQ adapted from the Willet FFQ(Reference Willett, Reynolds and Cottrell-Hoehner25) was administered to collect dietary information for the year prior to pregnancy. Additionally, the NBDPS interview collected information on cereal consumption for the period 3 months before through the end of pregnancy. The NBDPS protocol was approved by the institutional review board at the US Centers for Disease Control and Prevention and each NBDPS site. A waiver of signed informed consent was approved for interview data collection.
Analytical sample
In total, 11 829 mothers of control children and 32 017 mothers of case children participated in the NBDPS. On average, mothers of control and case children were interviewed 9·5 and 11·7 months, respectively, after their EDD(Reference Tinker, Gibbs and Strickland26). Our current analysis comprised mothers of 11 157 control children and 15 524 case children with isolated, non-cardiac birth defects who completed the NBDPS FFQ; only case children classified as isolated were included to improve the homogeneity of birth defect phenotypes examined. Among those with a completed FFQ, 271 control and 495 case mothers were excluded due to reporting consumption of <500 or >5000 calories/d, and an additional 440 control and 621 case mothers were excluded due to not reporting BMI, leaving data for 10 446 control children and 14 408 case children with isolated, non-cardiac birth defects in our analytical sample (Table 1).
Table 1 Frequency of controls and selected isolated birth defects, US National Birth Defects Prevention Study, 1997–2011
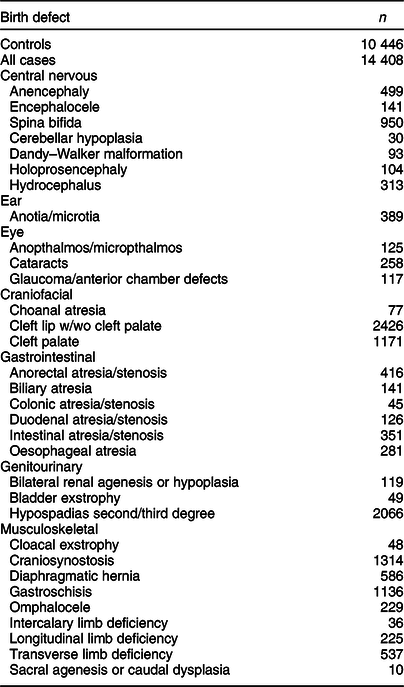
Fewer controls were available for anencephaly, encephalocele, and spina bifida (n 10 352), glaucoma and cataracts (n 8854), cleft lip w/wo cleft palate and cleft palate (n 10 315), and hypospadias (n 5314).
Dietary arsenic exposure assessment
For our primary analyses, we estimated maternal exposure to total and inorganic arsenic in diet for the year prior to pregnancy using responses to the NBDPS FFQ. The total arsenic content in each FFQ item was determined using total arsenic concentrations reported in the US Food and Drug Administration Total Diet Study (TDS). The TDS monitors numerous contaminants, including total arsenic, in a variety of foods commonly consumed in the USA. To determine contaminant concentrations, foods were sampled from retail outlets throughout four US regions (Northeast, North Central, South and West), prepared as they would be consumed and analysed using hydride generation-atomic absorption analysis(27). Two contemporaneous TDS reports were available (1991–2005(28) and 2006–2013(29)) for EDD included in the NBDPS. Because the NBDPS collected dietary consumption for the year before pregnancy, to retain the same TDS estimates for mothers with EDD within the same calendar year, mothers with EDD from 1997–2006 were linked to 1991–2005 estimates, and those with EDD from 2007–2011 were linked to 2006–2013 estimates.
Each FFQ item was linked to all corresponding TDS items. For FFQ items that were linked to multiple similar TDS items, an overall mean total arsenic concentration for the FFQ item was estimated averaging the concentrations for each relevant TDS item. For example, maternal egg consumption was collected in the FFQ, and the 1991–2005 TDS provided arsenic concentration estimates for fried eggs, scrambled eggs and boiled eggs separately. As such, the total arsenic concentration estimate for eggs was the overall mean total concentration for all three egg dishes. Additionally, available concentration estimates sometimes differed between TDS versions (e.g. the 1991–2005 TDS included three egg dishes, whereas the 2006–2013 included two egg dishes). Total arsenic concentration estimates for each FFQ item were calculated using all available data from each TDS version. Calculated total arsenic concentration estimates for FFQ items that differed on available data between TDS versions tended to be similar. Because the TDS assumes concentration estimates below the limit of detection (LOD) to be 0 μg/g, all such estimates were assumed to be 0 μg/g in our study. TDS concentration estimates for total arsenic were available for fifty-five of the fifty-eight FFQ items (see online supplemental Table A.1).
We applied a similar approach to assign maternal exposure to inorganic arsenic in diet using available reported estimates(Reference Schoof, Yost and Eickhoff30). Because these available inorganic arsenic concentration estimates applied one-half the LOD for food items below the limit, all such estimates for inorganic arsenic were assumed to be one-half the LOD in our study. Concentration estimates for inorganic arsenic were available for twenty-four of the fifty-eight FFQ items (Table A.1).
