Menopause, the last menstrual period, marks the end of reproductive life in women. With ageing of the population, it is estimated that worldwide 1·2 billion women will be menopausal by the year 2030(Reference Hill1). While menopause is inevitable, the age at which women reach menopause may vary depending on several factors such as geography and ethnicity. According to a meta-analysis of thirty-six studies (which included data from thirty-five countries), the overall mean age of natural menopause was 48·8 years (95% CI 48·3, 49·2) with substantial geographic variation. For example, while the mean age of menopause in the USA (49·1 years) and Asia (48·8 years) were closest to the overall mean, it was higher in Europe (50·5 years) and Australia (51·3 years) and lower in Africa (48·4 years), Latin America (47·2 years) and the Middle East (47·4 years)(Reference Schoenaker, Jackson and Rowlands2, Reference Davis, Lambrinoudaki and Lumsden3).
At birth, the human ovaries contain approximately 1 000 000 primordial follicles(Reference Edmonds and Dewhurst4). This un-replenishable pool of follicles is further reduced to about 100 000 per ovary by the time of menarche. The fate of the remaining follicles is either to develop, reach maturity and then ovulate or degenerate by the process known as atresia(Reference Sherwood5). At the perimenopausal transition stage, only about 100–1000 follicles are left in each ovary and exhaustion of the follicle pool is accompanied by permanently elevated levels of pituitary gonadotrophins and the progressive reduction in antimullerian hormone (AMH) which confirms ovarian senescence(Reference O'Connor, Holman and Wood6). The hypo-oestrogenic changes taking place during the perimenopause (menopausal transition) are a result of the interactions taking place between the hypothalamic–pituitary axis and the reproductive endocrine axis marking this irreversible decline in ovarian responsiveness(Reference Davis, Lambrinoudaki and Lumsden3).
The menopausal transition is the shift from normal reproductive life to the last menstrual period and can last for up to 10–15 years(Reference Morrison, Brinton and Schmidt7). According to the Staging of Reproductive Aging Workshop(Reference Harlow, Gass and Hall8), it is divided in two stages: early and late. The early menopausal transition is marked by changes in menstrual cycle length and is characterised by an increase in follicle-stimulating hormone (FSH) level, a decrease in AMH and inhibin B levels, while oestrogen level remains stable. The late transition is marked by oligomenorrhoea (infrequent periods) and can last for 1–3 years on average. This stage is accompanied by an increase in anovulatory cycles and also major fluctuations in hormonal levels. FSH level remains elevated while there is a consequent decrease in AMH and inhibin levels as well as oestrogen level. After the final menstrual period, ovarian ageing is marked by a decrease in antral follicular count, and termination of ovulation and menstruation. In addition, there are further declines in AMH, inhibin and oestradiol levels(Reference Burger, Hale and Dennerstein9, Reference Su and Freeman10). Ovarian ageing is also accompanied by loss of responsiveness to FSH and luteinising hormone, hence causing a disruption in the negative feedback mechanism owing to the almost negligible inhibin level and decline in oestrogen level. Consequently, the production of gonadotropin-releasing hormone is up-regulated, stimulating the release of FSH and luteinising hormone. Thus, during the initial years after menopause the level of FSH peaks and gradually declines in the last postmenopause stage(Reference Davis, Lambrinoudaki and Lumsden3).
These hormonal fluctuations as a result of the neuroendocrine and reproductive endocrine interactions influence the risk of both intermediate and long-term health outcomes associated with menopause(Reference Davis, Lambrinoudaki and Lumsden3). One of the most common intermediate sequelae of the menopause transition, vasomotor menopausal symptoms (VMS), is defined as either the presence of hot flushes and/or night sweats. VMS is reported by 40–60 % perimenopausal women and 8–80 % postmenopausal women around the world(Reference Freeman and Sherif11). The timing of onset of menopause can influence the length of the menopausal transition and hence the duration for the presence of VMS. Evidence also shows a link between an early onset of menopause and an increased risk of osteoporosis, CVD, depression and mortality. Conversely, a later age at menopause has been associated with a higher prevalence of hormone-related cancers such as breast, endometrial (uterine) and ovarian cancers(Reference Gold12). Moreover, the presence of VMS has also been associated with an increased risk of CVD(Reference Pines13). Previous studies have demonstrated a link between reproductive factors, socio-demographic factors and the onset of natural menopause, presence of VMS and risk of hormone-related cancer(Reference Kaczmarek14–Reference Lawlor, Ebrahim and Smith16). However, its relationship with diet, a modifiable risk factor, has received less attention and current evidence of association is conflicting. Therefore, the aim of this review is to give an overview of the mechanistic pathway relating diet with age at natural menopause as well as to elucidate the relationship between diet and VMS (an intermediate sequelae of menopause) in addition to the risk of hormone-dependent cancers such as breast, endometrial and ovarian cancers (long-term outcomes of menopause) which are more commonly prevalent in developed countries.