Grams of each FFQ item consumed/d by the mother were estimated using the reported number of servings consumed and grams/serving for that item as reported by the US Department of Agriculture Food Composition Database(31). The estimated grams consumed of each FFQ item/d was multiplied by the relevant arsenic concentration estimate to determine arsenic consumed/FFQ item/d and converted to μg/d. Summing the arsenic concentration estimates for each FFQ item produced overall total and inorganic arsenic consumption/d (μg/d) estimates for each mother. Using maternal reported pre-pregnancy body weight, estimates in μg/kg-bw/d for total and inorganic arsenic were calculated. Four additional items were included in the FFQ from 2006–2011 (soya milk; Hawaiian punch, lemonade, or other fruit drinks; tofu, tempeh or soya burgers; biscuits, scones, croissants and muffins). Because consumption estimates for these items were not available for mothers from 1997–2005, they were not included in arsenic consumption/d estimates.
For a secondary analysis described below, we added estimates for total arsenic in breakfast cereals consumed. The TDS included total arsenic estimates for oatmeal, wheat cereal/farina, fruit-flavoured, sweetened cereal, shredded wheat cereal, raisin bran cereal, crisped rice cereal, granola cereal and oat ring cereal. Cereals reported were reviewed and matched to corresponding TDS total arsenic concentration estimates. For consistency with the exposure assessment used in our primary analyses, we applied conservative cereal definitions in matching reported cereals. For example, although several cereals are oat-based and may contain similar ingredients to oat rings, only cereals that were oat-based and ring-shaped were included in estimates for oat ring cereals. To more closely correspond with maternal pre-pregnancy FFQ responses, only responses to cereals consumed during the 3 months prior to conception were included in the total arsenic consumption estimates. The resultant total arsenic consumption estimate was added to that from the primary analysis. Additionally, no concentration estimates for inorganic arsenic were available for cereals; therefore, secondary analyses including cereal consumption were only completed for total arsenic exposure. In all analyses, maternal exposure to arsenic in diet was examined without adjusting for consumption of arsenic through drinking water due to its generally low concentrations in public drinking water throughout much of the USA and the low proportion (8 %) of NBDPS case and control mothers who reported using well water(Reference Suhl, Conway and Rhoads8).
Child and maternal characteristics
Table 2 lists the child and maternal characteristics examined. Hospital or birth records were used to collect child sex (male and female) and maternal age at delivery (<20, 20–24, 25–29, 30–34, 35–39 and ≥40 years). The NBDPS interview was used to collect data on child plurality (1 and 2+) and first-degree family history of same birth defect (yes and no), along with maternal education at delivery (0–8, 9–11, 12, 13–15 and ≥16 years), race/ethnicity (non-Hispanic White, non-Hispanic Black, Hispanic and other), pre-pregnancy BMI (<18·5, 18·5–24·9, 25–29·9 and ≥30·0 kg/m2), gravidity (0, 1 and ≥2), folic acid supplementation during the respective critical exposure period (1 month before (B1) through the first month following conception (M1) for neural tube defects (NTD: comprised of anencephaly, spina bifida and encephalocele) or B1 through the third month following conception (M3) for non-NTD) (yes and no), pre-pregnancy dietary folate equivalents (<600 and ≥600 µg/d), tertiles of total energy intake based on the distributions among control mothers (low (<1067·5 calories/d), middle (1067·5–<1486·6) and high (≥1486·6)), alcohol consumption (no drinking, drinking with no binge episodes and drinking and ≥1 binge episode) and tobacco smoke exposure (no active or passive smoking, active smoking only, passive smoking only and active and passive smoking) during the defect-relevant critical exposure period (B1-M1 and B1-M3) and NBDPS site (Arkansas, California, Georgia, Iowa, Massachusetts, New Jersey, New York, North Carolina, Texas and Utah). All child and maternal characteristics except for pre-pregnancy BMI, which was incorporated into the arsenic exposure estimates, were considered as covariates in multivariable analysis. Additionally, mothers who reported use of folate antagonist medications were excluded from NTD analyses.
Table 2 Distributions of child and maternal characteristics among controls and isolated case children, US National Birth Defects Prevention Study, 1997–2011
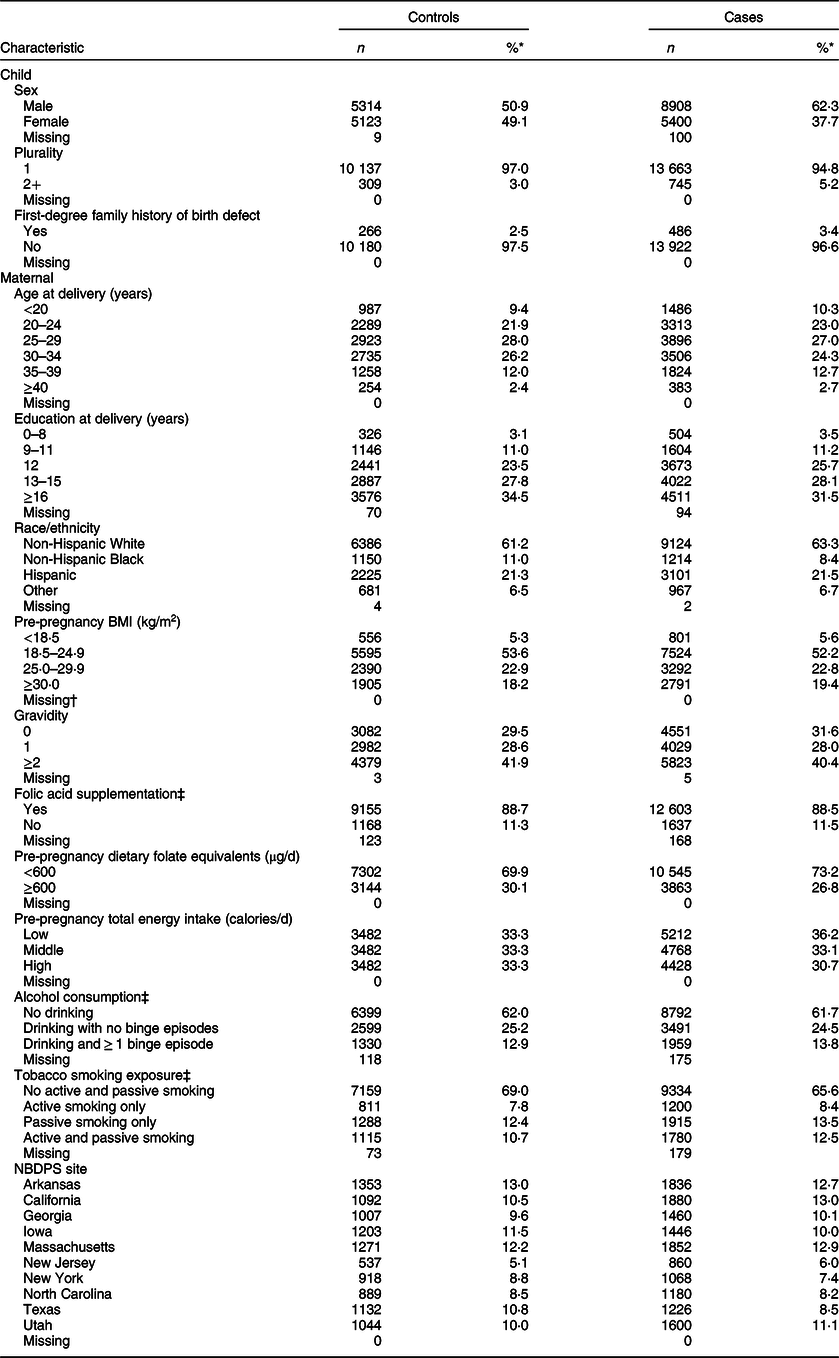
NBDPS, National Birth Defects Prevention Study.
* Due to rounding, proportions may not total to 100.
† Four hundred and eighty-nine control and 911 case mothers were excluded from analyses due to not reporting BMI.
‡ During the period 1 month before (B1) through the third month following (M3) conception.
Primary analyses
We estimated proportions for selected characteristics for case and control children and mothers, as well as maternal exposures. We estimated the mean, median and standard deviation for both maternal pre-pregnancy total and inorganic arsenic in diet (μg/kg-bw/d). In association analyses, arsenic in diet was examined as categorical tertiles of exposure (low, middle and high) based on cut-offs of the arsenic distributions among control mothers. Although the number of available control mothers was constant for most birth defects, fewer control children were available for certain defects – OFC (Utah did not include OFC in 2003), glaucoma and cataracts (defects not included in NBDPS prior to 2000), NTD (mothers who reported use of folate antagonist medications were excluded), and hypospadias (only included male controls). As such, defect-specific tertile cut-offs using the relevant, available data for control children and mothers were estimated for these birth defects.
Crude and adjusted OR and 95 % CI were estimated using unconditional logistic regression analysis to examine associations between tertiles of maternal pre-pregnancy arsenic exposure in diet and non-cardiac, isolated birth defects in children. For all analyses, the low tertile of exposure was used as the reference category. A multivariable model for each arsenic exposure–birth defect pairing was constructed using a change-in-estimate approach. Each individual covariate was included in a model with the arsenic exposure variable of interest; if the covariate altered the crude OR estimate by >10 %, it was included in the final multivariable model for that pairing. Analyses were conducted for an arsenic exposure–birth defect pairing when at least five case mothers were included in the low and middle or high tertile of arsenic exposure. Additionally, when sparse data resulted in model convergence failure, Firth’s logistic regression(Reference Firth32) was used to estimate OR and 95 % profile likelihood CI. Case and control children or mothers with missing data for one or more characteristic or exposure were removed from adjusted analysis.
Secondary analyses
We conducted three sets of secondary analyses. The first set restricted analyses for three maternal covariates – diabetes, plurality and maternal folate status. We excluded case and control children whose mothers reported pre-pregnancy diabetes, given that pre-pregnancy diabetes is strongly associated with some birth defects(Reference Correa, Gilboa and Besser33,Reference Tinker, Gilboa and Moore34) . Twinning is also associated with some birth defects(Reference Dawson, Tinker and Jamieson35); therefore, we excluded case and control children from multiple pregnancies. Folate is necessary in arsenic metabolism, and studies suggest low folate intake may impair arsenic metabolism(Reference Gamble, Liu and Ahsan36,Reference Gamble, Liu and Ahsan37) . The influence of folate intake on arsenic metabolism was examined by restricting to mothers with reported deficient folate intake in early pregnancy. A dichotomous variable was created by combining responses for folic acid supplementation and dietary folate intake. Mothers who reported no folic acid supplementation and pre-pregnancy dietary folate intake <600 μg/d were considered to have deficient folate intake.