Age at natural menopause
Natural menopause refers to cessation of the menstrual cycle without any surgical procedures such as oophorectomy or ovarian failure as a result of chemotherapy or radiotherapy(Reference Davis, Lambrinoudaki and Lumsden3). A premature menopause is one which is reached before the age of 40 years, an early menopause between 40 and 45 years and a late menopause is one after the age of 55 years(Reference Okeke, Anyaehie and Ezenyeaku17, Reference Faubion, Kuhle and Shuster18). Depletion of the ovarian reserve and its responsiveness to pituitary gonadotropins governs the lifespan of the ovary and thus influences the onset of the timing of the natural menopause(Reference Sherman19). Dietary factors and diet-related disorders can either enhance the lifetime of the ovaries by delaying follicular atresia or by maintaining sex-hormone levels involved in the feedback mechanisms of the menstrual cycle. However, the exact mechanisms still need to be elucidated. The association of age at natural menopause with chronic disease, ageing and general health makes it an important subject of clinical and public interest(Reference Gold12).
Metabolic disorders such as diabetes could accelerate reproductive ageing by causing premature ovarian failure through several mechanisms. This has been demonstrated in a study(Reference Monterrosa-Castro, Blümel and Portela-Buelvas20) including women from eleven Latin American countries. The author reported that diabetic women had an earlier menopause as opposed to non-diabetic women. Similarly, a recent study conducted in the Southern part of India demonstrated that an early menopause was more likely to be reported by diabetic women(Reference Sekhar, Medarametla and Rahman21). This is further supported by a British study which investigated the association between various food groups and the timing of the onset of natural menopause among 914 women in the Women's Cohort Study. It was found that a high consumption of refined pasta and rice, which are high glycaemic index foods, were associated with an earlier onset of natural menopause(Reference Dunneram, Greenwood and Burley22). Furthermore, findings from the prospective Nurses Health Study II demonstrated that a high vitamin D intake was associated with a lower risk of an early onset of menopause(Reference Purdue-Smithe, Whitcomb and Szegda23) which could be due to the fact that a high serum 25-dihydroxyvitamin D concentration could reduce the risk of diabetes as well as metabolic syndrome(Reference Parker, Hashmi and Dutton24). These findings thus indicate that the presence of type II diabetes, a diet-related disease could lead to an earlier onset of menopause.
Vegetarianism has also been linked to an earlier age at natural menopause(Reference Dunneram, Greenwood and Burley22, Reference Biela25). Vegetarian diets are usually characterised by a high dietary fibre and low-fat content, particularly saturated fats. They tend to include more whole grains, vegetable protein sources such as legumes, nuts and soya protein, and exclude red meat. Dietary fibre may potentially interfere in the enterohepatic circulation of sex hormones, by modifying the metabolic pathway of oestrogens, leading to a decrease in oestrogen bioavailability(Reference Gold12, Reference Bagga, Ashley and Geffrey26). Karelis et al.(Reference Karelis, Fex and Filion27) demonstrated that vegetarians had higher levels of sex-hormone binding globulin, higher total fibre intake as well as lower levels of free oestradiol, free testosterone, dehydroepiandrosterone sulphate and a lower BMI. An intervention study also reported that a change in fibre intake was significantly and independently associated with a decrease in serum bioavailable oestradiol and total oestradiol concentrations while no association was found between a change in fat intake and the hormone concentrations(Reference Rock, Flatt and Thomson28).
Conversely, intakes of green and yellow vegetables as well as fresh legumes have been associated with a delayed onset of menopause(Reference Dunneram, Greenwood and Burley22, Reference Nagata, Takatsuka and Kawakami29). Ovarian ageing is closely associated with increased levels of reactive oxygen species which arises mainly due to an imbalance between reactive oxygen species production and non-enzymatic antioxidant defences(Reference Agarwal, Gupta and Sharma30). Oocyte maturation, ovulation, luteolysis and follicle atresia are all affected by reactive oxygen species(Reference Ruder, Hartman and Blumberg31). Antioxidant properties of foods have been found to be positively associated with a reduced rate of follicular atresia. A recent in vivo study demonstrated a reduced atretic follicle count with use of resveratrol (a polyphenol found in the skin of red grapes and berries)(Reference Ozcan, Ficicioglu and Yildirim32). These contradictory findings could be because while few studies looked at the associations with dietary patterns, others considered the associations with individual food items. Moreover, differences in the participants’ characteristics and distribution of age at natural menopause could further influence the findings. The confounders used in the analyses and large sample sizes could also explain the differences.