The second set of analyses examined interaction between maternal arsenic exposure in diet and maternal tobacco smoke exposure during the relevant critical period, as the high levels of arsenic in cigarettes may influence birth defect development or arsenic exposure. To examine potential effect modification, smoking was examined as a dichotomous variable (none, any (active or passive)). Effect modification on the additive scale was evaluated by estimating relative excess risk due to interaction (RERI) and bootstrap 95 % CI for the middle and high tertiles of arsenic exposures. RERI estimates equal to 0 indicate the absence of an interaction effect on the additive scale; effect modification on the additive scale was considered to be present if the 95 % CI for RERI estimates for the either middle or high tertile excluded zero.
The third set of secondary analyses focused on examining high-dose arsenic exposure, maximum values reported in the TDS and adding an additional dietary exposure source. For analyses of high-dose exposure, we initially defined high-dose exposure as above the withdrawn WHO and current US EPA tolerable arsenic guidelines. However, due to sparse data for high exposure in our analytical sample, we instead defined high-dose exposure to be the 90th percentile of arsenic exposure among all control mothers. We also re-evaluated our primary analyses by using the maximum reported total arsenic concentration value for foods reported in the TDS. Additionally, analyses for total arsenic consumption were expanded to include reports of cereal consumption during the 3 months prior to conception. All primary and secondary analyses were conducted using SAS v. 9.4(38).
Results
The distribution of covariates for control and all case children and their mothers are shown in Table 2. Distributions of covariates for each non-cardiac defect examined are listed in Tables A.2–A.6. The proportion of missing responses observed for each covariate was less than 5 %.
Arsenic exposure in diet
Maternal mean estimates were similar between control and all case children for total (Table 3) and inorganic arsenic (Table 4) in diet, although the distributions were strongly positively skewed for each exposure (total arsenic skewness: controls = 5·7, cases = 9·7; inorganic arsenic skewness: controls = 3·6, cases = 4·1). Median estimates for total arsenic ranged from 0·06 (colonic atresia/stenosis) to 0·18 (cloacal exstrophy) μg/kg-bw/d; median estimates for inorganic arsenic ranged from 0·05 (Dandy–Walker malformation) to 0·09 (sacral agenesis or caudal dysplasia) μg/kg-bw/d. The estimated tertiles using all control mothers for total (low = <0·07, middle = 0·07–0·21 and high = ≥0·22 μg/kg-bw/d) and inorganic (low = <0·05, middle = 0·05–0·07 and high = ≥0·08 μg/kg-bw/d) arsenic were similar to those used for certain defects (OFC, glaucoma, cataracts, NTD and hypospadias) as described in primary analyses. Sacral agenesis or caudal dysplasia included less than five case children in the low tertile and was excluded from association analysis.
Table 3 Distribution of total arsenic (μg/kg-bw/d) for controls and isolated cases, US National Birth Defects Prevention Study, 1997–2011
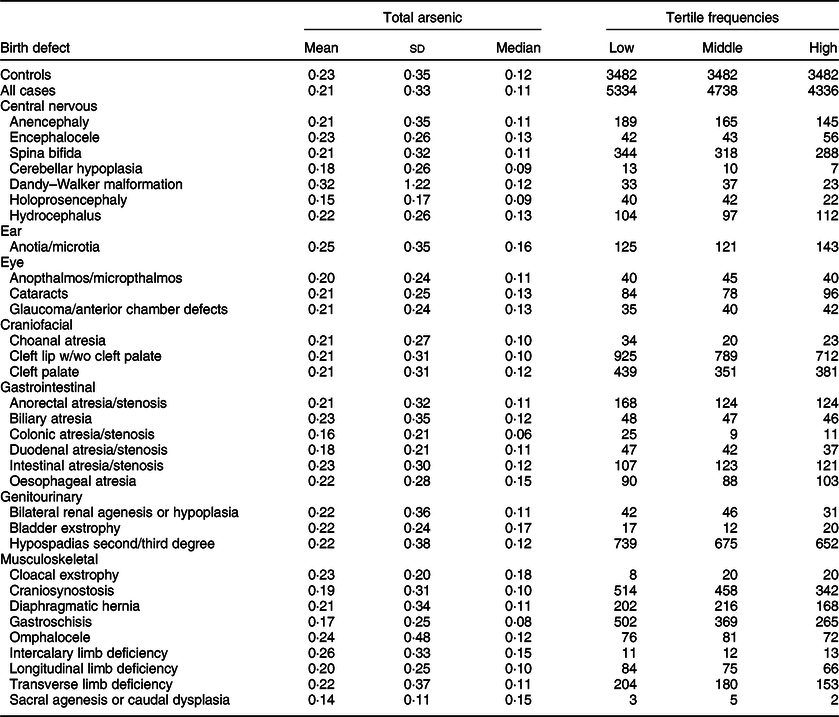
bw, body weight.
Table 4 Distribution of inorganic arsenic (μg/kg-bw/d) for controls and isolated cases. US National Birth Defects Prevention Study, 1997–2011
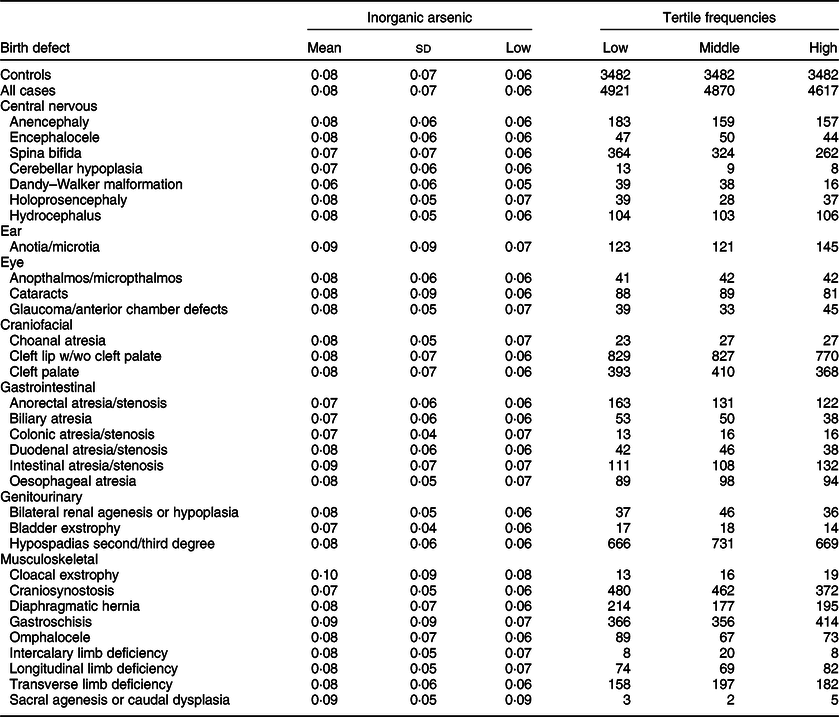
bw, body weight.
Primary analyses
Compared to the low tertile for total arsenic, threefold but imprecise associations were observed in both the middle and high tertiles for cloacal exstrophy with the 95 % CI excluding 1·0 (Table 5). Weak positive associations (adjusted ORs of 1·2–1·5), with 95 % CI including 1·0, were observed in the middle and high tertiles for intercalary limb deficiency. Additional weak associations were observed in the high tertile only for encephalocele, glaucoma/anterior chamber defects and bladder exstrophy. For maternal exposure to inorganic arsenic, associations were positive but attenuated in both the middle and high tertiles for cloacal exstrophy, with the 95 % CI including 1·0 (Table 6). A weak association with a 95 % CI that included 1·0 persisted in the high tertile for glaucoma/anterior chamber defects, whereas the association in the high tertile for intercalary limb deficiency was attenuated, but a twofold, but imprecise, association was observed in the middle tertile with the 95 % CI excluding 1·0. Also, mostly weak associations in the high and middle tertiles were observed for colonic atresia/stenosis, oesophageal atresia, bilateral renal agenesis or hypoplasia, hypospadias, and gastroschisis, in the high tertile only for choanal atresia and intestinal atresia/stenosis, or middle tertile only for encephalocele, intercalary limb deficiency, and transverse limb deficiency. Estimated associations for total or inorganic arsenic were near the null or inverse for the remainder of exposure–birth defect pairs examined.
Table 5 OR and 95 % CI for associations between maternal total arsenic exposure in diet (μg/kg-bw/d) and selected isolated non-cardiac birth defects, US National Birth Defects Prevention Study, 1997–2011
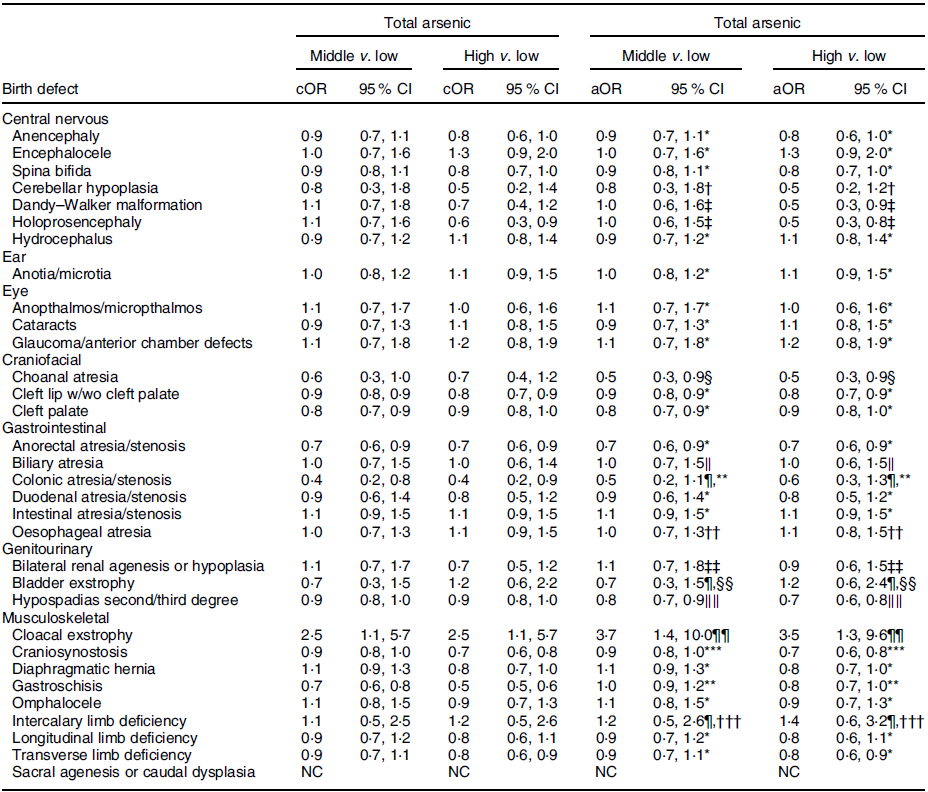
bw, body weight; cOR, crude OR; aOR, adjusted OR; NBDPS, National Birth Defects Prevention Study.