High consumptions of meat, fat and protein have been positively associated with a delayed onset of menopause (Table 1). Cholesterol, the starting product of steroidogenesis can be synthesised by de novo synthesis in the endocrine tissue (e.g. granulosa-lutein cells in the ovaries) from acetate, the end-product of fat oxidation(Reference Berg, Tymoczko, Stryer and Zimmerman33). Therefore, an excessive dietary fat intake can result in higher serum oestradiol levels. In addition, during the menopausal transition, significant changes occur in body composition. For instance, redistribution of body fat takes place such that there is an increase of total and central body fat, and also a redistribution of fat from lower body subcutaneous fat towards the abdominal region. This increase in adipose tissue becomes the main site for oestrogen production along with other hormones such as leptin, adiponectin and resistin(Reference Zsakai, Karkus and Utczas34, Reference Ho, Wu and Chan35). Therefore, these endocrine changes taking place during the menopausal transition together with a high-fat diet predisposes the woman to a later onset of menopause.
Table 1. Evidence for the associations between diet and onset of menopause
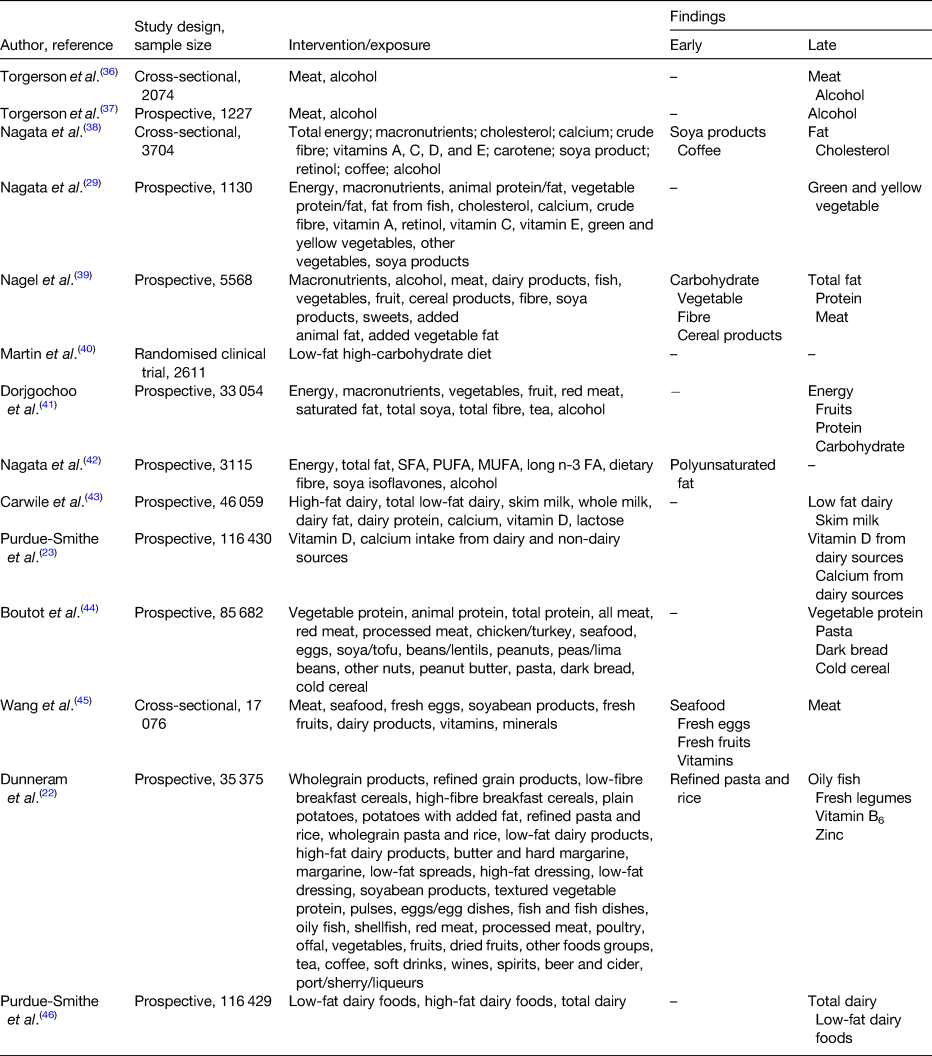
Menopause and its associated sequelae
The timing of menopause could determine the duration of the presence of VMS which is mostly prevalent during the perimenopausal years as a consequence of lowered oestrogen levels (Fig. 1). Previous randomised controlled trials have mainly focused on the study of phytoestrogen extracts and their influence on the presence of VMS. However, the study of foods consumed as part of the normal diet in relation to the presence of VMS has received less attention. The decline in oestrogen levels during the menopausal transition is postulated to be one of the causes for the presence of VMS. A low oestrogen level has been associated with narrowing of the thermoneutral zone between the core body temperatures, resulting in a lowered sweating threshold and hence a higher likelihood to experience hot flushes and night sweats. However, given that about 20 % of premenopausal women also report hot flushes suggests that the decline in oestrogen levels is not the sole endocrine change causing VMS(Reference O'Neill and Eden47). Dhanoya et al.(Reference Dhanoya, Sievert and Muttukrishna48) demonstrated that both AMH and FSH were associated with the presence of hot flushes while the level of oestradiol was not related with hot flushes.