* Crude estimate.
† Adjusted for maternal tobacco smoking exposure 1 month before (B1) through third month following (M3) conception.
‡ Adjusted for maternal race/ethnicity.
§ Adjusted for maternal age at delivery.
‖ Adjusted for maternal race/ethnicity and total pre-pregnancy energy intake.
¶ Firth’s logistic regression used.
** Adjusted for maternal age and education at delivery and tobacco smoking exposure (B1–M3).
†† Adjusted for maternal age at delivery and race/ethnicity.
‡‡ Adjusted for maternal tobacco smoking exposure (B1–M3) and NBDPS site.
§§ Adjusted for maternal race/ethnicity, total pre-pregnancy energy intake and NBDPS site.
‖‖ Adjusted for maternal age at delivery and NBDPS site.
¶¶ Adjusted for child sex and maternal tobacco smoking exposure (B1–M3).
*** Adjusted for maternal age at delivery and race/ethnicity and NBDPS site.
††† Adjusted for NBDPS site.
Table 6 OR and 95 % CI for associations between maternal inorganic arsenic exposure in diet (μg/kg-bw/d) and selected isolated non-cardiac birth defects, US National Birth Defects Prevention Study, 1997–2011
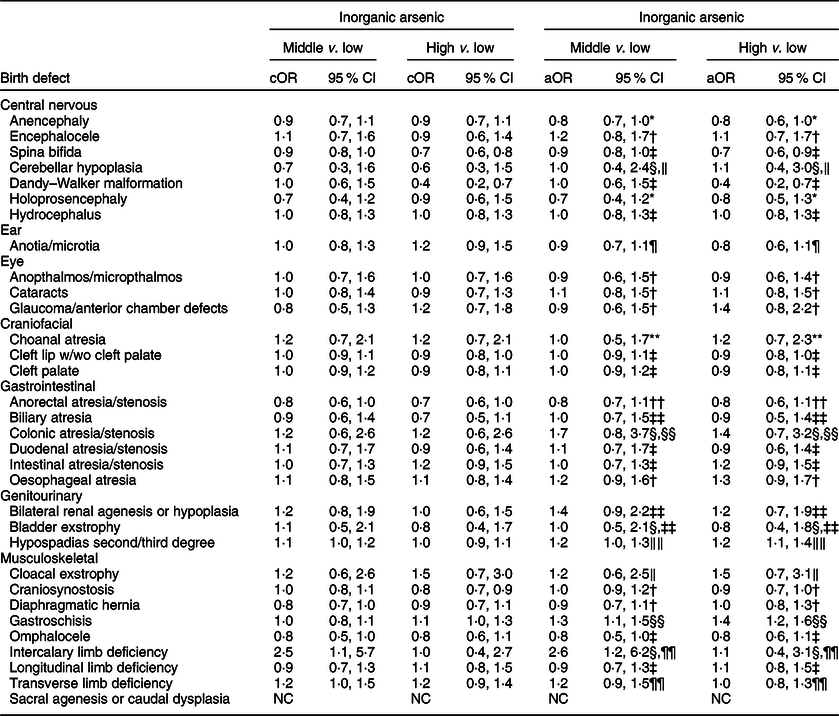
bw, body weight; cOR: crude OR; aOR, adjusted OR; NBDPS, National Birth Defects Prevention Study.
* Adjusted for maternal race/ethnicity.
† Adjusted for maternal total pre-pregnancy energy intake.
‡ Crude estimate.
§ Firth’s logistic regression used.
‖ Adjusted for maternal race/ethnicity, total pre-pregnancy energy intake, tobacco smoking exposure 1 month before (B1) through third month following (M3) conception, and NBDPS site.
¶ Adjusted for maternal race/ethnicity and NBDPS site.
** Adjusted for maternal age at delivery and race/ethnicity, pre-pregnancy dietary folate equivalents and energy intake, and NBDPS site.
†† Adjusted for pre-pregnancy dietary folate equivalents.
‡‡ Adjusted for pre-pregnancy dietary folate equivalents and total energy intake and NBDPS site.
§§ Adjusted for maternal age and education at delivery, race/ethnicity, and tobacco smoking exposure (B1–M3).
‖‖ Adjusted for maternal race/ethnicity and total pre-pregnancy energy intake.
¶¶ Adjusted for NBDPS site.
Secondary analyses
Findings for restricted analyses of selected covariates tended to be similar to those from our primary analyses. Specifically, estimated associations for total and inorganic arsenic in diet excluding mothers with reported pre-pregnancy diabetes did not differ substantively from those that included mothers with and without pre-pregnancy diabetes (Table A.7). Associations restricted to singleton pregnancies were also generally similar to those that included singleton and mulitple pregnancies (Table A.8). Only a small number of mothers were observed to have deficient folate intake (Table A.9). For total arsenic in diet, associations for mothers with deficient folate intake were imprecise, but largely similar to those observed for all mothers in the primary analyses, although those for hydrocephalus, anotia/microtia and diaphragmatic hernia were increased for both the middle and high tertiles. Associations for inorganic arsenic in diet tended to be similar to the primary analyses, except those for encephalocele, which were increased in both the middle and high tertiles.
Modestly increased RERI estimates with 95 % CI suggestive of a joint effect between smoking and arsenic exposure were observed for total arsenic and anencephaly, Dandy–Walker malformation, anotia/microtia, anopthalmos/micropthalmos, cleft palate, intestinal atresia/stenosis and transverse limb deficiency (Table A.10), as well as inorganic arsenic and cleft palate and diaphragmatic hernia (Table A.11); an inverse RERI and 95 % CI were observed for total arsenic and omphalocele. Compared to analyses of the main effect of arsenic, associations for mothers who reported smoking were increased, and often positive, whereas those for non-smoking mothers were decreased for each of the aforementioned defects except omphalocele, for which associations for non-smoking mothers were increased (Tables A.10, A.11).
Analyses for mothers classified with high-dose exposure (90th percentile of arsenic consumption among control mothers; total arsenic = 0·51 µg/kg-bw/d; inorganic arsenic = 0·16 µg/kg-bw/d) to total or inorganic arsenic in diet were imprecise with associations mostly near or below the null (Table A.12). Results for analyses using maximum concentration values for total arsenic tended to be similar to those of the primary analyses (Table A.13). Findings for estimates of total arsenic in diet that included exposure from pre-pregnancy cereal consumption tended to be similar to those from our primary analyses (Table A.14).
Discussion
We examined associations between maternal exposure to total and inorganic arsenic in diet and a spectrum of isolated non-cardiac birth defects using individual-level diet information from a multisite population-based case–control study. Associations for the middle and high tertiles of exposure, compared to the low tertile, were increased for total arsenic and cloacal exstrophy and intercalary limb deficiency, as well as for inorganic arsenic and colonic atresia/stenosis, oesophageal atresia, bilateral renal agenesis or hypoplasia, hypospadias, cloacal exstrophy and gastroschisis; however, 95 % CI for most associations included 1·0. Associations for the remainder of birth defects examined tended to be near or below the null. With the small number of available case children for several defects and the limitations in our exposure assessment, the observed associations may represent true signals between arsenic exposures and birth defects or chance findings. As such, these exploratory findings should be interpreted with caution.
The mostly weak associations observed in this study may be due to low-level arsenic exposure in diet. Although the estimated total and inorganic arsenic intake among control and case mothers was generally similar to reported estimates(Reference Jara and Winter9), they were below the previously established WHO and US EPA guidelines for total and inorganic arsenic, respectively. Furthermore, our use of a single source of arsenic exposure may have underestimated total exposure derived from multiple sources. Well water used for drinking can be an important source of arsenic exposure; however, in the NBDPS, a low proportion (8 %) of mothers reported using well water as their drinking water source(Reference Suhl, Conway and Rhoads8). Additionally, although certain occupations may present potential for high-dose exposures to arsenic, we previously identified a low proprtion (1 %) of Iowa OFC case and control mothers as occupationally exposed to arsenic(Reference Suhl, Leonard and Weyer17). Based on the low proportion of well water users and the small number of mothers occupationally exposed to arsenic, we did not consider these sources as major contributors to arsenic exposure in our current study.
Because our study is the first to examine dietary arsenic and a spectrum of birth defects, the only comparable study is our previous feasiblity study examining multi-source arsenic exposure and cleft lip with/without cleft palate (n 294) and cleft palate (n 141) among the NBDPS sample in Iowa. Although data from our current and previous studies are not completely independent, only the previously reported positive association for the high tertile of total arsenic in diet and cleft lip with/without cleft palate (adjusted OR: 1·6, 95 % CI (1·0, 2·5)) differed from the current study(Reference Suhl, Leonard and Weyer17). The discordance in findings between our previous and current studies could be due to the small sample size and reduced precision observed in the previous study. Our observed associations with some craniofacial, gastrointestinal, genitourinary and musculoskeletal defects in this exploratory US study are novel.
Our study had several limitations. Dietary assessment in the NBDPS relied on retrospective self-reports of diet during the year prior to pregnancy. The FFQ used in the NBDPS did not capture all dietary sources of arsenic and may lead to misclassification of arsenic consumption. For example, some food items that are common sources of organic (e.g. shrimp) or inorganic (e.g. rice) arsenic were not included as independent items in the FFQ. Specifically, shrimp consumption was not requested, and consumption of rice was included in a single question asking for a consumption estimate for rice and pasta combined. Another limitation was the dietary information requested was for the year prior to pregnancy; potential changes in dietary habits in early pregnancy would not have been captured. Finally, estimated arsenic from diet may be underestimated due, in part, to the exclusion of multiethnic food items and racial/ethnic variability in dietary intake amounts. Our sample was largely (84 %) comprised of non-Hispanic White and Hispanic mothers. Although the multiethnic food items included in the FFQ, and evaluated by the TDS, were largely representative of our sample, the food intakes of mothers of non-Hispanic Black and other races/ethnicities may be underestimated. Further, there may also be racial/ethnic variability in dietary intakes, such as serving sizes and individual food items within a group, unaccounted for by the FFQ that may contribute to underestimation of arsenic consumption for selected racial/ethnic groups.