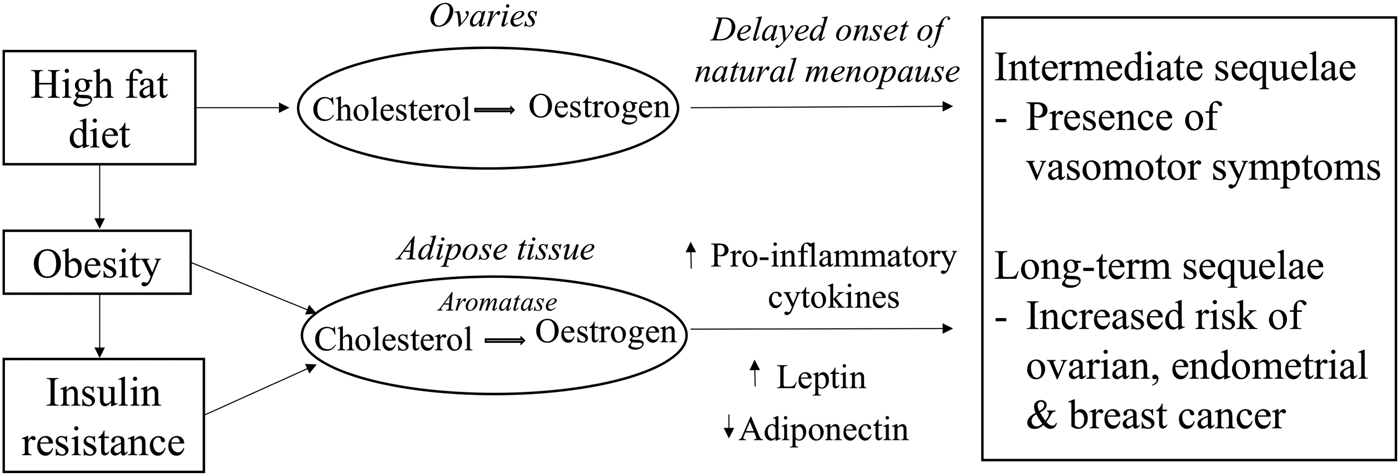
Fig. 1. Potential mechanistic pathways through which diet can influence circulating oestrogen levels and women's reproductive health.
Prolonged exposure to oestrogens as a consequence of a delayed menopause increases the risk of hormone-dependent cancers such as ovarian, endometrial and breast cancer as demonstrated previously by several epidemiological studies(49–Reference Xu, Xiang and Ruan51). Other hormones such as progesterone may also be important. These hypotheses have been investigated in earlier published reviews(Reference Travis and Key52–Reference Mungenast and Thalhammer54). Other factors such as diet (Fig. 1), a modifiable risk factor may also explain the variation in oestrogen and other sex hormones levels(Reference Wu, Pike and Stram55–Reference Gaskins, Mumford and Zhang57). Diet-related pathologies may also promote tumourigenesis while some components of the diet may be protective against these cancers. Therefore, the next sections explore the evidence for the hypothesis that diet is a major determinant for the presence of VMS and for the risk of hormone-related cancers.
Presence of vasomotor symptoms
VMS such as hot flushes and night sweats are one of the most common symptoms experienced by women during the menopausal transition. The median duration of these symptoms is 4 years but may persist as long as 15 years for some women(Reference O'Neill and Eden47).
Evidence for a link between diet and presence of VMS arises from studies which have previously explored the associations between phytoestrogen extracts or phyto-oestrogen-rich foods and frequency or severity of VMS. A Cochrane review of forty-three randomised controlled trials did not support the beneficial effects of phyto-oestrogen supplements for the reduction of the frequency or severity of VMS mainly due to the small size of the trials and also the high risk of bias while the same review stated the promising effect of genistein, a phytoestrogen found in soya(Reference Lethaby, Marjoribanks and Kronenberg58). A recent review further indicated the beneficial effect of isoflavones against hot flushes(Reference Schmidt, Arjomand-Wolkart and Birkhauser59).
As mentioned previously, women tend to accumulate subcutaneous fat in the abdominal region during the menopausal transition which leads to endocrine changes in terms of higher circulating oestradiol level(Reference Zsakai, Karkus and Utczas34). A prospective study of 6040 women demonstrated that a Mediterranean-style diet and a fruit-rich diet were both inversely associated with VMS. Conversely, diets with high-fat and sugar contents increased the risk of VMS(Reference Herber-Gast and Mishra60). This could imply that a healthier diet which prevents obesity could also be protective against VMS. The same study reported that even after adjusting for BMI, the same associations were observed. Therefore, the mechanism involved between diet and presence of VMS still remains unclear.
Ovarian cancer
Women of reproductive age undergo cyclical cellular changes in their genital tract during the menstrual cycle(Reference Begum and A61). During each cycle, several follicles containing an ovum undergo a maturation and selection process where ordinarily one of them is selected and released from the ovary during ovulation on or around the 14th day of the cycle(Reference Nair, Taylor, Santoro and Neal-Perry62). The menstrual cycle is under the influence of various hormones namely gonadotrophin-releasing hormone, luteinising hormone, FSH, oestrogen and progesterone(Reference Beshay, Carr, Falcone and Hurd63). During ovulation the surface of the ovary ruptures to release the ovum, following which the cells on the surface of the ovary, known as the epithelial cells, proliferate to close the breach under the influence of oestrogen. Improper proliferation of those cells can result in formation of cysts or even cancers such as surface epithelial tumours which are a sub group among the diverse types of ovarian tumours(Reference Lengyel64).
Oestrogen and progesterone are steroid hormones synthesised from cholesterol(Reference Berg, Tymoczko, Stryer and Zimmerman33); individuals having a high-fat diet provide the substrate for excessive oestrogen synthesis which stimulates cell proliferation in the female genital tract. Diets high in animal protein also contains xeno-oestrogens which have carcinogenic potential(Reference Fucic, Gamulin and Ferencic65). Leptin, another hormone secreted by the adipose tissue under the influence of factors such as high lipid levels in blood, has several effects on the body such as producing a feeling of satiety, as well as stimulating the release of gonadotropin-releasing hormone which in turn stimulates release of luteinising hormone and FSH(Reference Ogura, Irahara and Kiyokawa66). High levels of luteinising hormone may result in the immature release of the ovum and high levels of oestrogen secondary to high circulating cholesterol levels in the body (as a result of high saturated fat and energy intake). Consequently, this may result in improper re-epithelialisation of the ovaries. Chronic stimulation of ovaries in this way may predispose to development of abnormal growths which subsequently can undergo malignant transformation. Therefore, diets high in energy, fats or animal protein may promote development of ovarian cancer.
n-3 Fatty acid (FA), is a PUFA which can be obtained through dietary sources (flaxseeds, walnuts, rapeseed oil and oily fish) only. The n-3 family of PUFA comprises α-linolenic acid, EPA and DHA. According to in vivo studies, EPA and DHA have been found to be precursors for anti-inflammatory lipid mediators(Reference Azrad, Turgeon and Demark-Wahnefried67). Oestrogen has proliferative effects on oestrogen-sensitive tissues and thus could be involved in the pathogenesis of some hormone-dependent cancers such as ovarian cancer. Dietary n-3 PUFA deter the promotion and progression stages of carcinogenesis through several mechanistic pathways. One of the mechanisms involves changes in oestrogen metabolism which could result in reduced oestrogen-stimulated cell growth(Reference Larsson, Kumlin and Ingelman-Sundberg68, Reference Calder69).
PGE2, an arachidonic acid (an n-6 PUFA found in meat and fish)-derived eicosanoid stimulates the activity of aromatase P450, which converts C19 steroids to oestrogens, while conversely PGE3 (derived from EPA metabolism) does not activate aromatase P450. Hence, an increased intake of EPA, which leads to increased production of PGE3 and decreased production of PGE2, is expected to decrease oestrogen production and thus reduce oestrogen-stimulated cell growth(Reference Larsson, Kumlin and Ingelman-Sundberg68). In addition, n-3 PUFA can influence the regulation of two transcription factors; sterol regulatory element binding protein-1c and PPARα. Sterol regulatory element binding protein-1c is involved in inducing a set of lipogenic enzymes in liver and n-3 PUFA can potentially inhibit the expression and processing of sterol regulatory element binding protein-1c and thus inhibits the de novo lipogenesis of FA, making it an important consideration for the carcinogenesis. Merritt et al.(Reference Merritt, Cramer and Missmer70) demonstrated that a higher intake of n-3 PUFA may be protective for ovarian cancer, while a greater consumption of trans-fat was associated with an increased risk of ovarian cancer. However, the clinical effects of n-3 PUFA do not solely rely on its concentration alone, but most importantly on the ratio of n-3 PUFA to n-6 PUFA in the cells(Reference Hu, Zhang and Song71). For instance, in a study using a knockout mouse model, it was demonstrated that a dietary of n-6:n-3 PUFA ratio lower than 5 was effective in suppressing tumour growth, and prolonging animal lifespan(Reference Berquin, Min and Wu72). Thus, a high intake of n-3 PUFA relative to that of n-6 PUFA may decrease endogenous oestrogen production and reduce the risk of ovarian cancer.