Because the TDS only provided estimated concentrations of total arsenic – a composite measure of all forms of arsenic – reliance on these estimates alone may mask the effects of more harmful forms of arsenic, such as inorganic arsenic. As such, we relied on available reported inorganic arsenic concentration estimates(Reference Schoof, Yost and Eickhoff30). These estimates, however, covered fewer FFQ items than those from the TDS and may not accurately reflect inorganic arsenic concentrations throughout much of the USA, as it included only foods collected from two communities in Texas in 1997(Reference Schoof, Yost and Eickhoff30). Nonetheless, these estimates currently represent the best available data for concentrations of inorganic arsenic in foods in the USA during the time period of our study. Recently published European estimates of inorganic arsenic concentrations in food may present an additional data source for exposure assessment for future studies using more contemporary diet assessment(39). Additionally, the TDS did not begin using inductively coupled plasma MS (ICP-MS) until 2014, which postdates our study period. Our estimates of dietary total arsenic relied on concentration estimates from hydride generation-atomic absorption, which has potentially higher LOD than inductively coupled plasma MS. As such, our estimated total arsenic from diet may be an underestimation of the true arsenic burden due to the TDS reporting estimates <LOD as 0. Finally, although the NBDPS includes stillbirths ≥20 weeks gestation and elective terminations, it is possible that if arsenic is associated with early adverse pregnancy outcomes, such as miscarriages(Reference Quansah, Armah and Essumang40), we would not have observed these associations.
Our study had several strengths. The NBDPS provides a large, nationally representative comparator of US control mothers, reducing the potential for selection bias. A previous analysis comparing characteristics of control mothers to mothers of all live births at each site reported that they were similar for several maternal characteristics(Reference Cogswell, Bitsko and Anderka41). The inclusion of live births, stillbirths, and elective terminations and clinical geneticst review of clinical data to confirm diagnoses helped reduced potential biases related to case ascertainment. Use of the NBDPS FFQ responses and arsenic concentrations provided by the TDS(28,29) and published by Schoof, Yost(Reference Schoof, Yost and Eickhoff30) also allowed us to examine total and inorganic arsenic exposures from diet and a spectrum of birth defects – analyses which have not been conducted in previous studies of maternal arsenic exposure. Additional information provided by the NBDPS, such as dietary and supplemental folic acid intake, allowed us to explore the effects of folic acid on the relation between maternal dietary arsenic consumption and birth defects.
In conclusion, our study explored associations between maternal exposure to arsenic in diet and several isolated non-cardiac birth defects. Two defects showed positive associations with maternal exposure to total arsenic in diet in both the middle and high tertiles, and six defects showed positive associations with maternal exposure to inorganic arsenic in diet in both the middle and high tertiles, but most were weak. We recommend that future studies continue to use large samples with well-characterised case children; however, these studies will require improvements in dietary exposure assessment to more accurately capture maternal dietary habits during early pregnancy and require expansion in estimating arsenic content, particularly inorganic arsenic content, in food items. In addition, future studies should consider multiple sources of arsenic exposure to fully assess the impact of maternal arsenic exposure on birth defect development.
Acknowledgements
Acknowledgements: The authors thank the study participants and study staff who contributed to the NBDPS. This work was funded by the Centers for Disease Control and Prevention cooperative agreements under PA #96043, PA #02081, FOA #DD09-001, FOA #DD13-003 and NOFO #DD18-001 to the Centers for Birth Defects Research and Prevention participating in the National Birth Defects Prevention Study (NBDPS) and/or the Birth Defects Study To Evaluate Pregnancy exposureS (BD-STEPS), and grants (U01 DD001035 and U01 DD001223) awarded to the Iowa Center for Birth Defects Research and Prevention. The findings and conclusions in this manuscript are those of the authors and do not necessarily represent the official position of the Centers for Disease Control and Prevention. Financial support: This work was funded by the Centers for Disease Control and Prevention cooperative agreements under PA #96043, PA #02081, FOA #DD09-001, FOA #DD13-003 and NOFO #DD18-001 to the Centers for Birth Defects Research and Prevention participating in the National Birth Defects Prevention Study (NBDPS) and/or the Birth Defects Study To Evaluate Pregnancy exposureS (BD-STEPS), and grants (U01 DD001035 and U01 DD001223) awarded to the Iowa Center for Birth Defects Research and Prevention. Authorship: Conceptualisation and study design, J.S., K.M.C., J.J.O., P.A.R.; data collection, K.M.C., P.H.L., M.L.F., A.M.M., A.S., P.A.R.; data analysis, J.S., A.R.; initial manuscript draft, J.S. and all authors critically reviewed, commented and revised drafts of the manuscript. Ethics of human subject participation: All interviewed study participants provided informed consent. The Centers for Disease Control and Prevention Institutional Review Board (IRB), along with the IRBs for each participating site, have approved the NBDPS.
Conflicts of interest:
None.
Supplementary material
For supplementary material accompanying this paper visit https://doi.org/10.1017/S1368980022001318