Along with hormonal control, diet can also interfere at the level of FA and cholesterol biosynthesis and eventually affect sex steroid metabolism and thus risk of ovarian cancer(Reference Wong and Sul73). For instance, it has been found that feeding previously fasted animals a diet high in carbohydrate and low in fat content causes a dramatic induction of enzymes such as fatty acid synthase and mitochondrial glycerol-3-phosphate acyltransferase which are involved in FA and TAG synthesis. Fatty acid synthase and glycerol-3-phosphate acyltransferase are the two critical enzymes involved in FA and TAG biosynthesis. Fatty acid synthase catalyses the synthesis of long-chain FA, primarily palmitate, using acetyl-CoA and malonyl-CoA as substrates and NADPH as the reducing equivalent while glycerol-3-phosphate acyltransferase catalyses the first committed as well as the rate-limiting step in TAG and phospholipid biosynthesis(Reference Sul and Wang74).
Dietary variations are responsible for fluctuations in nutrient intake which can result in changes in circulating glucose, which in turn signal the secretion of hormones. For example, ingestion of a high-carbohydrate diet leads to a high circulating insulin level which consequently induces enzymes involved in FA and TAG synthesis, thus providing FA for membrane phospholipid biosynthesis in cancer cells. Conversely, during a state of fasting or starvation, glucagon level is elevated which suppresses activities of enzymes involved in FA and TAG biosynthesis by increasing the intracellular cAMP level(Reference Wong and Sul73–Reference Currie, Schulze and Zechner75). Moreover, in vivo studies have demonstrated that high-carbohydrate and low-fat diets lead to higher rates of lipogenesis than diets rich in fat and low in carbohydrates. The type of carbohydrate also affects lipogenesis such that diets with fructose as the primary source of carbohydrate cause higher rates of FA synthesis and higher activities of the lipogenic enzymes than diets containing equivalent amounts of glucose(Reference Hillgartner, Salati and Goodridge76).
Endometrial cancer
As mentioned previously, the cells in the endometrium undergo cyclical cellular changes during the menstrual cycle. Hormones such as oestrogen have a mitogenic effect on the cells of the endometrium(Reference Kaaks, Lukanova and Kurzer77, Reference Losordo and Isner78). Excessive exposure to oestrogen either exogenous or endogenous secondary to high-fat diet may cause increased proliferation of the endometrial cells. Cells proliferating at a faster rate are more prone to errors during DNA replication and the mutated cells can subsequently undergo malignant transformation, most commonly adenocarcinomas.
Endometrial cancer is a hormone-driven cancer, with approximately 80 % of endometrial cancers potentially arising due to either an excess of oestrogen or a lack of progesterone. In the normal endometrium, the proliferative effects of oestrogen are normally countered by progesterone, but the absence of progesterone allows oestrogen to induce oncogenesis, an effect that is amplified in situations of excess oestrogen (Fig. 1). One of the major emerging causes of the oestrogen/progesterone imbalance is obesity which is known to influence hormonal balance and level of growth factors(Reference Xu, Matthews and Xiang79, Reference Carlson, Thiel and Yang80). Evidence shows a positive link between increased dietary fat intake and obesity, thus associating fat intake to an increased risk of endometrial cancer(Reference Goodman, Wilkens and Hankin81). Central obesity, characterised by high abdominal fatness, is commonly observed among women during the menopausal years and is responsible for the increase in circulating NEFA and consequently promotes an increase in insulin resistance(82). In addition, long-term consumption of high glycaemic index diet is another risk factor for obesity and insulin resistance and is also hypothesised to be involved in the pathogenesis of endometrial cancer(Reference Mulholland, Murray and Cardwell83). Hyperinsulinaemia increases the risk of endometrial cancers mainly by the binding of insulin to insulin receptors on endometrial cells to stimulate the growth of endometrial stromal cells as well as through other pathways(Reference Kaaks, Lukanova and Kurzer77).
Breast cancer
The pathogenesis of breast cancer is intricate and multifactorial. The aetiology of breast cancer could include mutation in the BRCA1 gene, a family history of breast cancer or mutagens which can lead to DNA damage. It can also involve a similar hormonal pathogenesis as ovarian cancer(Reference Yoneda, Lendorf and Couchman84). Importantly, oestrogen influences growth, differentiation and functioning of the breast tissue (Fig. 1). Aromatase, an enzyme found in the adipose tissues helps convert circulating cholesterol to oestradiol(Reference Cleary and Grossmann85). Due to the higher proportion of fat cells in breasts of older women, their level of oestradiol in the breast tissues particularly postmenopause is likely to be higher than the plasma circulating level. The high oestradiol level in the breast tissues can trigger differential effects on the oestrogen receptor (ER) expression which are found in those tissues, thus influencing the behaviour of cancer cells(Reference Malara, Leotta and Sidoti86). Stromal cells in the breast tissues can also support metastatic activity as they do not only control growth of normal breast epithelial cells but also that of neoplastic epithelial cells by secreting growth factors in response to the levels of endogenous hormones(Reference Bussard, Mutkus and Stumpf87).
High cholesterol level, as a result of a high-fat diet, has also been stated as a risk factor for breast cancer among women during the late peri-menopausal and post-menopausal state(Reference Llaverias, Danilo and Mercier88). According to studies in mice(Reference Wu, Ishikawa and Sirianni89, Reference Baek, Yu and He90), oxysterol 27-hydroxycholestrol, a metabolite of cholesterol synthesis has been identified in the pathogenesis of breast cancer. The 27-hydroxycholestrol could stimulate the growth of breast cancer cell lines by binding and activating ER in a similar way as oestradiol. There is also evidence that postmenopausal women experience an increase in their cholesterol level and thus its metabolite 27-hydroxycholestrol which could help explain the increase in breast cancer risk among obese and hypercholesteraemic women(Reference Derby, Crawford and Pasternak91). However, according to a recent EPIC-Heidelberg Cohort Study publication including 530 incident cases of breast cancer, a high level of 27-hydroxycholestrol was associated with a reduced risk of breast cancer among postmenopausal women and no association was found among premenopausal women(Reference Lu, Le Cornet and Sookthai92).
Moreover, a fat-rich diet is positively correlated with insulin resistance(Reference De Souza, Araujo and Bordin93). Insulin resistance, a major factor in the pathogenesis of premenopausal breast cancer, is also involved in the aetiology of postmenopausal breast cancer. Insulin can bind to insulin receptors found on the epithelial cells of the breast. This insulin signalling can contribute to cancer through mitogenic activity mediated by the phosphatidylinositol-3 kinase and mitogen-activated protein kinase/Akt signalling pathways(Reference Orgel and Mittelman94). Insulin also has anti-apoptotic characteristics and thus promotes tumour-invasive activity. Insulin resistance is also accompanied by high levels of proinflammatory cytokines and leptin as well as a decreased level of adiponectin which concomitantly lead to both ER-positive and ER-negative breast cancer(Reference Rose, Gracheck and Vona-Davis95). Moreover, insulin resistance is associated with an increased oestrogen level as a result of enhanced aromatase activity and decreased production of sex-hormone binding globulin(Reference Mukhopadhyay, Liu and Bandyopadhyay96, Reference Daka, Rosen and Jansson97). This mechanistic pathway has been supported by an Italian-nested case–control study which demonstrated that both pre- and postmenopausal women with hyperglycaemia had an increased risk of breast cancer(Reference Sieri, Muti and Claudia98).
In addition to the high circulating level of oestrogen as a result of obesity, the associated high levels of inflammatory markers, insulin-like growth factors and adipokines from the visceral fat also increases the risk of breast cancer among postmenopausal women(Reference Howe, Subbaramaiah and Hudis99). While high circulating oestrogen level among premenopausal women can be a risk factor for breast cancer(Reference Key, Appleby and Reeves100), some studies have demonstrated that obesity can be protective among premenopausal women. Obesity can lead to irregular ovarian cycles and hence lower circulating oestrogen levels. As demonstrated by a meta-analysis of prospective studies, waist circumference was associated with ER-positive and progesterone receptor-positive breast cancers in postmenopausal women while in premenopausal women waist circumference was positively associated with ER-negative breast cancer(Reference Chen, Chen and Zhang101). This would suggest a lower likelihood of a hormonal pathogenesis for breast cancer among premenopausal women. Chronic inflammation, abnormally high levels of insulin-like growth factor and insulin resistance have been linked to premenopausal breast cancer(Reference Luque, López-Sánchez and Villa-Osaba102).
Other protective effect of diet against the risk of hormone-dependent cancers
Vitamins such as B6, B12 and folate are required for normal DNA repair mechanisms and proper DNA replication. Folate receptor α expression is correlated with stage and grade of ovarian cancer, suggesting this pathway may be relevant to ovarian carcinogenesis and progression(Reference Harris, Cramer and Vitonis103). Ascorbic acid, vitamin E and other trace elements such as selenium having antioxidant properties help to protect from free radical injury and maintain normal cellular function. Vitamin C is recognised for its beneficial effect in cancer chemoprevention mainly as it has the potential to stimulate immune function, impede nitrosamine formation, minimise DNA damage and block the metabolic activation of carcinogens(Reference Bandera, Gifkins and Moore104). Vitamin A helps to control epithelisation of tissues and also has antioxidant properties to help protect from DNA damage(Reference Donaldson105). Although these current theories support the plausible role of these micronutrients in hormone-dependent cancer, prospective studies as well as a recent pooled analysis of cohort studies and meta-analyses reported no association between dietary vitamins A, C or E and the risk of ovarian and endometrial cancers(Reference Bandera, Gifkins and Moore104, Reference Navarro Silvera, Jain and Howe106–Reference Koushik, Wang and Anderson108). In addition, the World Cancer Research Fund/American Institute for Cancer Research(82) reported inconclusive association between nutrients such as vitamin A, C, E as well as folate and the incidence of ovarian, endometrial, breast cancers.
Moreover, a recent meta-analysis of cohort and case–control studies suggested that vitamin D intake was protective against premenopausal breast cancer(Reference Estébanez, Gómez-Acebo and Palazuelos109). A large cohort study including 68 567 postmenopausal women further demonstrated that women with a high intake of calcium, and vitamin D had a reduced risk of postmenopausal breast cancer(Reference McCullough, Rodriguez and Diver110). Experimental studies have also suggested that vitamin D intake could reduce the stimulatory effect of androgen in human ovarian cancer cell lines and also reduce obesity-induced endometrial cancer(Reference Ahonen, Zhuang and Aine111, Reference Yu, Cline and Maxwell112). However, systematic reviews concluded that the evidence to support the association between vitamin D intake and endometrial and ovarian cancers are not consistent and strong, thus calling for further prospective studies. One of the limitations was that since most of the studies included in this systematic review were case–control studies, diet was thus measured only at one time period and was very prone to misreporting due to recall bias, therefore not accounting for diet change over time and vitamin D production through the skin(Reference Cook113, Reference McCullough, Bandera and Moore114).
Flavonoids, a group of heterogeneous polyphenols, have multiple health benefits. The main sources of flavonoids include fruits, vegetables, tea and wine(Reference Romagnolo and Selmin115). Flavonoids reportedly have several properties which contribute to the various health benefits including antioxidant, anti-mutagenic and anti-proliferative properties. Among them, isoflavones and some flavones, flavanones, and flavanols also have oestrogenic or anti-oestrogenic activity, which makes these compounds of particular interest for modulation of reproductive cancer risks(Reference Rossi and La Vecchia116). According to a large prospective cohort study including 171 940 US women, 723 of whom developed ovarian cancer over a period of 16–22 years of follow-up, demonstrated inverse associations between flavonol and flavanone intakes and ovarian cancer risk(Reference Cassidy, Huang and Rice117). Further supporting the chemoprotective role of the flavonol in ovarian cancer risk, two in vitro studies demonstrated that kaempferol induces apoptosis in ovarian cancer cells by regulating pro-apoptotic and anti-apoptotic protein expressions and by preventing angiogenesis in ovarian cancer cells(Reference Luo, Rankin and Li118, Reference Luo, Rankin and Juliano119). Furthermore, a meta-analysis of six cohort and six case–control studies demonstrated that intakes of flavonols and flavones are protective against breast cancer, especially among postmenopausal women(Reference Hui, Qi and Qianyong120), thus supporting the chemo-preventive role of fruits and vegetables in hormone-related cancers.
Conclusion
In summary, evidence shows that diets predisposing to obesity and insulin resistance are the main drivers of sex hormone fluctuations among both pre- and post-menopausal women. Fluctuations in oestrogen levels have been associated with the timing of the onset of natural menopause, the presence of VMS and longer term sequelae such as ovarian, endometrial and breast cancer. Studies have demonstrated that both the consumption of more balanced diets, rich in fibre, fruits and vegetables (and, by contrast, those less healthy containing processed meats and rich in fat) can alter circulating levels of oestrogen and other sex hormones. Diet could consequently influence the timing of natural menopause and hence affect its associated sequelae. However, further evidence around the hypothesis that diet might influence timing of menopause and presence of VMS are required in observational trials and use of metabolomics may be valuable in revealing mechanistic pathways. Additional observational studies may also clarify the association between diet and hormone-related cancers.
Acknowledgements
The authors would like to thank Dr Nigel A. Simpson for his valuable feedback.
Financial Support
Y. D. is in receipt of a scholarship from the Commonwealth Scholarships Commission, UK. The funder had no role in the writing of this manuscript.
Conflict of Interest
J. E. C. is a director of the University of Leeds spin out company Dietary Assessment Ltd.
Authorship
Y. D. drafted the manuscript. J. E. C. and D. C. G. critically revised the manuscript for important intellectual content. All the authors have read and approved the final manuscript.